Abstract
We evaluated the effects of acetazolamide on Na+-HCO3- cotransport in basolateral membrane vesicles isolated from the rabbit renal cortex. Na+ uptake stimulated by an imposed inward HCO3- gradient was not significantly reduced by 1.2 mM acetazolamide, indicating that acetazolamide does not directly inhibit Na+-HCO3- cotransport. 4,4'-Diisothiocyanostilbene-2,2'-disulfonate (DIDS)-sensitive Na+-base cotransport was found to be absolutely CO2/HCO3--dependent. We therefore tested whether acetazolamide-sensitive availability of HCO3- at the basolateral membrane could be rate-limiting for Na+-base cotransport under some conditions. In the presence of a CO2/HCO3- buffer system but absence of an initial HCO3- gradient, Na+ influx was stimulated fivefold by an outward NH4+ gradient. This stimulation of Na+ influx by an outward NH4+ gradient was inhibited greater than 75% by 0.6 mM acetazolamide, suggesting that acetazolamide blocked the ability of the NH4+ gradient to generate an inward HCO3- gradient. In the presence of an inward HCO3- gradient, Na+ influx was inhibited greater than 70% by an inward NH4+ gradient. This inhibition of Na+ influx was reduced to only 35% by 0.6 mM acetazolamide, suggesting that acetazolamide blocked the ability of NH4+ to collapse the inward HCO3- gradient. Similarly, Na+ influx in the presence of an inward HCO3- gradient was inhibited greater than 80% by an outward acetate gradient, and this inhibition was reduced to only 50% by acetazolamide. Thus, acetazolamide caused either inhibition or stimulation of Na+ uptake depending on the conditions with respect to pH and HCO3- gradients. The indirect interaction of acetazolamide with the basolateral membrane Na+-HCO3- cotransport system may be an important mechanism underlying inhibition of proximal tubule acid secretion by this agent.
Full text
PDF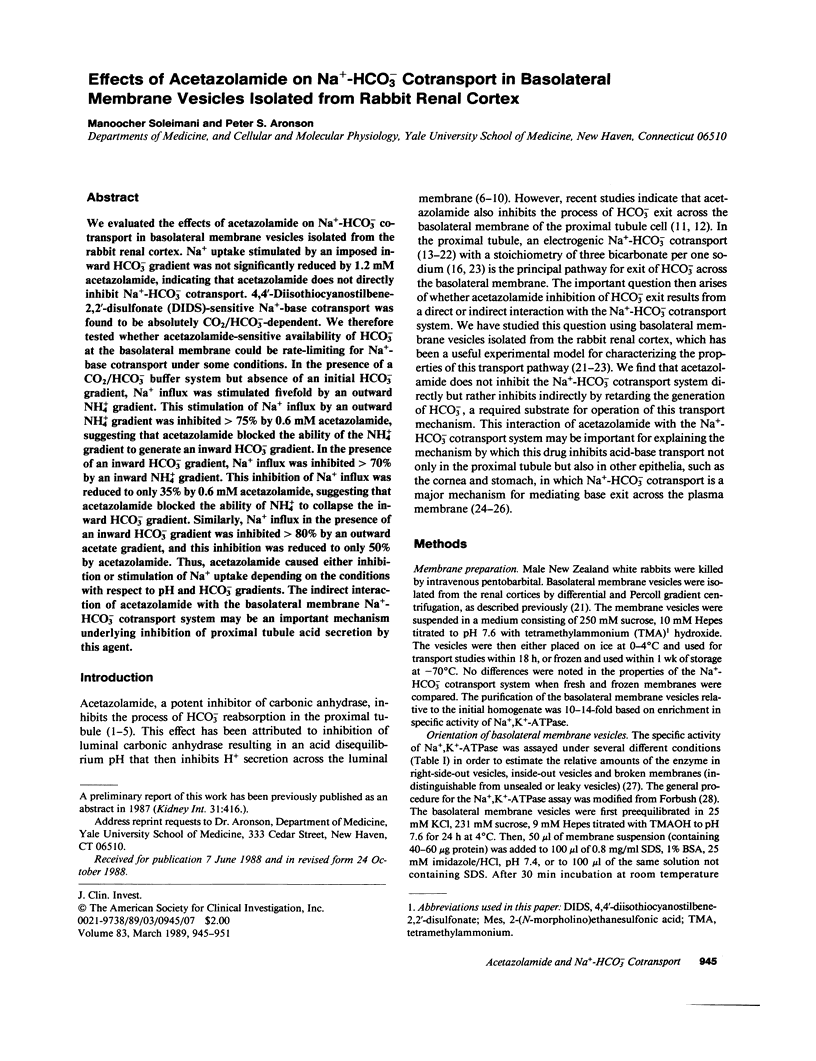
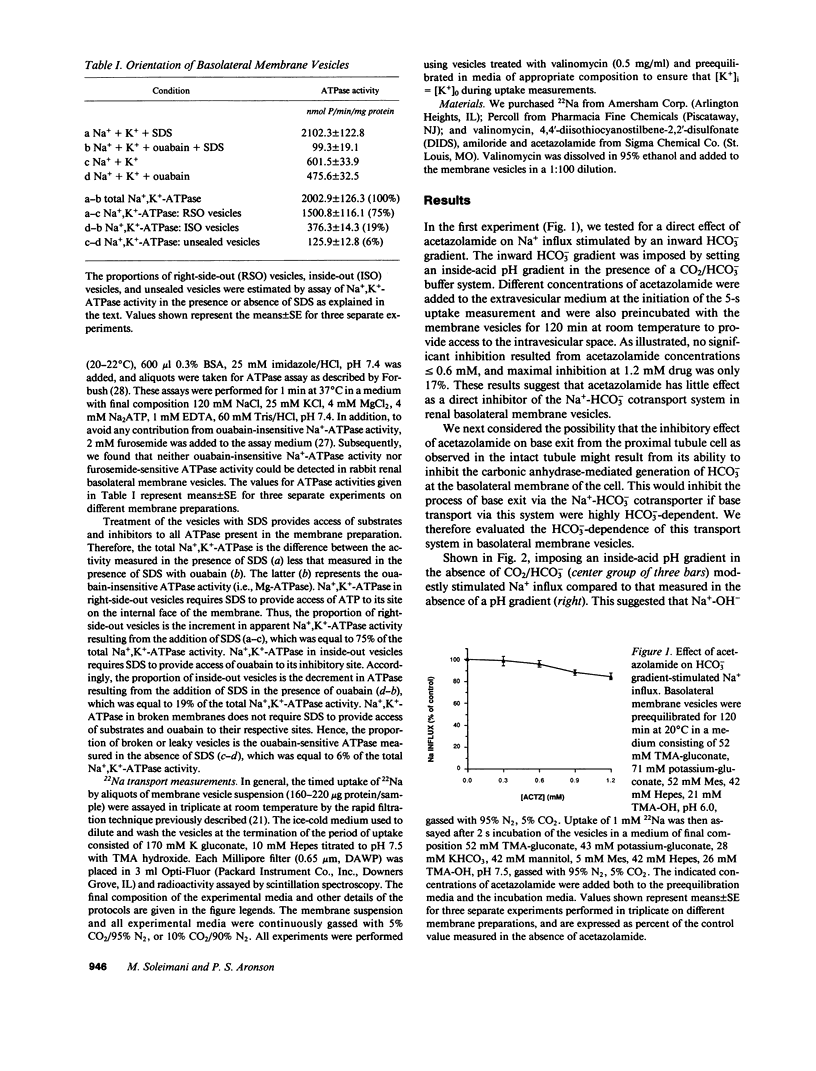
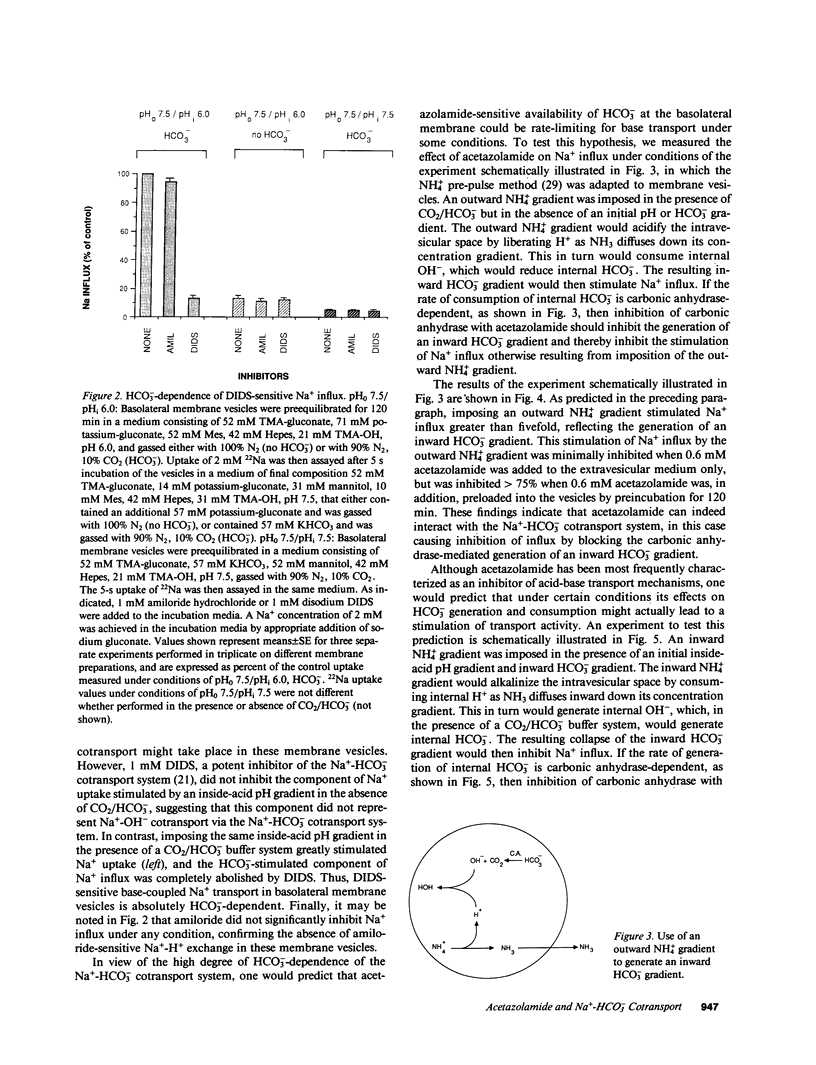
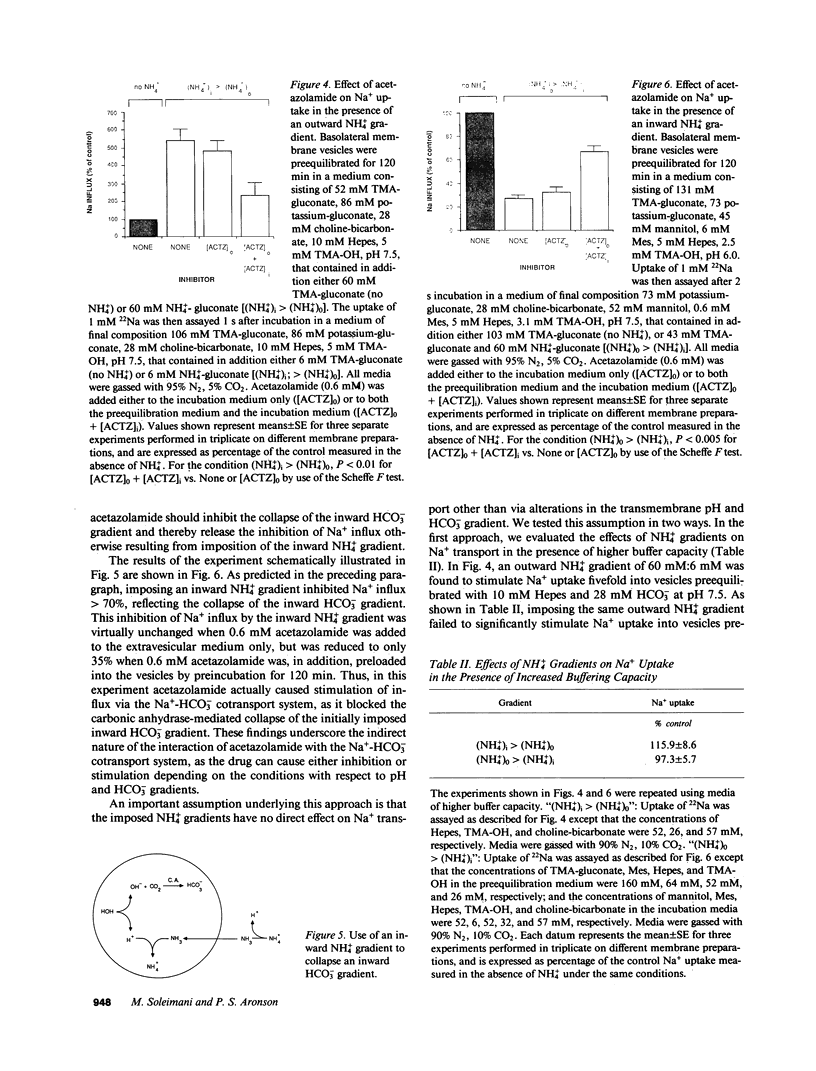
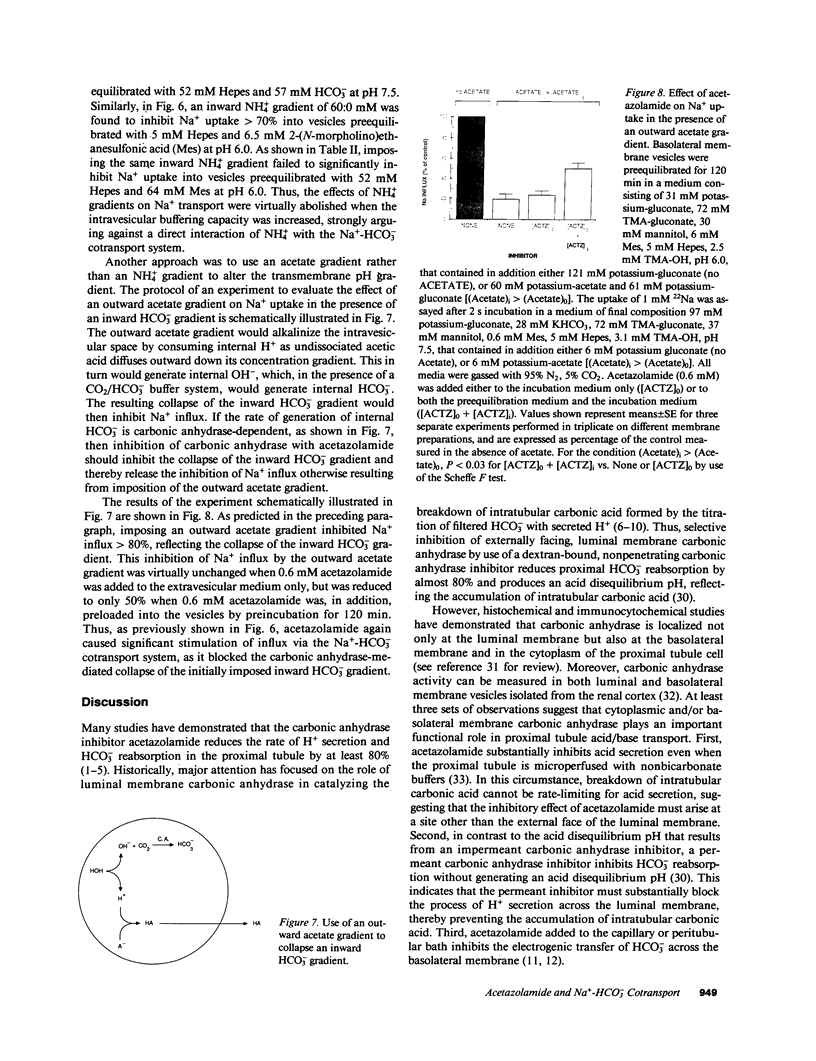
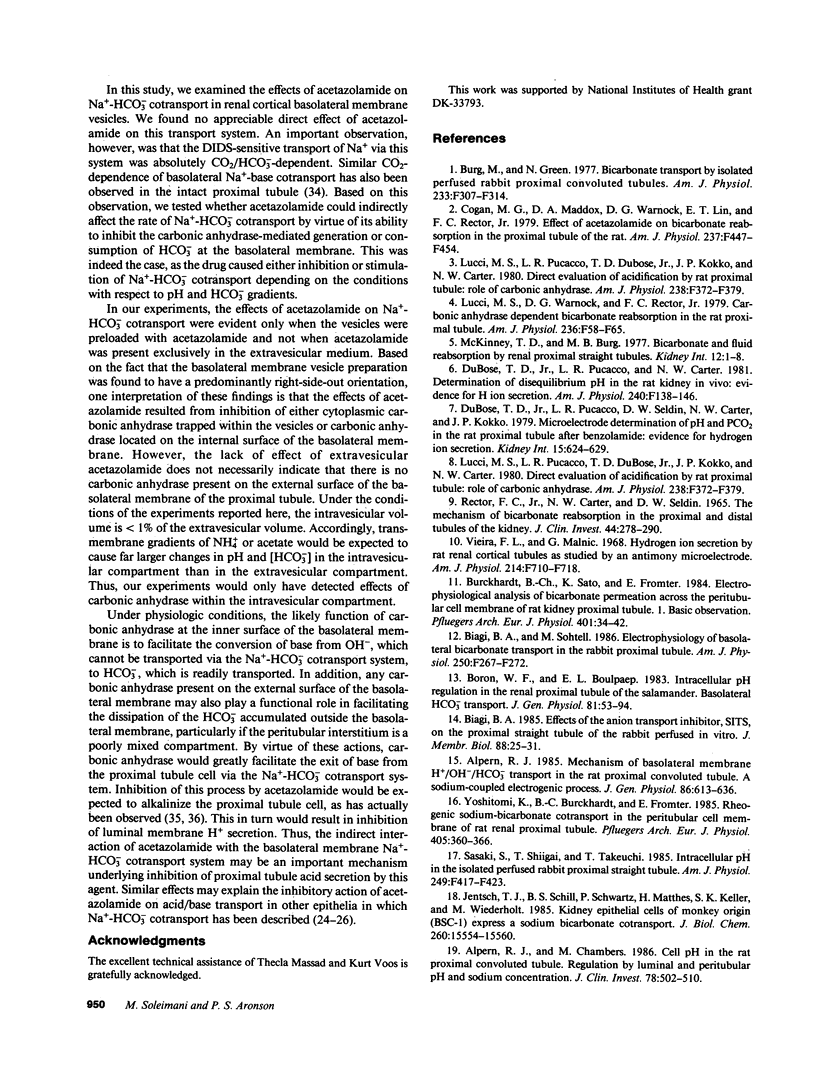
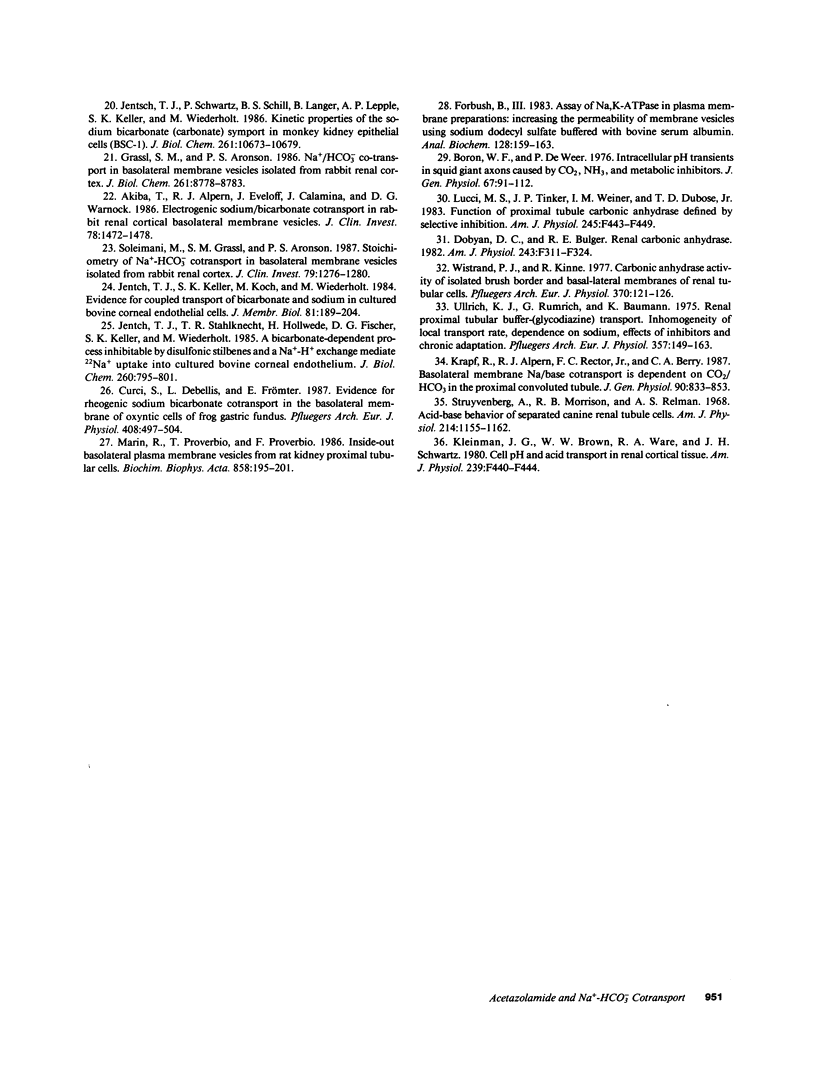
Images in this article
Selected References
These references are in PubMed. This may not be the complete list of references from this article.
- Akiba T., Alpern R. J., Eveloff J., Calamina J., Warnock D. G. Electrogenic sodium/bicarbonate cotransport in rabbit renal cortical basolateral membrane vesicles. J Clin Invest. 1986 Dec;78(6):1472–1478. doi: 10.1172/JCI112738. [DOI] [PMC free article] [PubMed] [Google Scholar]
- Alpern R. J., Chambers M. Cell pH in the rat proximal convoluted tubule. Regulation by luminal and peritubular pH and sodium concentration. J Clin Invest. 1986 Aug;78(2):502–510. doi: 10.1172/JCI112602. [DOI] [PMC free article] [PubMed] [Google Scholar]
- Alpern R. J. Mechanism of basolateral membrane H+/OH-/HCO-3 transport in the rat proximal convoluted tubule. A sodium-coupled electrogenic process. J Gen Physiol. 1985 Nov;86(5):613–636. doi: 10.1085/jgp.86.5.613. [DOI] [PMC free article] [PubMed] [Google Scholar]
- Biagi B. A. Effects of the anion transport inhibitor, SITS, on the proximal straight tubule of the rabbit perfused in vitro. J Membr Biol. 1985;88(1):25–31. doi: 10.1007/BF01871210. [DOI] [PubMed] [Google Scholar]
- Biagi B. A., Sohtell M. Electrophysiology of basolateral bicarbonate transport in the rabbit proximal tubule. Am J Physiol. 1986 Feb;250(2 Pt 2):F267–F272. doi: 10.1152/ajprenal.1986.250.2.F267. [DOI] [PubMed] [Google Scholar]
- Boron W. F., Boulpaep E. L. Intracellular pH regulation in the renal proximal tubule of the salamander. Basolateral HCO3- transport. J Gen Physiol. 1983 Jan;81(1):53–94. doi: 10.1085/jgp.81.1.53. [DOI] [PMC free article] [PubMed] [Google Scholar]
- Boron W. F., De Weer P. Intracellular pH transients in squid giant axons caused by CO2, NH3, and metabolic inhibitors. J Gen Physiol. 1976 Jan;67(1):91–112. doi: 10.1085/jgp.67.1.91. [DOI] [PMC free article] [PubMed] [Google Scholar]
- Burckhardt B. C., Sato K., Frömter E. Electrophysiological analysis of bicarbonate permeation across the peritubular cell membrane of rat kidney proximal tubule. I. Basic observations. Pflugers Arch. 1984 May;401(1):34–42. doi: 10.1007/BF00581530. [DOI] [PubMed] [Google Scholar]
- Burg M., Green N. Bicarbonate transport by isolated perfused rabbit proximal convoluted tubules. Am J Physiol. 1977 Oct;233(4):F307–F314. doi: 10.1152/ajprenal.1977.233.4.F307. [DOI] [PubMed] [Google Scholar]
- Cogan M. G., Maddox D. A., Warnock D. G., Lin E. T., Rector F. C., Jr Effect of acetazolamide on bicarbonate reabsorption in the proximal tubule of the rat. Am J Physiol. 1979 Dec;237(6):F447–F454. doi: 10.1152/ajprenal.1979.237.6.F447. [DOI] [PubMed] [Google Scholar]
- Curci S., Debellis L., Frömter E. Evidence for rheogenic sodium bicarbonate cotransport in the basolateral membrane of oxyntic cells of frog gastric fundus. Pflugers Arch. 1987 May;408(5):497–504. doi: 10.1007/BF00585075. [DOI] [PubMed] [Google Scholar]
- Dobyan D. C., Bulger R. E. Renal carbonic anhydrase. Am J Physiol. 1982 Oct;243(4):F311–F324. doi: 10.1152/ajprenal.1982.243.4.F311. [DOI] [PubMed] [Google Scholar]
- DuBose T. D., Jr, Pucacco L. R., Carter N. W. Determination of disequilibrium pH in the rat kidney in vivo: evidence of hydrogen secretion. Am J Physiol. 1981 Feb;240(2):F138–F146. doi: 10.1152/ajprenal.1981.240.2.F138. [DOI] [PubMed] [Google Scholar]
- DuBose T. D., Jr, Pucacco L. R., Seldin D. W., Carter N. W., Kokko J. P. Microelectrode determination of pH and PCO2 in rat proximal tubule after benzolamide: evidence for hydrogen ion secretion. Kidney Int. 1979 Jun;15(6):624–629. doi: 10.1038/ki.1979.82. [DOI] [PubMed] [Google Scholar]
- Forbush B., 3rd Assay of Na,K-ATPase in plasma membrane preparations: increasing the permeability of membrane vesicles using sodium dodecyl sulfate buffered with bovine serum albumin. Anal Biochem. 1983 Jan;128(1):159–163. doi: 10.1016/0003-2697(83)90356-1. [DOI] [PubMed] [Google Scholar]
- Grassl S. M., Aronson P. S. Na+/HCO3-co-transport in basolateral membrane vesicles isolated from rabbit renal cortex. J Biol Chem. 1986 Jul 5;261(19):8778–8783. [PubMed] [Google Scholar]
- Jentsch T. J., Keller S. K., Koch M., Wiederholt M. Evidence for coupled transport of bicarbonate and sodium in cultured bovine corneal endothelial cells. J Membr Biol. 1984;81(3):189–204. doi: 10.1007/BF01868713. [DOI] [PubMed] [Google Scholar]
- Jentsch T. J., Schill B. S., Schwartz P., Matthes H., Keller S. K., Wiederholt M. Kidney epithelial cells of monkey origin (BSC-1) express a sodium bicarbonate cotransport. Characterization by 22Na+ flux measurements. J Biol Chem. 1985 Dec 15;260(29):15554–15560. [PubMed] [Google Scholar]
- Jentsch T. J., Schwartz P., Schill B. S., Langner B., Lepple A. P., Keller S. K., Wiederholt M. Kinetic properties of the sodium bicarbonate (carbonate) symport in monkey kidney epithelial cells (BSC-1). Interactions between Na+, HCO-3, and pH. J Biol Chem. 1986 Aug 15;261(23):10673–10679. [PubMed] [Google Scholar]
- Jentsch T. J., Stahlknecht T. R., Hollwede H., Fischer D. G., Keller S. K., Wiederholt M. A bicarbonate-dependent process inhibitable by disulfonic stilbenes and a Na+/H+ exchange mediate 22Na+ uptake into cultured bovine corneal endothelium. J Biol Chem. 1985 Jan 25;260(2):795–801. [PubMed] [Google Scholar]
- Kleinman J. G., Brown W. W., Ware R. A., Schwartz J. H. Cell pH and acid transport in renal cortical tissue. Am J Physiol. 1980 Nov;239(5):F440–F444. doi: 10.1152/ajprenal.1980.239.5.F440. [DOI] [PubMed] [Google Scholar]
- Krapf R., Alpern R. J., Rector F. C., Jr, Berry C. A. Basolateral membrane Na/base cotransport is dependent on CO2/HCO3 in the proximal convoluted tubule. J Gen Physiol. 1987 Dec;90(6):833–853. doi: 10.1085/jgp.90.6.833. [DOI] [PMC free article] [PubMed] [Google Scholar]
- Lucci M. S., Pucacco L. R., DuBose T. D., Jr, Kokko J. P., Carter N. W. Direct evaluation of acidification by rat proximal tubule: role of carbonic anhydrase. Am J Physiol. 1980 May;238(5):F372–F379. doi: 10.1152/ajprenal.1980.238.5.F372. [DOI] [PubMed] [Google Scholar]
- Lucci M. S., Pucacco L. R., DuBose T. D., Jr, Kokko J. P., Carter N. W. Direct evaluation of acidification by rat proximal tubule: role of carbonic anhydrase. Am J Physiol. 1980 May;238(5):F372–F379. doi: 10.1152/ajprenal.1980.238.5.F372. [DOI] [PubMed] [Google Scholar]
- Lucci M. S., Tinker J. P., Weiner I. M., DuBose T. D., Jr Function of proximal tubule carbonic anhydrase defined by selective inhibition. Am J Physiol. 1983 Oct;245(4):F443–F449. doi: 10.1152/ajprenal.1983.245.4.F443. [DOI] [PubMed] [Google Scholar]
- Lucci M. S., Warnock D. G., Rector F. C., Jr Carbonic anhydrase-dependent bicarbonate reabsorption in the rat proximal tubule. Am J Physiol. 1979 Jan;236(1):F58–F65. doi: 10.1152/ajprenal.1979.236.1.F58. [DOI] [PubMed] [Google Scholar]
- Marín R., Proverbio T., Proverbio F. Inside-out basolateral plasma membrane vesicles from rat kidney proximal tubular cells. Biochim Biophys Acta. 1986 Jun 13;858(1):195–201. doi: 10.1016/0005-2736(86)90306-8. [DOI] [PubMed] [Google Scholar]
- McKinney T. D., Burg M. B. Biocarbonate and fluid absorption by renal proximal straight tubules. Kidney Int. 1977 Jul;12(1):1–8. doi: 10.1038/ki.1977.72. [DOI] [PubMed] [Google Scholar]
- RECTOR F. C., Jr, CARTER N. W., SELDIN D. W. THE MECHANISM OF BICARBONATE REABSORPTION IN THE PROXIMAL AND DISTAL TUBULES OF THE KIDNEY. J Clin Invest. 1965 Feb;44:278–290. doi: 10.1172/JCI105142. [DOI] [PMC free article] [PubMed] [Google Scholar]
- Sasaki S., Shigai T., Takeuchi J. Intracellular pH in the isolated perfused rabbit proximal straight tubule. Am J Physiol. 1985 Sep;249(3 Pt 2):F417–F423. doi: 10.1152/ajprenal.1985.249.3.F417. [DOI] [PubMed] [Google Scholar]
- Soleimani M., Grassi S. M., Aronson P. S. Stoichiometry of Na+-HCO-3 cotransport in basolateral membrane vesicles isolated from rabbit renal cortex. J Clin Invest. 1987 Apr;79(4):1276–1280. doi: 10.1172/JCI112948. [DOI] [PMC free article] [PubMed] [Google Scholar]
- Struyvenberg A., Morrison R. B., Relman A. S. Acid-base behavior of separated canine renal tubule cells. Am J Physiol. 1968 May;214(5):1155–1162. doi: 10.1152/ajplegacy.1968.214.5.1155. [DOI] [PubMed] [Google Scholar]
- Ullrich K. J., Rumrich G., Baumann K. Renal proximal tubular buffer-(glycodiazine) transport. Inhomogeneity of local transport rate, dependence on sodium, effect of inhibitors and chronic adaptation. Pflugers Arch. 1975 Jun 26;357(3-4):149–163. doi: 10.1007/BF00585971. [DOI] [PubMed] [Google Scholar]
- Wistrand P. J., Kinne R. Carbonic anhydrase activity of isolated brush border and basal-lateral membranes of renal tubular cells. Pflugers Arch. 1977 Aug 29;370(2):121–126. doi: 10.1007/BF00581684. [DOI] [PubMed] [Google Scholar]
- Yoshitomi K., Burckhardt B. C., Frömter E. Rheogenic sodium-bicarbonate cotransport in the peritubular cell membrane of rat renal proximal tubule. Pflugers Arch. 1985 Dec;405(4):360–366. doi: 10.1007/BF00595689. [DOI] [PubMed] [Google Scholar]