Abstract
Many oligomeric enzymes are functional only in the assembled form, and it is often difficult to determine unambiguously why monomers are inactive. In some cases individual monomers cannot fold into stable correct ("native") conformations without contributions from interchain interactions. For other oligomers, catalysis requires the contributions of amino acid residues at the interface between adjacent polypeptide chains, and monomers are inactive because they cannot form complete active sites. A test for the presence of shared sites was devised that is based on the formation of active hybrid oligomers from appropriate inactive parental mutants produced by site-directed mutagenesis. This approach was applied in a study of the catalytic trimer of aspartate transcarbamoylase (aspartate carbamoyltransferase, EC 2.1.3.2) from Escherichia coli, using three mutants, in which Ser-52 was replaced by His, Lys-84 by Gln, or His-134 by Ala. Hybrid trimers formed from the virtually inactive Ser and Lys mutants were 10(5) more active than the parental proteins, and the specific activities of each hybrid were about 33% that of the wild-type trimer, as expected for the scheme based on shared sites. Hybrids from the His and Lys mutants had comparable specific activities. Moreover, one hybrid with approximately 33% activity had one high-affinity binding site for a bisubstrate analog as compared to about three for wild-type trimer. As a further test, hybrids were also formed from wild-type and double-mutant (Lys-84----Gln and His-134----Ala) trimers. The hybrid containing two chains with the double mutation and one wild-type chain had very little activity, and that composed of one double mutant and two wild-type chains had 32% the specific activity of wild-type trimers. This negative complementation experiment is in quantitative accord with the scheme based on shared sites at or near the interfaces between adjacent chains. The techniques used to demonstrate shared active sites in the catalytic subunits of aspartate transcarbamoylase can be applied generally to various types of oligomers (dimers, tetramers, etc.) to determine whether the participation of amino acid residues from adjoining chains is essential for forming active sites in oligomeric enzymes.
Full text
PDF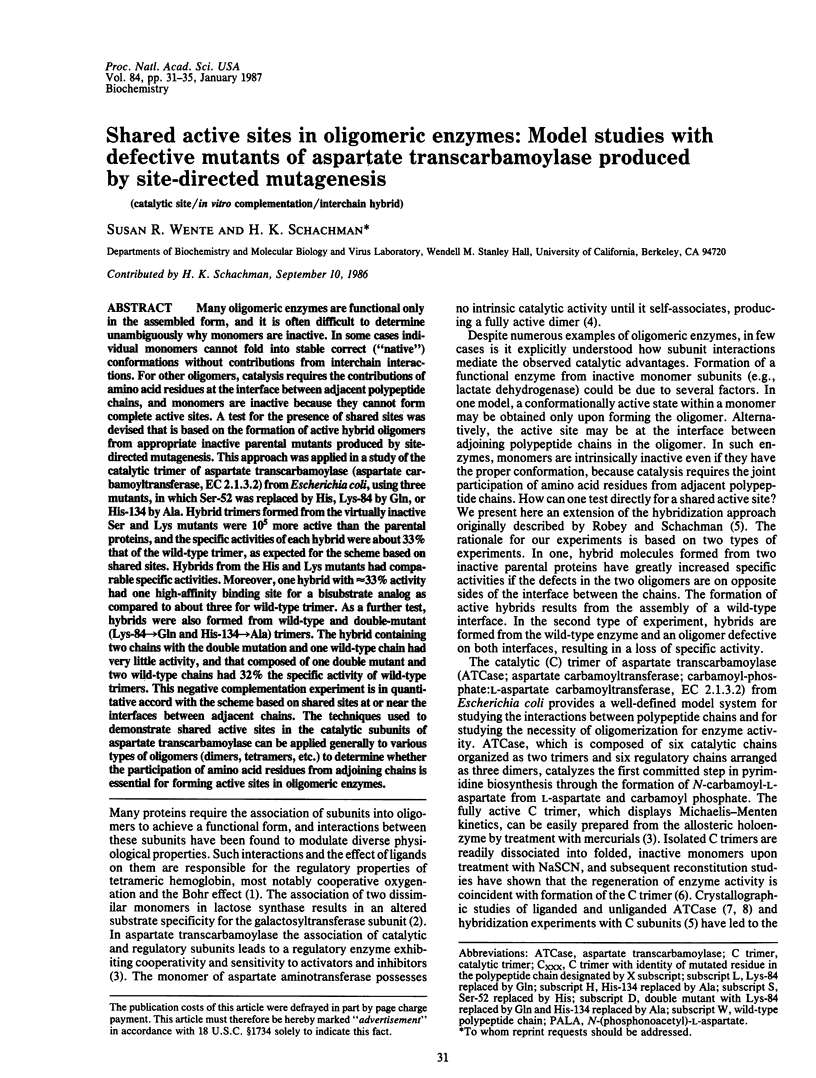
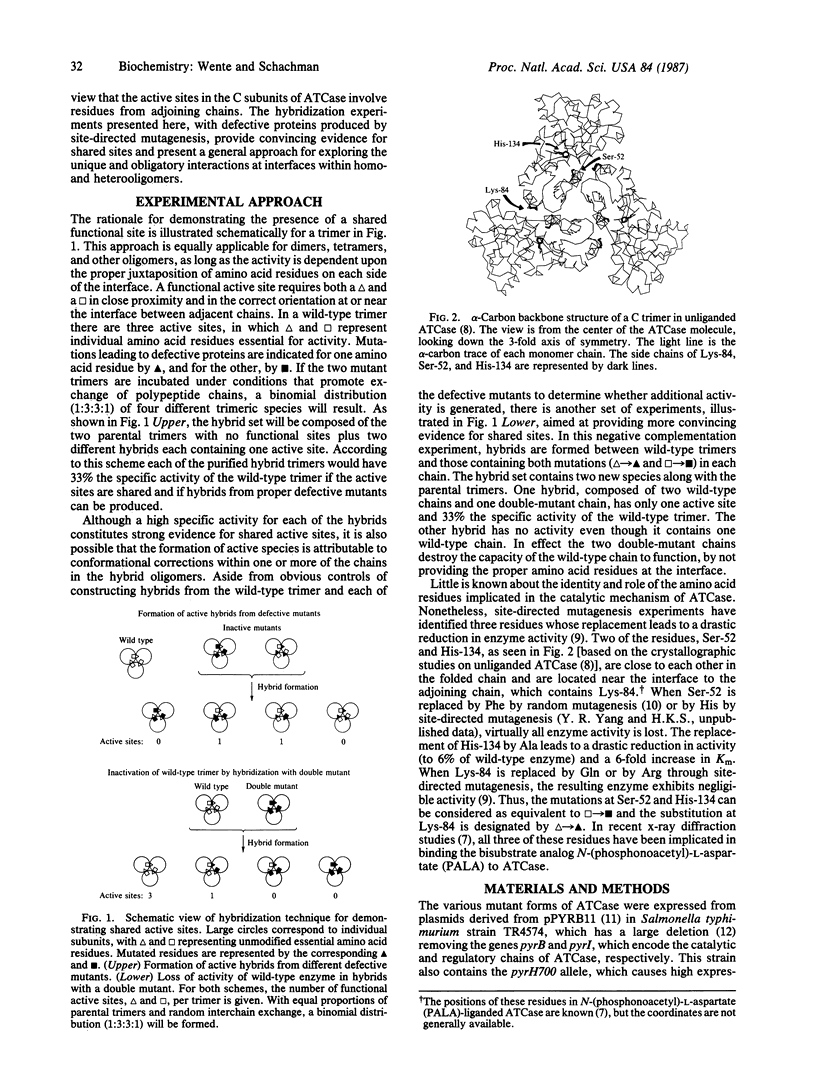
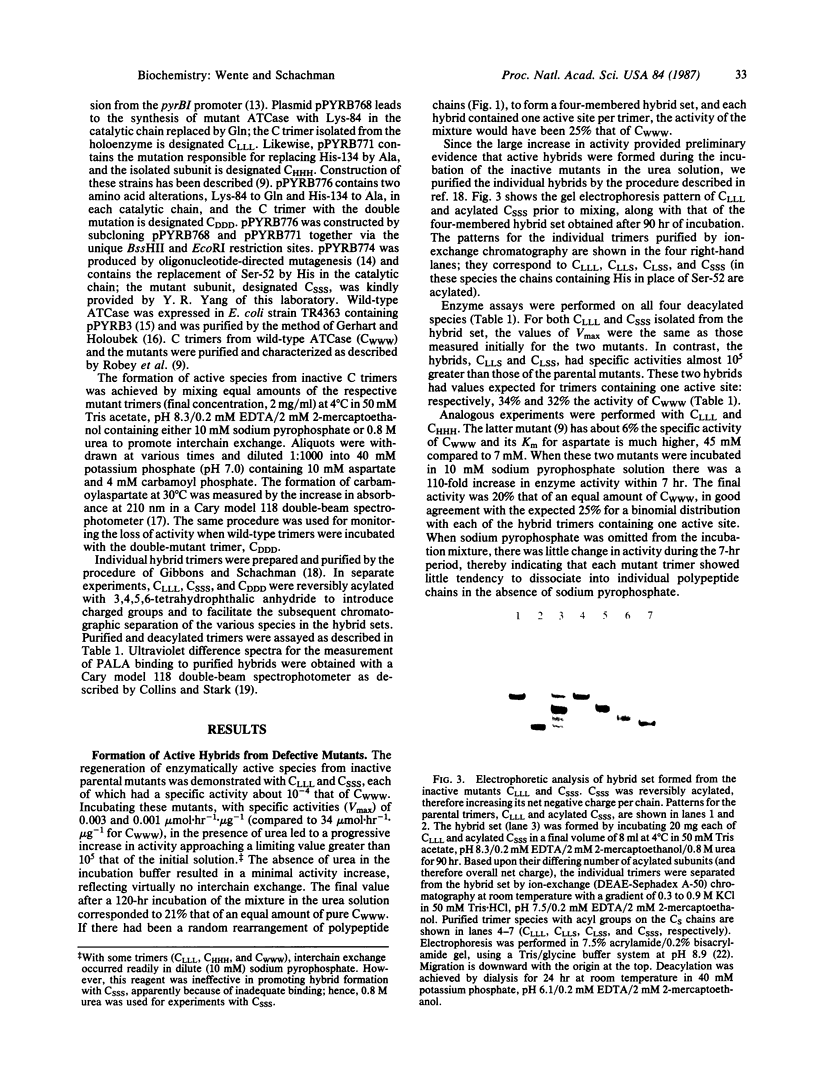
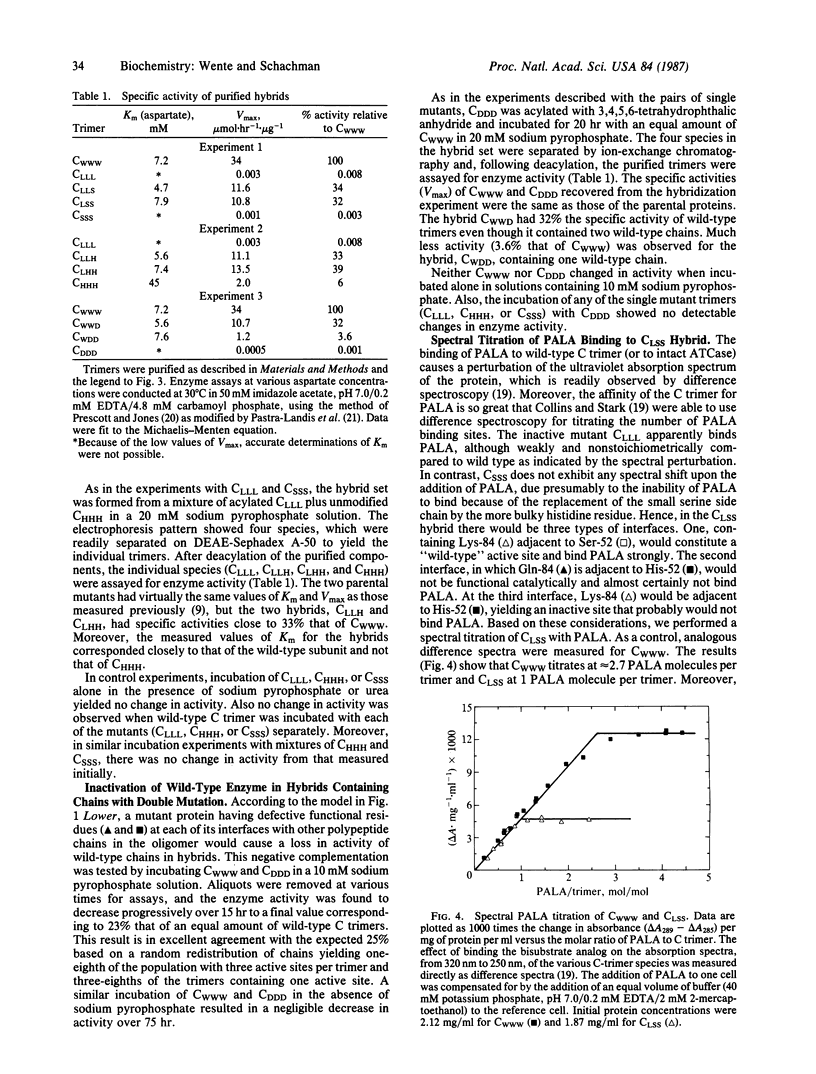
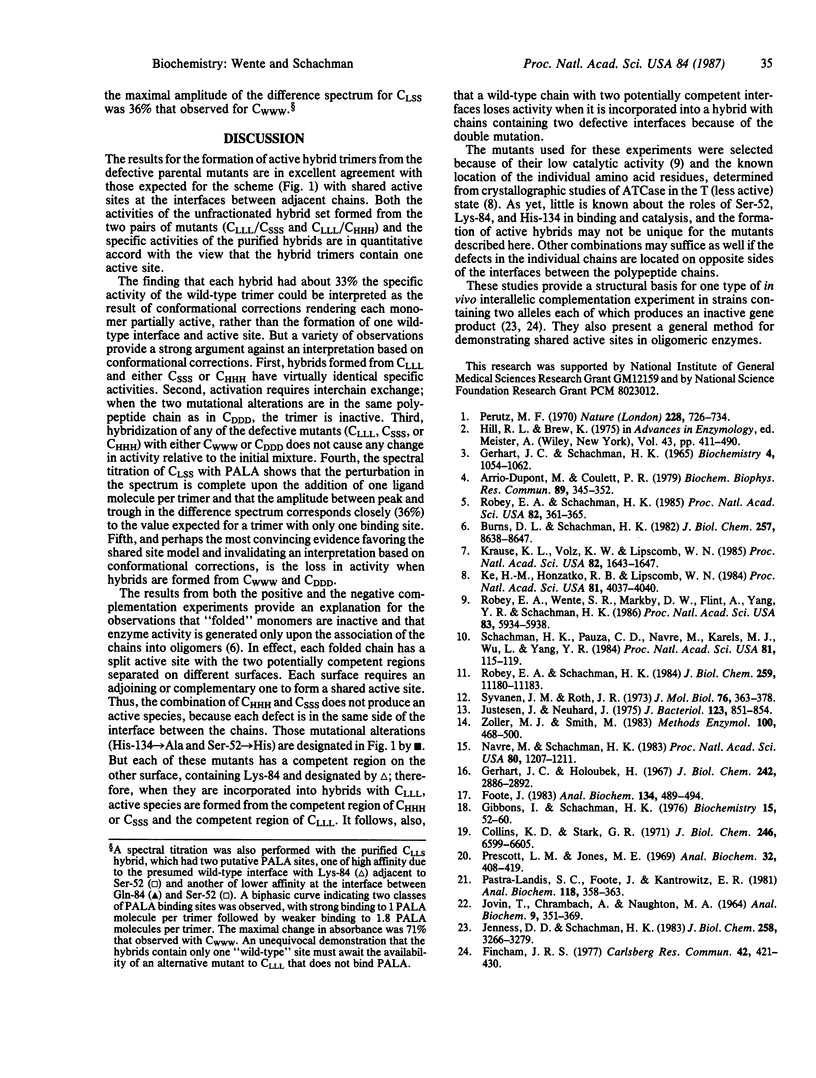
Images in this article
Selected References
These references are in PubMed. This may not be the complete list of references from this article.
- Arrio-Dupont M., Coulet P. R. Aspartate aminotransferase immobilized on collagen films. Activity of dissociated subunits. Biochem Biophys Res Commun. 1979 Jul 27;89(2):345–352. doi: 10.1016/0006-291x(79)90636-3. [DOI] [PubMed] [Google Scholar]
- Burns D. L., Schachman H. K. Assembly of the catalytic trimers of aspartate transcarbamoylase from folded monomers. J Biol Chem. 1982 Aug 10;257(15):8638–8647. [PubMed] [Google Scholar]
- Collins K. D., Stark G. R. Aspartate transcarbamylase. Interaction with the transition state analogue N-(phosphonacetyl)-L-aspartate. J Biol Chem. 1971 Nov;246(21):6599–6605. [PubMed] [Google Scholar]
- Foote J. An ultraviolet spectrophotometric assay for aspartate transcarbamylase. Anal Biochem. 1983 Oct 15;134(2):489–494. doi: 10.1016/0003-2697(83)90328-7. [DOI] [PubMed] [Google Scholar]
- Gerhart J. C., Holoubek H. The purification of aspartate transcarbamylase of Escherichia coli and separation of its protein subunits. J Biol Chem. 1967 Jun 25;242(12):2886–2892. [PubMed] [Google Scholar]
- Gerhart J. C., Schachman H. K. Distinct subunits for the regulation and catalytic activity of aspartate transcarbamylase. Biochemistry. 1965 Jun;4(6):1054–1062. doi: 10.1021/bi00882a012. [DOI] [PubMed] [Google Scholar]
- Gibbons I., Schachman H. K. A method for the separation of hybrids of chromatographically identical oligomeric proteins. Use of 3,4,5,6-tetrahydrophthaloyl groups as a reversible "chromatographic handle". Biochemistry. 1976 Jan 13;15(1):52–60. doi: 10.1021/bi00646a009. [DOI] [PubMed] [Google Scholar]
- Hill R. L., Brew K. Lactose synthetase. Adv Enzymol Relat Areas Mol Biol. 1975;43:411–490. doi: 10.1002/9780470122884.ch5. [DOI] [PubMed] [Google Scholar]
- JOVIN T., CHRAMBACH A., NAUGHTON M. A. AN APPARATUS FOR PREPARATIVE TEMPERATURE-REGULATED POLYACRYLAMIDE GEL ELECTROPHORESIS. Anal Biochem. 1964 Nov;9:351–369. doi: 10.1016/0003-2697(64)90192-7. [DOI] [PubMed] [Google Scholar]
- Jenness D. D., Schachman H. K. Genetic characterization of the folding domains of the catalytic chains in aspartate transcarbamoylase. J Biol Chem. 1983 Mar 10;258(5):3266–3279. [PubMed] [Google Scholar]
- Justesen J., Neuhard J. pyrR identical to pyrH in Salmonella typhimurium: control of expression of the pyr genes. J Bacteriol. 1975 Sep;123(3):851–854. doi: 10.1128/jb.123.3.851-854.1975. [DOI] [PMC free article] [PubMed] [Google Scholar]
- Ke H. M., Honzatko R. B., Lipscomb W. N. Structure of unligated aspartate carbamoyltransferase of Escherichia coli at 2.6-A resolution. Proc Natl Acad Sci U S A. 1984 Jul;81(13):4037–4040. doi: 10.1073/pnas.81.13.4037. [DOI] [PMC free article] [PubMed] [Google Scholar]
- Krause K. L., Volz K. W., Lipscomb W. N. Structure at 2.9-A resolution of aspartate carbamoyltransferase complexed with the bisubstrate analogue N-(phosphonacetyl)-L-aspartate. Proc Natl Acad Sci U S A. 1985 Mar;82(6):1643–1647. doi: 10.1073/pnas.82.6.1643. [DOI] [PMC free article] [PubMed] [Google Scholar]
- Navre M., Schachman H. K. Synthesis of aspartate transcarbamoylase in Escherichia coli: transcriptional regulation of the pyrB-pyrI operon. Proc Natl Acad Sci U S A. 1983 Mar;80(5):1207–1211. doi: 10.1073/pnas.80.5.1207. [DOI] [PMC free article] [PubMed] [Google Scholar]
- Pastra-Landis S. C., Foote J., Kantrowitz E. R. An improved colorimetric assay for aspartate and ornithine transcarbamylases. Anal Biochem. 1981 Dec;118(2):358–363. doi: 10.1016/0003-2697(81)90594-7. [DOI] [PubMed] [Google Scholar]
- Perutz M. F. Stereochemistry of cooperative effects in haemoglobin. Nature. 1970 Nov 21;228(5273):726–739. doi: 10.1038/228726a0. [DOI] [PubMed] [Google Scholar]
- Prescott L. M., Jones M. E. Modified methods for the determination of carbamyl aspartate. Anal Biochem. 1969 Dec;32(3):408–419. doi: 10.1016/s0003-2697(69)80008-4. [DOI] [PubMed] [Google Scholar]
- Robey E. A., Schachman H. K. Regeneration of active enzyme by formation of hybrids from inactive derivatives: implications for active sites shared between polypeptide chains of aspartate transcarbamoylase. Proc Natl Acad Sci U S A. 1985 Jan;82(2):361–365. doi: 10.1073/pnas.82.2.361. [DOI] [PMC free article] [PubMed] [Google Scholar]
- Robey E. A., Schachman H. K. Site-specific mutagenesis of aspartate transcarbamoylase. Replacement of tyrosine 165 in the catalytic chain by serine reduces enzymatic activity. J Biol Chem. 1984 Sep 25;259(18):11180–11183. [PubMed] [Google Scholar]
- Robey E. A., Wente S. R., Markby D. W., Flint A., Yang Y. R., Schachman H. K. Effect of amino acid substitutions on the catalytic and regulatory properties of aspartate transcarbamoylase. Proc Natl Acad Sci U S A. 1986 Aug;83(16):5934–5938. doi: 10.1073/pnas.83.16.5934. [DOI] [PMC free article] [PubMed] [Google Scholar]
- Schachman H. K., Pauza C. D., Navre M., Karels M. J., Wu L., Yang Y. R. Location of amino acid alterations in mutants of aspartate transcarbamoylase: Structural aspects of interallelic complementation. Proc Natl Acad Sci U S A. 1984 Jan;81(1):115–119. doi: 10.1073/pnas.81.1.115. [DOI] [PMC free article] [PubMed] [Google Scholar]
- Syvanen J. M., Roth J. R. Structural genes for catalytic and regulatory subunits of aspartate transcarbamylase. J Mol Biol. 1973 May 25;76(3):363–378. doi: 10.1016/0022-2836(73)90510-x. [DOI] [PubMed] [Google Scholar]
- Zoller M. J., Smith M. Oligonucleotide-directed mutagenesis of DNA fragments cloned into M13 vectors. Methods Enzymol. 1983;100:468–500. doi: 10.1016/0076-6879(83)00074-9. [DOI] [PubMed] [Google Scholar]