Abstract
Neutral salts exhibit very marked differences as eluants of proteins from affinity columns. We observe: (i) that the relative potencies of neutral salts as eluants are independent of the protein or the affinity ligand in the systems studied, (ii) that the absolute salt concentration necessary to elute any given protein bound to the affinity matrix is proportional to the algebraic sum of a set of elution coefficients defined herein for the separate ions present in the solution, and (iii) that the proportionality between elution potency and elution coefficient is a function of the affinity of the protein for the immobilized ligand. Given the concentration of one neutral salt required for elution of a protein of interest from an affinity column, the elution capability of any neutral salt at any temperature can be quantitatively predicted for that protein. Accordingly, application and elution protocols for affinity chromatography can be designed to optimize the yield and fold purification of proteins.
Full text
PDF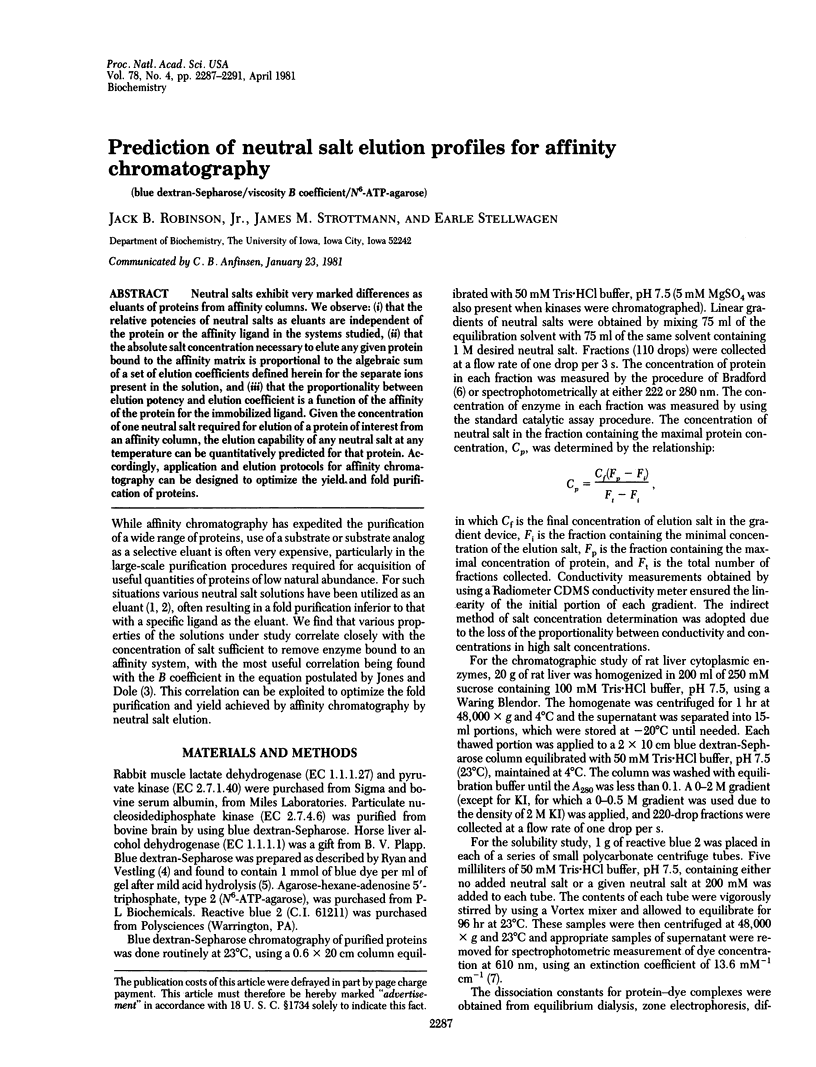
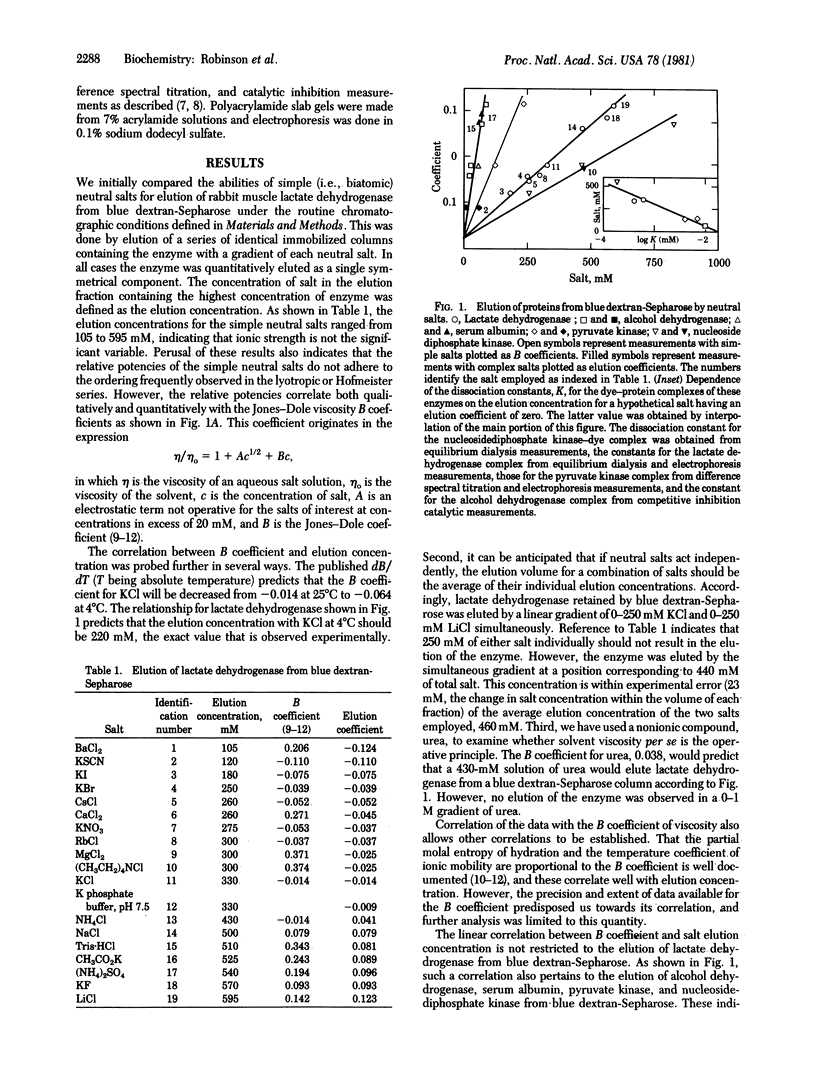
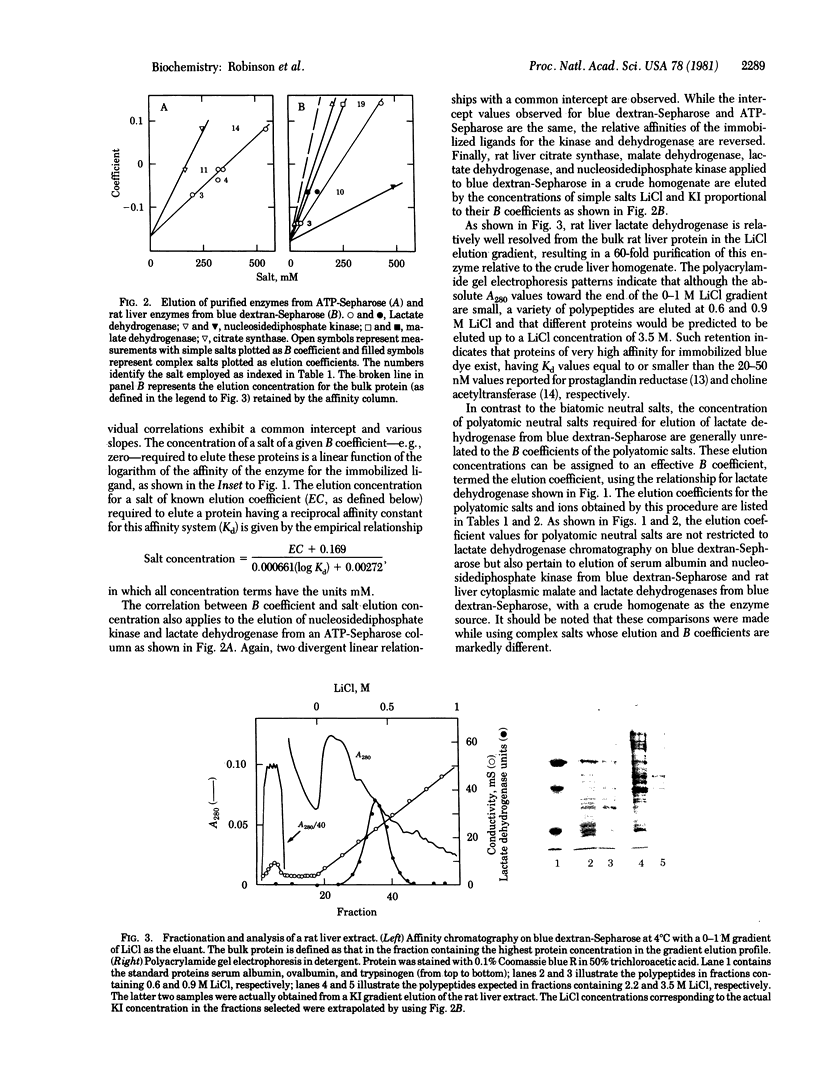
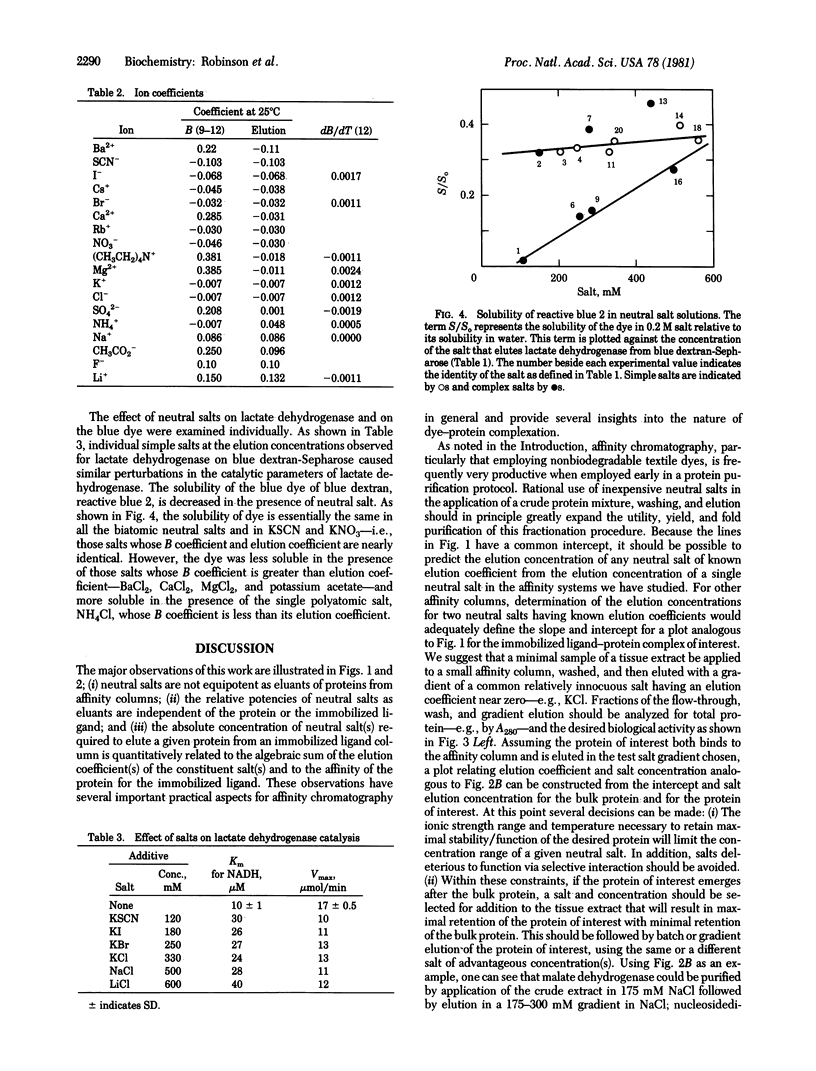
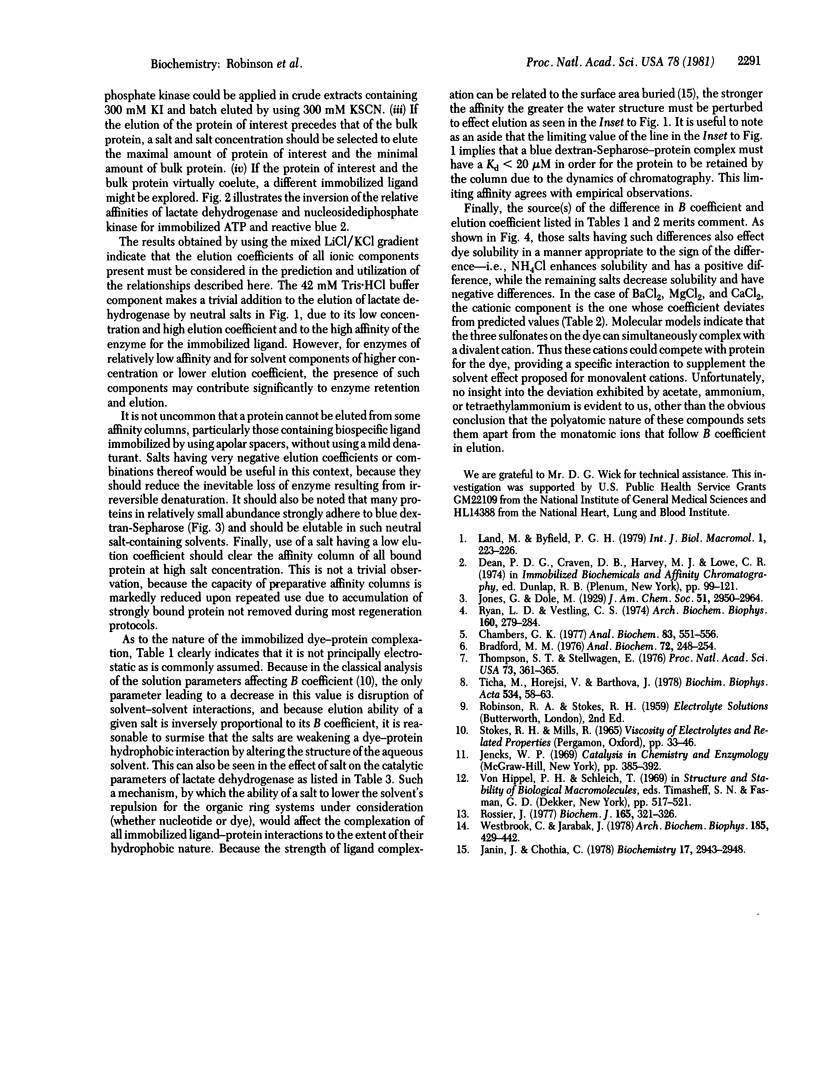
Images in this article
Selected References
These references are in PubMed. This may not be the complete list of references from this article.
- Bradford M. M. A rapid and sensitive method for the quantitation of microgram quantities of protein utilizing the principle of protein-dye binding. Anal Biochem. 1976 May 7;72:248–254. doi: 10.1006/abio.1976.9999. [DOI] [PubMed] [Google Scholar]
- Chambers G. K. Determination of Cibacron Blue F3gA substitution in blue Sephadex and blue dextran-Sepharose. Anal Biochem. 1977 Dec;83(2):551–556. doi: 10.1016/0003-2697(77)90058-6. [DOI] [PubMed] [Google Scholar]
- Dean P. D., Craven D. B., Harvey M. J., Lowe C. R. An analysis of affinity chromatography using immobilised alkyl nucleotides. Adv Exp Med Biol. 1974;42(0):99–121. doi: 10.1007/978-1-4684-6982-0_8. [DOI] [PubMed] [Google Scholar]
- Janin J., Chothia C. Role of hydrophobicity in the binding of coenzymes. Appendix. Translational and rotational contribution to the free energy of dissociation. Biochemistry. 1978 Jul 25;17(15):2943–2948. doi: 10.1021/bi00608a001. [DOI] [PubMed] [Google Scholar]
- Rossier J. Acetyl-coenzyme A and coenzyme A analogues. Their effects on rat brain choline acetyltransferase. Biochem J. 1977 Aug 1;165(2):321–326. doi: 10.1042/bj1650321. [DOI] [PMC free article] [PubMed] [Google Scholar]
- Ryan L. D., Vestling C. S. Rapid purification of lactate dehydrogenase from rat liver and hepatoma: a new approach. Arch Biochem Biophys. 1974 Jan;160(1):279–284. doi: 10.1016/s0003-9861(74)80035-4. [DOI] [PubMed] [Google Scholar]
- Thompson S. T., Stellwagen E. Binding of Cibacron blue F3GA to proteins containing the dinucleotide fold. Proc Natl Acad Sci U S A. 1976 Feb;73(2):361–365. doi: 10.1073/pnas.73.2.361. [DOI] [PMC free article] [PubMed] [Google Scholar]
- Tichá M., Horejsí V., Barthová J. Affinity electrophoresis of proteins interacting with Blue dextran. Biochim Biophys Acta. 1978 May 24;534(1):58–63. doi: 10.1016/0005-2795(78)90475-0. [DOI] [PubMed] [Google Scholar]
- Westbrook C., Jarabak J. 15-ketoprostaglandin delta13 reductase from human placenta: purification, kinetics, and inhibitor binding. Arch Biochem Biophys. 1978 Jan 30;185(2):429–442. doi: 10.1016/0003-9861(78)90186-8. [DOI] [PubMed] [Google Scholar]