Abstract
A procedure, called "mosaic nonequilibrium thermodynamics," for describing ion movement and energy transduction in biological membranes is tested in a model system: bacteriorhodopsin liposomes. The important steps in the theoretical derivations are summarized; one of the experimental tests of the postulated fundamental flow-force relationships is shown. Furthermore, how the quantitative method, even if used only qualitatively, facilitates analysis and understanding of experimental results (in this case, the effect of medium composition on the development of pH gradient and membrane potential in the bacteriorhodopsin liposomes) is shown. The main advantage of this method lies in its quantitative description of the effect of variation of system parameters on the performance of, in this case, the reconstituted proton pump bacteriorhodopsin. As an example, the method is shown to explain quantitatively the dependence of the steady-state pH gradient on the light intensity. Even in more refined analyses of experiments, the quantitative theoretical description is in full accordance with the experimental results; this is illustrated by considering the effect of valinomycin on the dependence of the initial rate of proton uptake into bacteriorhodopsin liposomes on light intensity. It is concluded that mosaic nonequilibrium thermodynamics describes ion movement and energy transduction in the model system of bacteriorhodopsin liposomes and, therefore, may be applied to any other biological system performing such processes.
Full text
PDF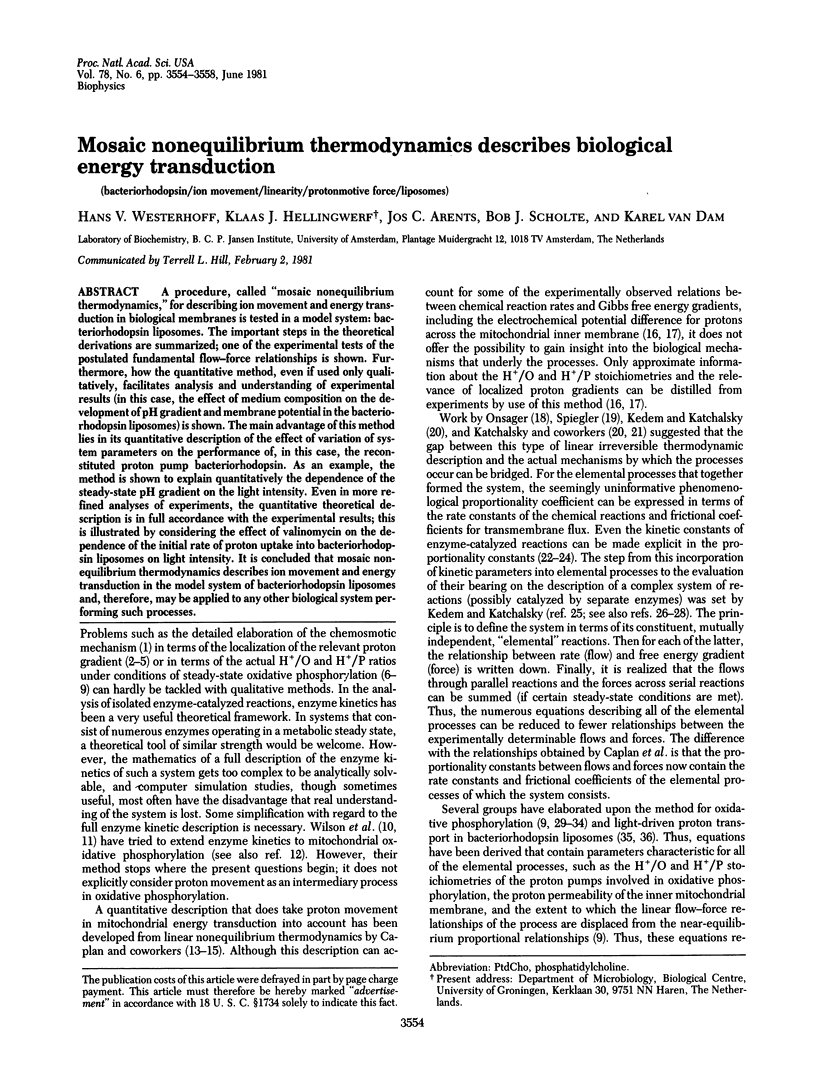
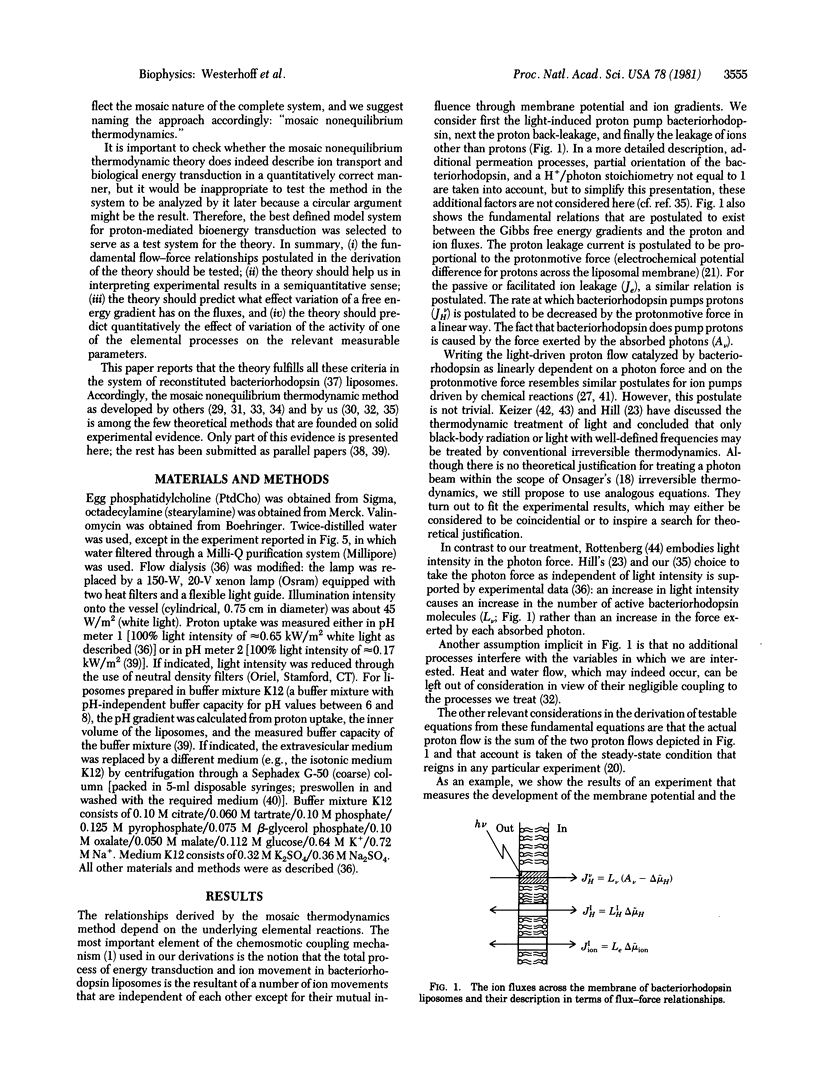
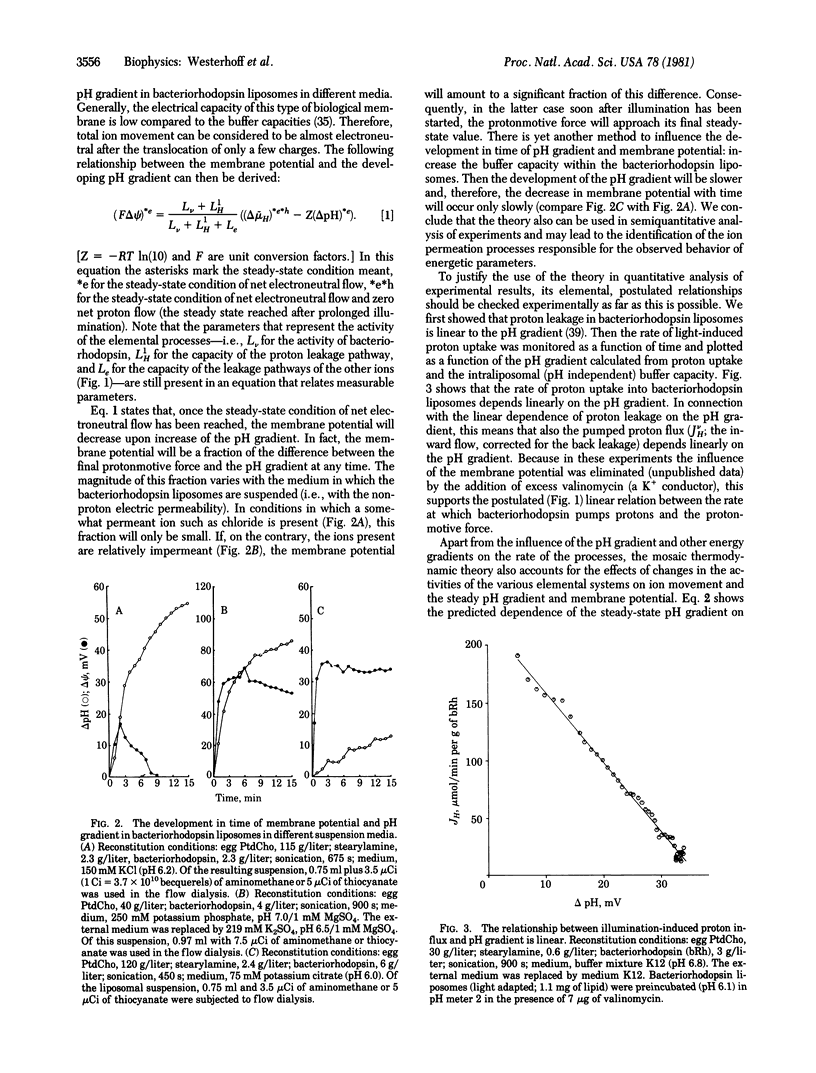
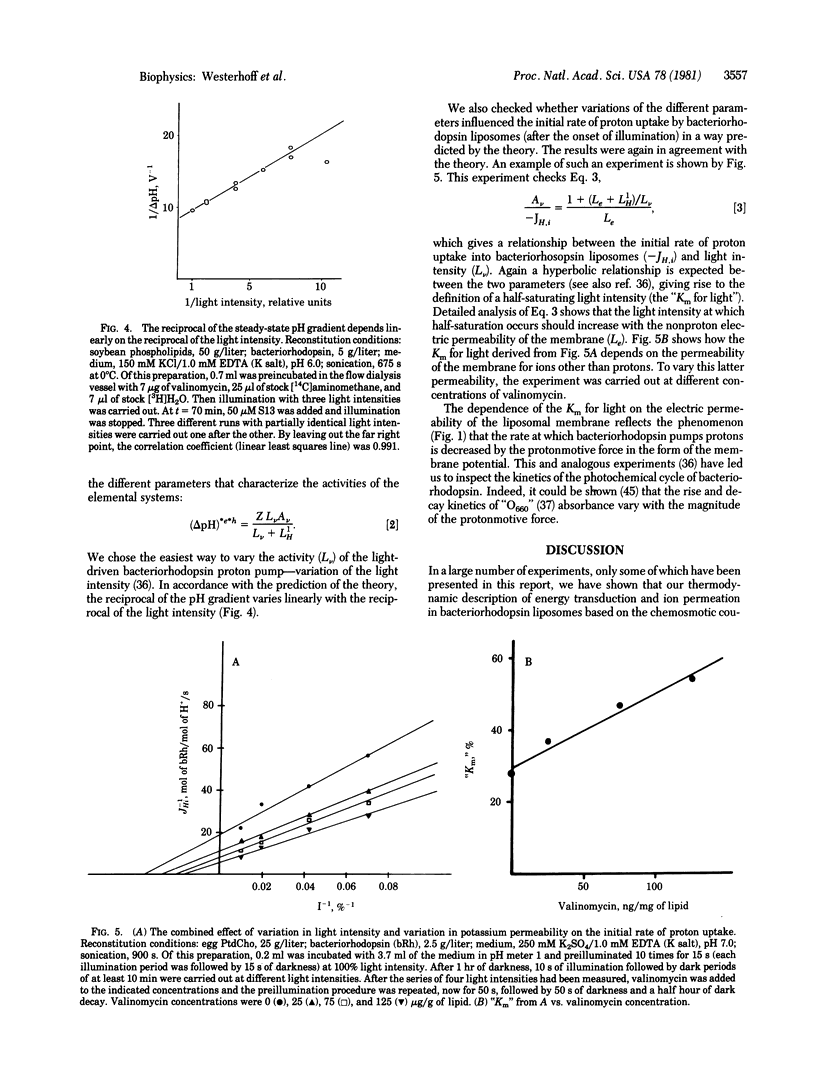
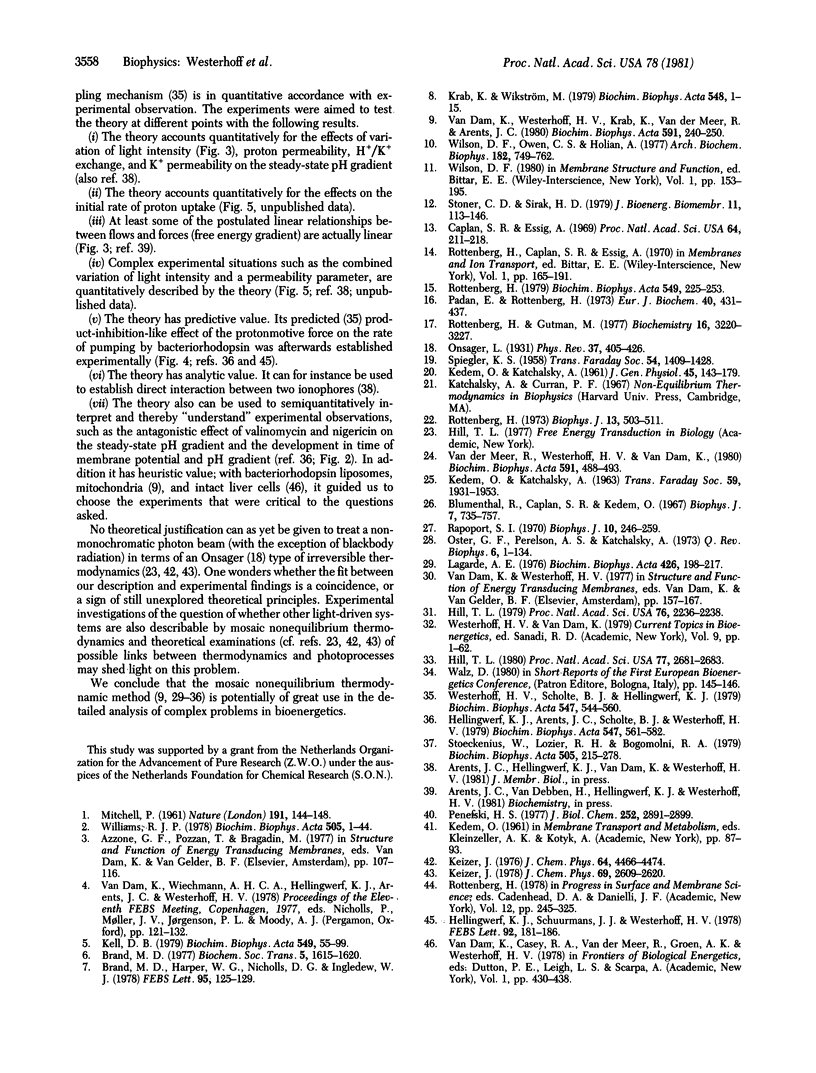
Selected References
These references are in PubMed. This may not be the complete list of references from this article.
- Blumenthal R., Caplan S. R., Kedem O. The coupling of an enzymatic reaction to transmembrane flow of electric current in a synthetic "active transport" system. Biophys J. 2008 Dec 31;7(6):735–757. doi: 10.1016/S0006-3495(67)86620-7. [DOI] [PMC free article] [PubMed] [Google Scholar]
- Brand M. D., Harper W. G., Nicholls D. G., Ingledew W. J. Unequal charge separation by different coupling spans of the mitochondrial electron transport chain. FEBS Lett. 1978 Nov 1;95(1):125–129. doi: 10.1016/0014-5793(78)80066-0. [DOI] [PubMed] [Google Scholar]
- Brand M. D. The stoicheiometric relationships between electron transport, proton translocation and adenosine triphosphate synthesis and hydrolysis in mitochondria. Biochem Soc Trans. 1977;5(5):1615–1620. doi: 10.1042/bst0051615. [DOI] [PubMed] [Google Scholar]
- Caplan S. R., Essig A. Oxidative phosphorylation: thermodynamic criteria for the chemical and chemiosmotic hypotheses. Proc Natl Acad Sci U S A. 1969 Sep;64(1):211–218. doi: 10.1073/pnas.64.1.211. [DOI] [PMC free article] [PubMed] [Google Scholar]
- Hellingwerf K. J., Arents J. C., Scholte B. J., Westerhoff H. V. Bacteriorhodopsin in liposomes. II. Experimental evidence in support of a theoretical model. Biochim Biophys Acta. 1979 Sep 11;547(3):561–582. doi: 10.1016/0005-2728(79)90034-3. [DOI] [PubMed] [Google Scholar]
- Hellingwerf K. J., Schuurmans J. J., Westerhoff H. V. Demonstration of coupling between the protonmotive force across bacteriorhodopsin and the flow through its photochemical cycle. FEBS Lett. 1978 Aug 15;92(2):181–186. doi: 10.1016/0014-5793(78)80749-2. [DOI] [PubMed] [Google Scholar]
- Hill T. L. Steady-state coupling of four membrane systems in mitochondrial oxidative phosphorylation. Proc Natl Acad Sci U S A. 1979 May;76(5):2236–2238. doi: 10.1073/pnas.76.5.2236. [DOI] [PMC free article] [PubMed] [Google Scholar]
- Hill T. L. Steady-state properties of coupled systems in mitochondrial oxidative phosphorylation. Proc Natl Acad Sci U S A. 1980 May;77(5):2681–2683. doi: 10.1073/pnas.77.5.2681. [DOI] [PMC free article] [PubMed] [Google Scholar]
- KEDEM O., KATCHALSKY A. A physical interpretation of the phenomenological coefficients of membrane permeability. J Gen Physiol. 1961 Sep;45:143–179. doi: 10.1085/jgp.45.1.143. [DOI] [PMC free article] [PubMed] [Google Scholar]
- Kell D. B. On the functional proton current pathway of electron transport phosphorylation. An electrodic view. Biochim Biophys Acta. 1979 Jul 3;549(1):55–99. doi: 10.1016/0304-4173(79)90018-1. [DOI] [PubMed] [Google Scholar]
- Lagarde A. E. A non-equilibrium thermodynamics analysis of active transport within the framework of the chemiosotic theory. Biochim Biophys Acta. 1976 Mar 5;426(2):198–217. doi: 10.1016/0005-2736(76)90332-1. [DOI] [PubMed] [Google Scholar]
- MITCHELL P. Coupling of phosphorylation to electron and hydrogen transfer by a chemi-osmotic type of mechanism. Nature. 1961 Jul 8;191:144–148. doi: 10.1038/191144a0. [DOI] [PubMed] [Google Scholar]
- Oster G. F., Perelson A. S., Katchalsky A. Network thermodynamics: dynamic modelling of biophysical systems. Q Rev Biophys. 1973 Feb;6(1):1–134. doi: 10.1017/s0033583500000081. [DOI] [PubMed] [Google Scholar]
- Padan E., Rottenberg H. Respiratory control and the proton electrochemical gradient in mitochondria. Eur J Biochem. 1973 Dec 17;40(2):431–437. doi: 10.1111/j.1432-1033.1973.tb03212.x. [DOI] [PubMed] [Google Scholar]
- Penefsky H. S. Reversible binding of Pi by beef heart mitochondrial adenosine triphosphatase. J Biol Chem. 1977 May 10;252(9):2891–2899. [PubMed] [Google Scholar]
- Rapoport S. I. The sodium-potassium exchange pump: relation of metabolism to electrical properties of the cell. I. Theory. Biophys J. 1970 Mar;10(3):246–259. doi: 10.1016/S0006-3495(70)86297-X. [DOI] [PMC free article] [PubMed] [Google Scholar]
- Rottenberg H., Gutman M. Control of the rate of reverse electron transport in submitochondrial particles by the free energy. Biochemistry. 1977 Jul 12;16(14):3220–3227. doi: 10.1021/bi00633a028. [DOI] [PubMed] [Google Scholar]
- Rottenberg H. Non-equilibrium thermodynamics of energy conversion in bioenergetics. Biochim Biophys Acta. 1979 Dec 13;549(3-4):225–253. doi: 10.1016/0304-4173(79)90001-6. [DOI] [PubMed] [Google Scholar]
- Rottenberg H. The thermodynamic description of enzyme-catalyzed reactions. The linear relation between the reaction rate and the affinity. Biophys J. 1973 Jun;13(6):503–511. doi: 10.1016/S0006-3495(73)86004-7. [DOI] [PMC free article] [PubMed] [Google Scholar]
- Stoeckenius W., Lozier R. H., Bogomolni R. A. Bacteriorhodopsin and the purple membrane of halobacteria. Biochim Biophys Acta. 1979 Mar 14;505(3-4):215–278. doi: 10.1016/0304-4173(79)90006-5. [DOI] [PubMed] [Google Scholar]
- Stoner C. D., Sirak H. D. Steady-state kinetics of the overall oxidative phosphorylation reaction in heart mitochondria. J Bioenerg Biomembr. 1979 Dec;11(5-6):113–146. doi: 10.1007/BF00743199. [DOI] [PubMed] [Google Scholar]
- Van Dam K., Westerhoff H. V., Krab K., van der Meer R., Arents J. C. Relationship between chemiosomotic flows and thermodynamic forces in oxidative phosphorylation. Biochim Biophys Acta. 1980 Jul 8;591(2):240–250. doi: 10.1016/0005-2728(80)90156-5. [DOI] [PubMed] [Google Scholar]
- Westerhoff H. V., Scholte B. J., Hellingwerf K. J. Bacteriorhodopsin in liposomes. I. A description using irreversible thermodynamics. Biochim Biophys Acta. 1979 Sep 11;547(3):544–560. doi: 10.1016/0005-2728(79)90033-1. [DOI] [PubMed] [Google Scholar]
- Williams R. J. The multifarious couplings of energy transduction. Biochim Biophys Acta. 1978 Sep 21;505(1):1–44. doi: 10.1016/0304-4173(78)90007-1. [DOI] [PubMed] [Google Scholar]
- Wilson D. F., Owen C. S., Holian A. Control of mitochondrial respiration: a quantitative evaluation of the roles of cytochrome c and oxygen. Arch Biochem Biophys. 1977 Aug;182(2):749–762. doi: 10.1016/0003-9861(77)90557-4. [DOI] [PubMed] [Google Scholar]