Abstract
Oligonucleotide-directed mutagenesis was used to investigate the nature of transition state stabilization in the catalytic mechanism of the serine protease, subtilisin BPN'. The gene for this extracellular enzyme from Bacillus amyloliquefaciens has been cloned and expressed in Bacillus subtilis. In the transition state complex, the carbonyl group of the peptide bond to be hydrolyzed is believed to adopt a tetrahedral configuration rather than the ground-state planar configuration. Crystallographic studies suggest that stabilization of this activated complex is accomplished in part through the donation of a hydrogen bond from the amide side group of Asn-155 to the carbonyl oxygen of the peptide substrate. To specifically test this hypothesis, leucine was introduced at position 155. Leucine is isosteric with asparagine but is incapable of donating a hydrogen bond to the tetrahedral intermediate. The Leu-155 variant was found to have an unaltered Km but a greatly reduced catalytic rate constant, kcat, (factor of 200-300 smaller) when assayed with a peptide substrate. These kinetic results are consistent with the Asn-155 mediating stabilization of the activated complex and lend further experimental support for the transition-state stabilization hypothesis of enzyme catalysis.
Full text
PDF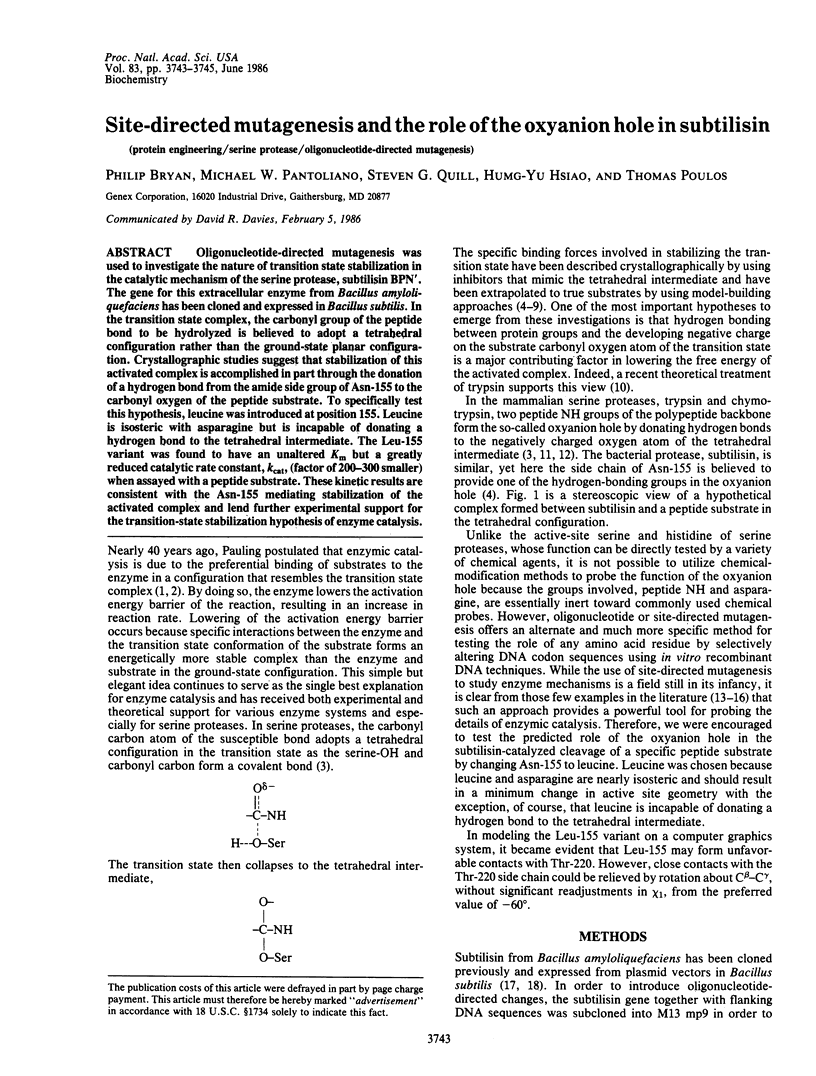
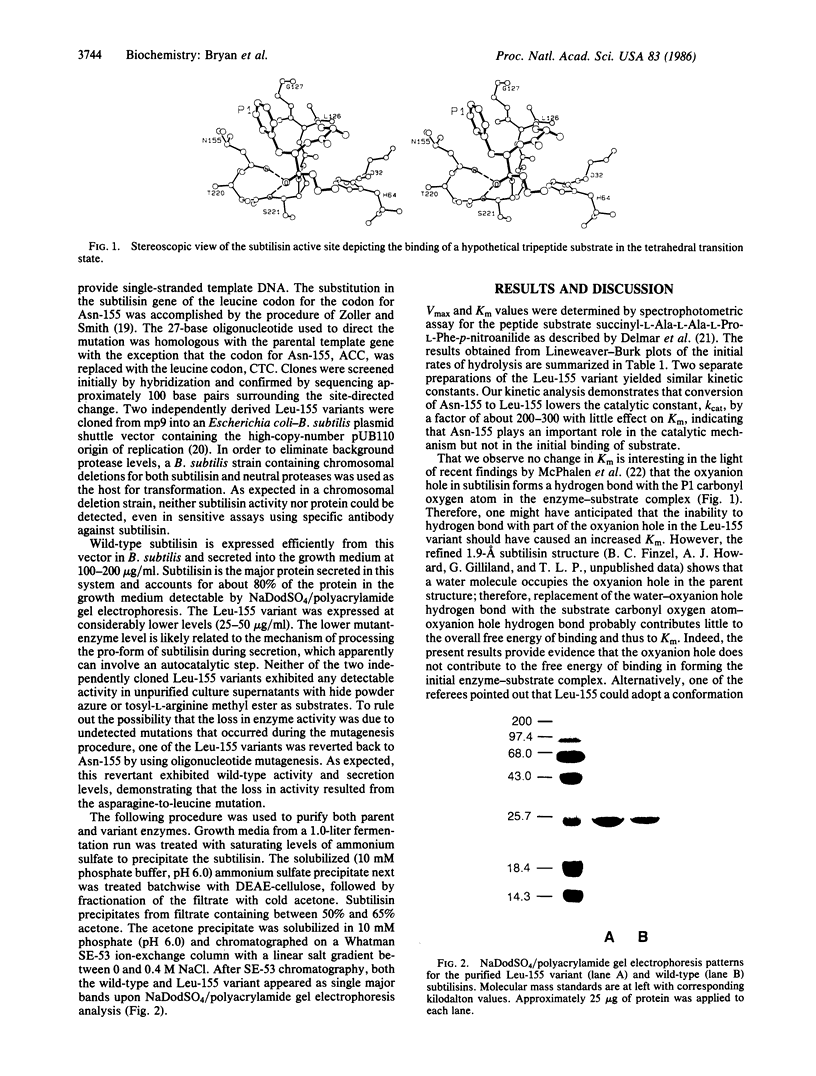
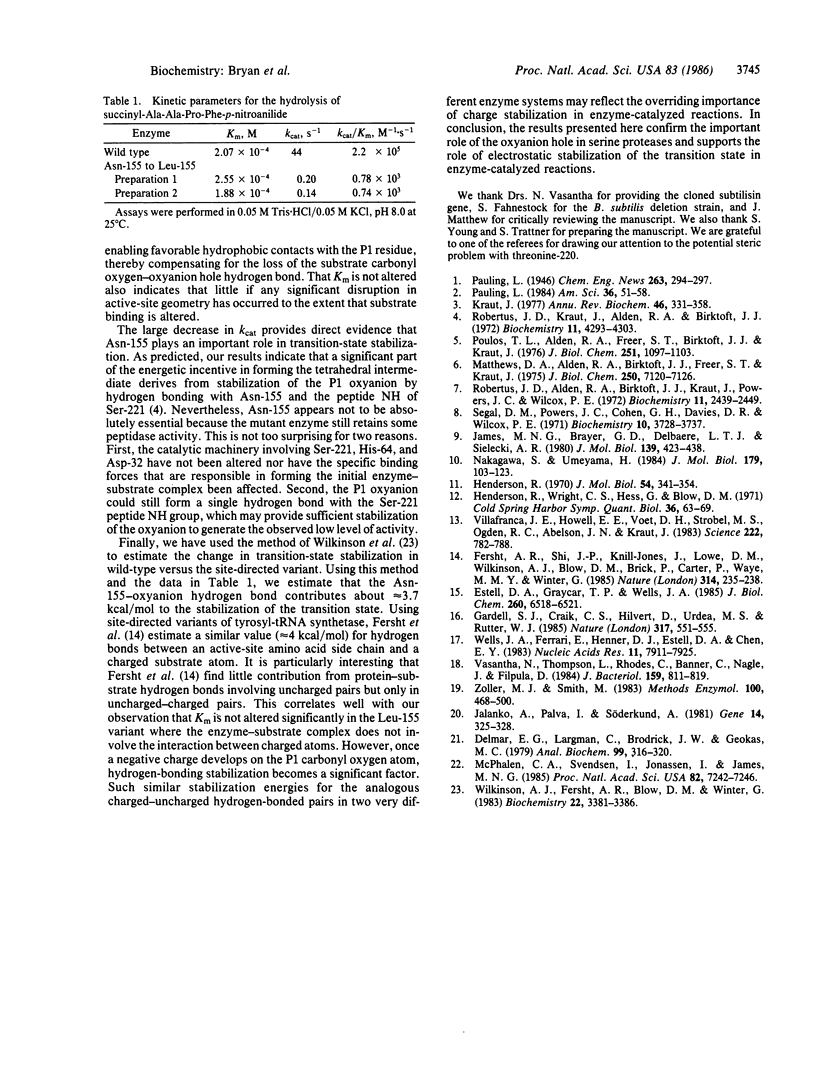
Images in this article
Selected References
These references are in PubMed. This may not be the complete list of references from this article.
- DelMar E. G., Largman C., Brodrick J. W., Geokas M. C. A sensitive new substrate for chymotrypsin. Anal Biochem. 1979 Nov 1;99(2):316–320. doi: 10.1016/s0003-2697(79)80013-5. [DOI] [PubMed] [Google Scholar]
- Estell D. A., Graycar T. P., Wells J. A. Engineering an enzyme by site-directed mutagenesis to be resistant to chemical oxidation. J Biol Chem. 1985 Jun 10;260(11):6518–6521. [PubMed] [Google Scholar]
- Fersht A. R., Shi J. P., Knill-Jones J., Lowe D. M., Wilkinson A. J., Blow D. M., Brick P., Carter P., Waye M. M., Winter G. Hydrogen bonding and biological specificity analysed by protein engineering. Nature. 1985 Mar 21;314(6008):235–238. doi: 10.1038/314235a0. [DOI] [PubMed] [Google Scholar]
- Gardell S. J., Craik C. S., Hilvert D., Urdea M. S., Rutter W. J. Site-directed mutagenesis shows that tyrosine 248 of carboxypeptidase A does not play a crucial role in catalysis. Nature. 1985 Oct 10;317(6037):551–555. doi: 10.1038/317551a0. [DOI] [PubMed] [Google Scholar]
- Henderson R. Structure of crystalline alpha-chymotrypsin. IV. The structure of indoleacryloyl-alpha-chyotrypsin and its relevance to the hydrolytic mechanism of the enzyme. J Mol Biol. 1970 Dec 14;54(2):341–354. doi: 10.1016/0022-2836(70)90434-1. [DOI] [PubMed] [Google Scholar]
- Henderson R., Wright C. S., Hess G. P., Blow D. M. -Chymotrypsin: what can we learn about catalysis from x-ray diffraction? Cold Spring Harb Symp Quant Biol. 1972;36:63–70. doi: 10.1101/sqb.1972.036.01.011. [DOI] [PubMed] [Google Scholar]
- Jalanko A., Palva I., Söderlund Restriction maps of plasmids pUB110 and pBD9. Gene. 1981 Sep;14(4):325–328. doi: 10.1016/0378-1119(81)90165-7. [DOI] [PubMed] [Google Scholar]
- James M. N., Brayer G. D., Delbaere L. T., Sielecki A. R., Gertler A. Crystal structure studies and inhibition kinetics of tripeptide chloromethyl ketone inhibitors with Streptomyces griseus protease B. J Mol Biol. 1980 May 25;139(3):423–438. doi: 10.1016/0022-2836(80)90139-4. [DOI] [PubMed] [Google Scholar]
- Kraut J. Serine proteases: structure and mechanism of catalysis. Annu Rev Biochem. 1977;46:331–358. doi: 10.1146/annurev.bi.46.070177.001555. [DOI] [PubMed] [Google Scholar]
- Matthews D. A., Alden R. A., Birktoft J. J., Freer S. T., Kraut J. X-ray crystallographic study of boronic acid adducts with subtilisin BPN' (Novo). A model for the catalytic transition state. J Biol Chem. 1975 Sep 25;250(18):7120–7126. [PubMed] [Google Scholar]
- McPhalen C. A., Svendsen I., Jonassen I., James M. N. Crystal and molecular structure of chymotrypsin inhibitor 2 from barley seeds in complex with subtilisin Novo. Proc Natl Acad Sci U S A. 1985 Nov;82(21):7242–7246. doi: 10.1073/pnas.82.21.7242. [DOI] [PMC free article] [PubMed] [Google Scholar]
- Nakagawa S., Umeyama H. Role of catalytic residues in the formation of a tetrahedral adduct in the acylation reaction of bovine beta-trypsin. A molecular orbital study. J Mol Biol. 1984 Oct 15;179(1):103–123. doi: 10.1016/0022-2836(84)90308-5. [DOI] [PubMed] [Google Scholar]
- Poulos T. L., Alden R. A., Freer S. T., Birktoft J. J., Kraut J. Polypeptide halomethyl ketones bind to serine proteases as analogs of the tetrahedral intermediate. X-ray crystallographic comparison of lysine- and phenylalanine-polypeptide chloromethyl ketone-inhibited subtilisin. J Biol Chem. 1976 Feb 25;251(4):1097–1103. [PubMed] [Google Scholar]
- Robertus J. D., Alden R. A., Birktoft J. J., Kraut J., Powers J. C., Wilcox P. E. An x-ray crystallographic study of the binding of peptide chloromethyl ketone inhibitors to subtilisin BPN'. Biochemistry. 1972 Jun 20;11(13):2439–2449. doi: 10.1021/bi00763a009. [DOI] [PubMed] [Google Scholar]
- Robertus J. D., Kraut J., Alden R. A., Birktoft J. J. Subtilisin; a stereochemical mechanism involving transition-state stabilization. Biochemistry. 1972 Nov 7;11(23):4293–4303. doi: 10.1021/bi00773a016. [DOI] [PubMed] [Google Scholar]
- Segal D. M., Powers J. C., Cohen G. H., Davies D. R., Wilcox P. E. Substrate binding site in bovine chymotrypsin A-gamma. A crystallographic study using peptide chloromethyl ketones as site-specific inhibitors. Biochemistry. 1971 Sep 28;10(20):3728–3738. doi: 10.1021/bi00796a014. [DOI] [PubMed] [Google Scholar]
- Vasantha N., Thompson L. D., Rhodes C., Banner C., Nagle J., Filpula D. Genes for alkaline protease and neutral protease from Bacillus amyloliquefaciens contain a large open reading frame between the regions coding for signal sequence and mature protein. J Bacteriol. 1984 Sep;159(3):811–819. doi: 10.1128/jb.159.3.811-819.1984. [DOI] [PMC free article] [PubMed] [Google Scholar]
- Villafranca J. E., Howell E. E., Voet D. H., Strobel M. S., Ogden R. C., Abelson J. N., Kraut J. Directed mutagenesis of dihydrofolate reductase. Science. 1983 Nov 18;222(4625):782–788. doi: 10.1126/science.6356360. [DOI] [PubMed] [Google Scholar]
- Wells J. A., Ferrari E., Henner D. J., Estell D. A., Chen E. Y. Cloning, sequencing, and secretion of Bacillus amyloliquefaciens subtilisin in Bacillus subtilis. Nucleic Acids Res. 1983 Nov 25;11(22):7911–7925. doi: 10.1093/nar/11.22.7911. [DOI] [PMC free article] [PubMed] [Google Scholar]
- Zoller M. J., Smith M. Oligonucleotide-directed mutagenesis of DNA fragments cloned into M13 vectors. Methods Enzymol. 1983;100:468–500. doi: 10.1016/0076-6879(83)00074-9. [DOI] [PubMed] [Google Scholar]