Abstract
Escherichia coli C6 rel met cys was cultured in a stringently defined minimal medium containing 13C-enriched metabolites in order to (1) achieve maximal 13C isotopic enrichment of tRNA; and (2) produce site specific but natural, non-perturbing NMR probes of tRNA structure and function. Growth conditions were manipulated to achieve optimal culture growth concomitant with maximal in vivo incorporation of various 13C-enriched nucleic acid precursors, including L-[methyl-13C] methionine, [2-(13)C] adenine, and [2-(13)C] uracil. Effective blockage of purine biosynthesis de novo was accomplished with the addition of the antimetabolite 6-mercaptopurine to the growth medium. Transfer RNAs specifically 13C-enriched in all methyl groups (57 atom %), C2 of adenine (60 atom %), and C2 of uracil (82 atom %) and C2 of cytosine (73 atom %) have been produced.
Full text
PDF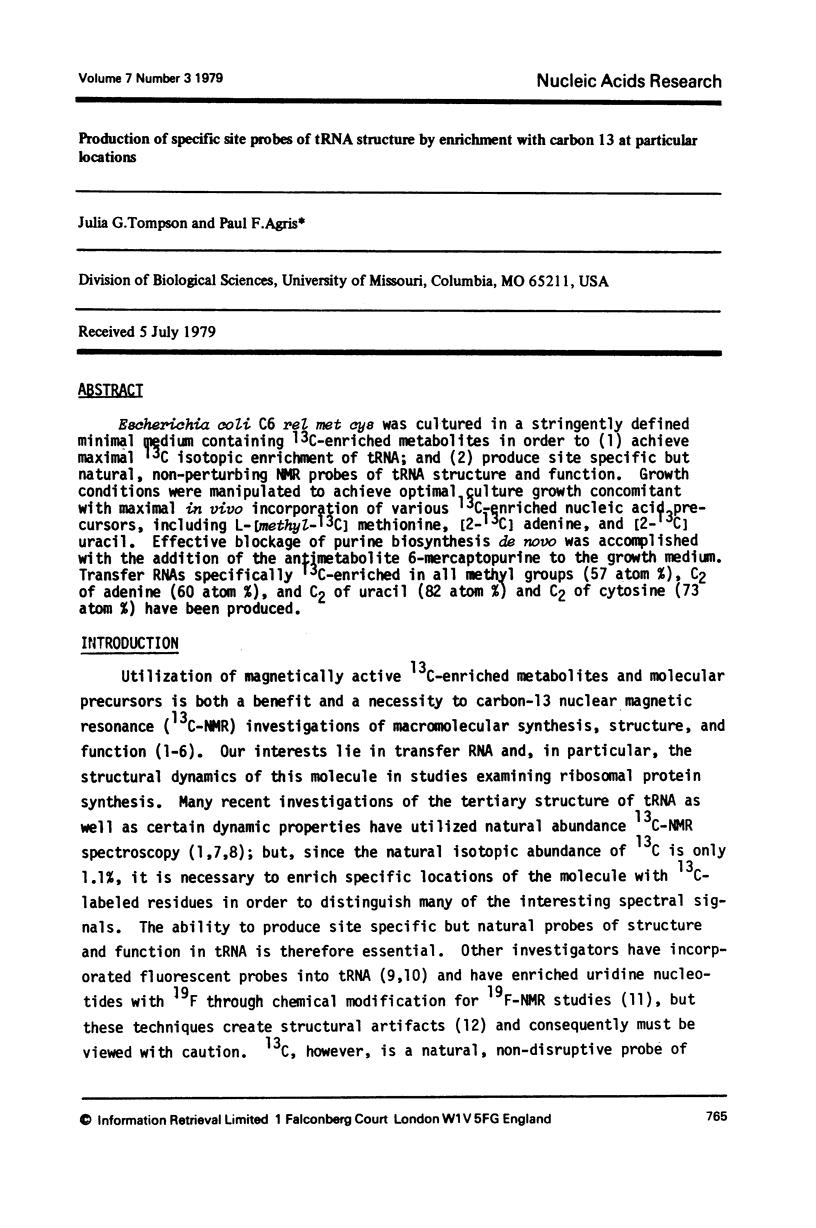
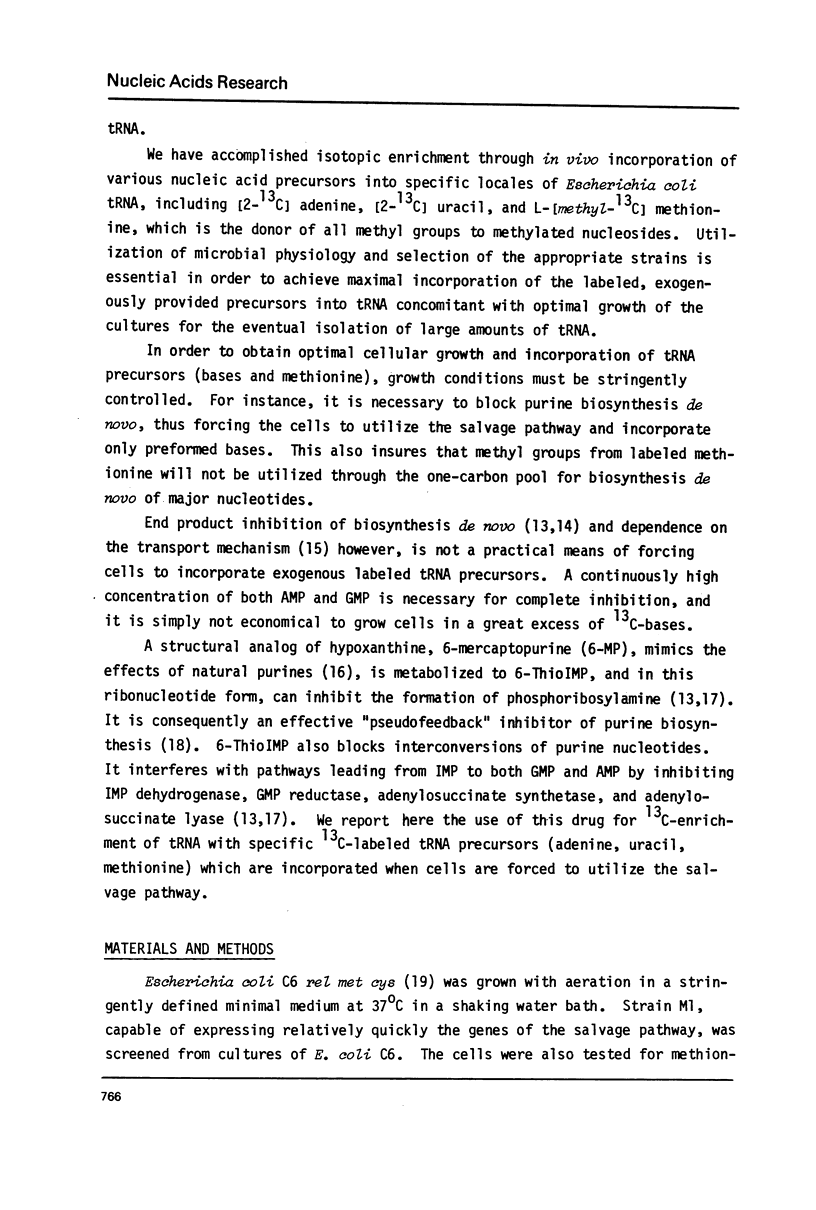
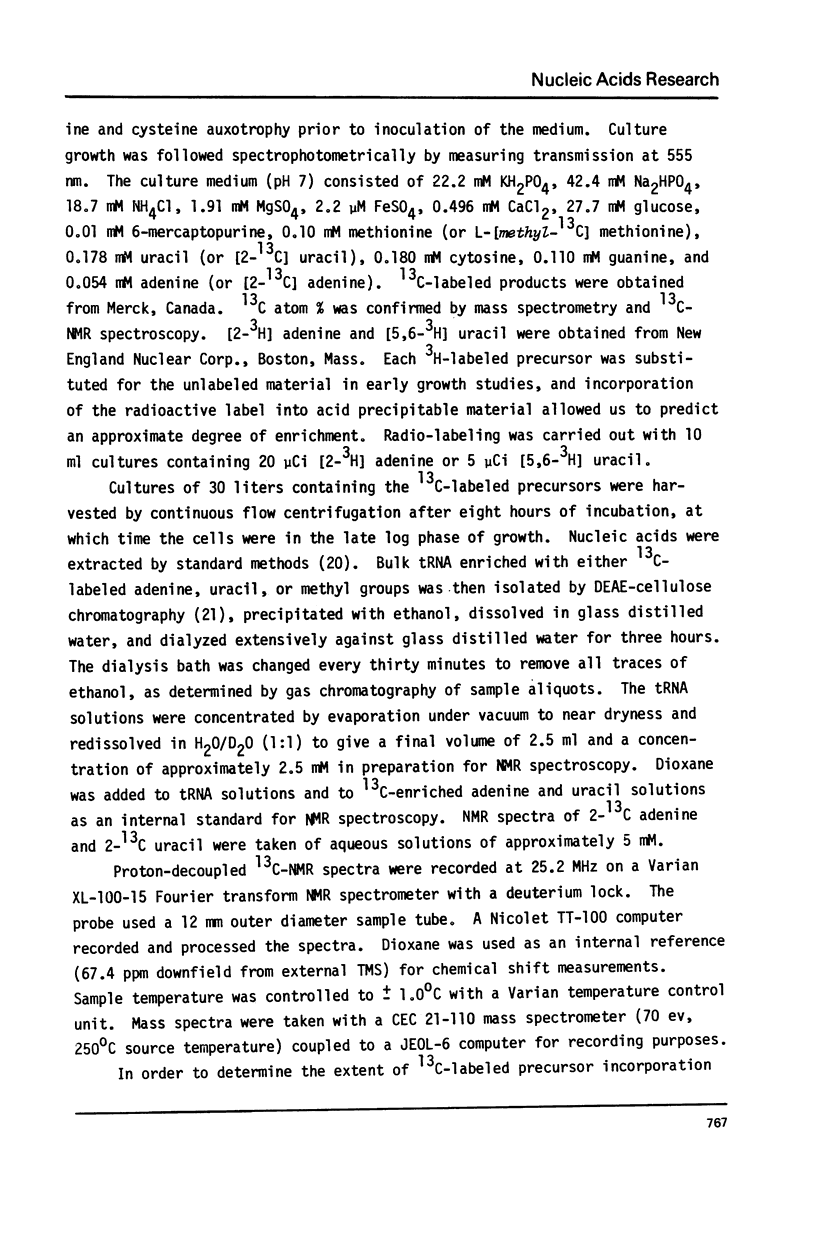
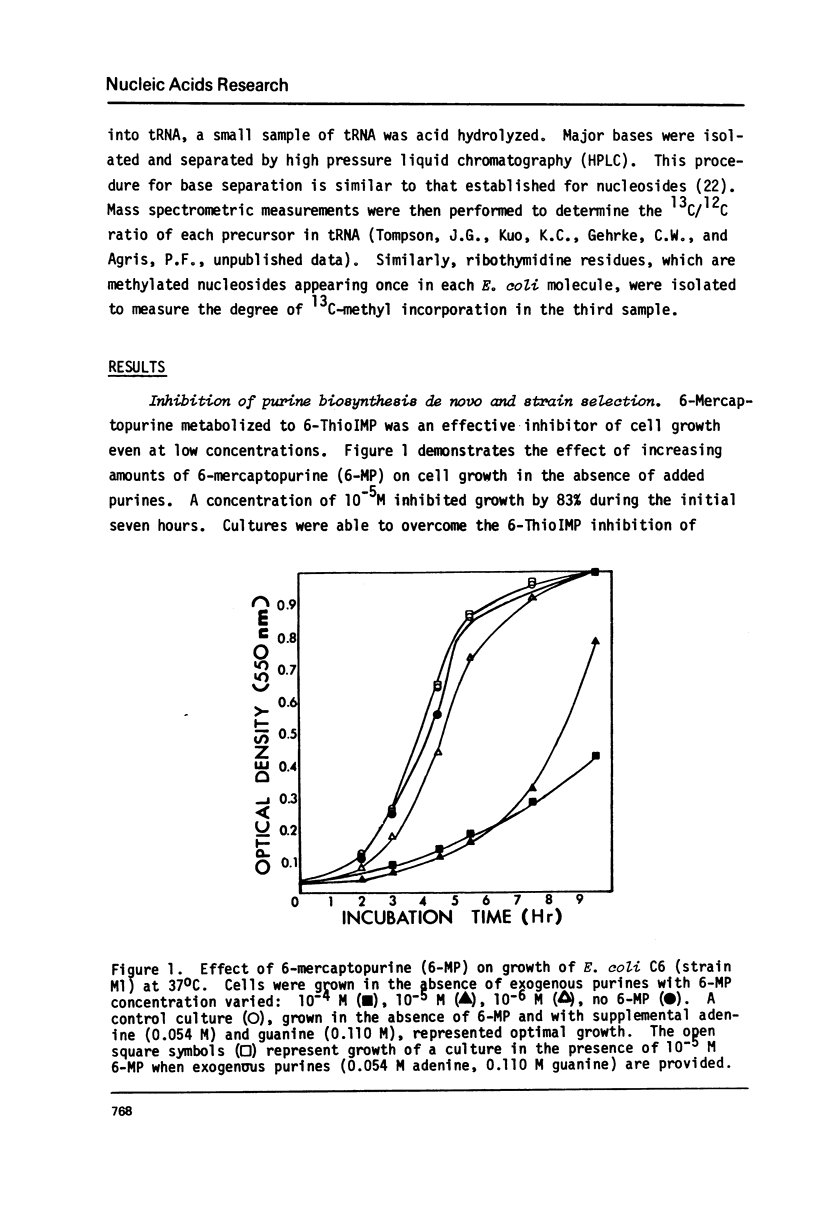
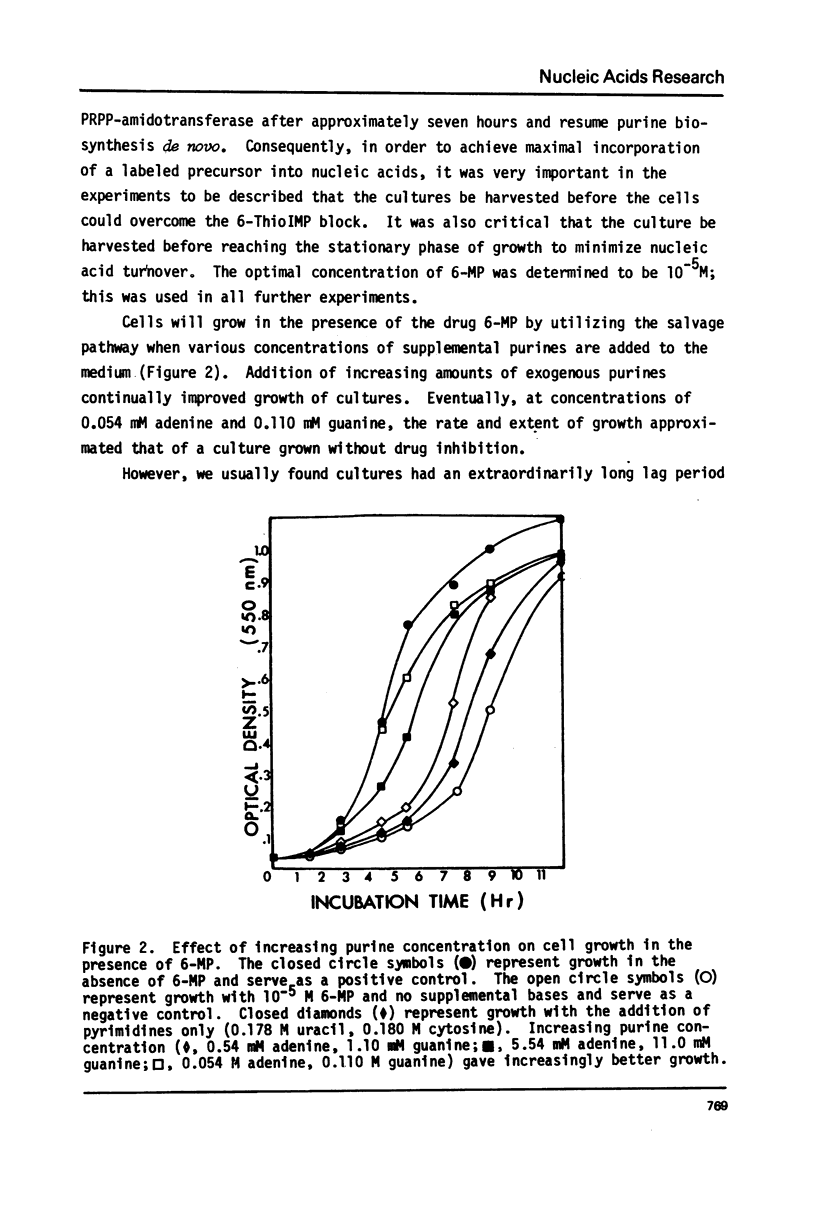
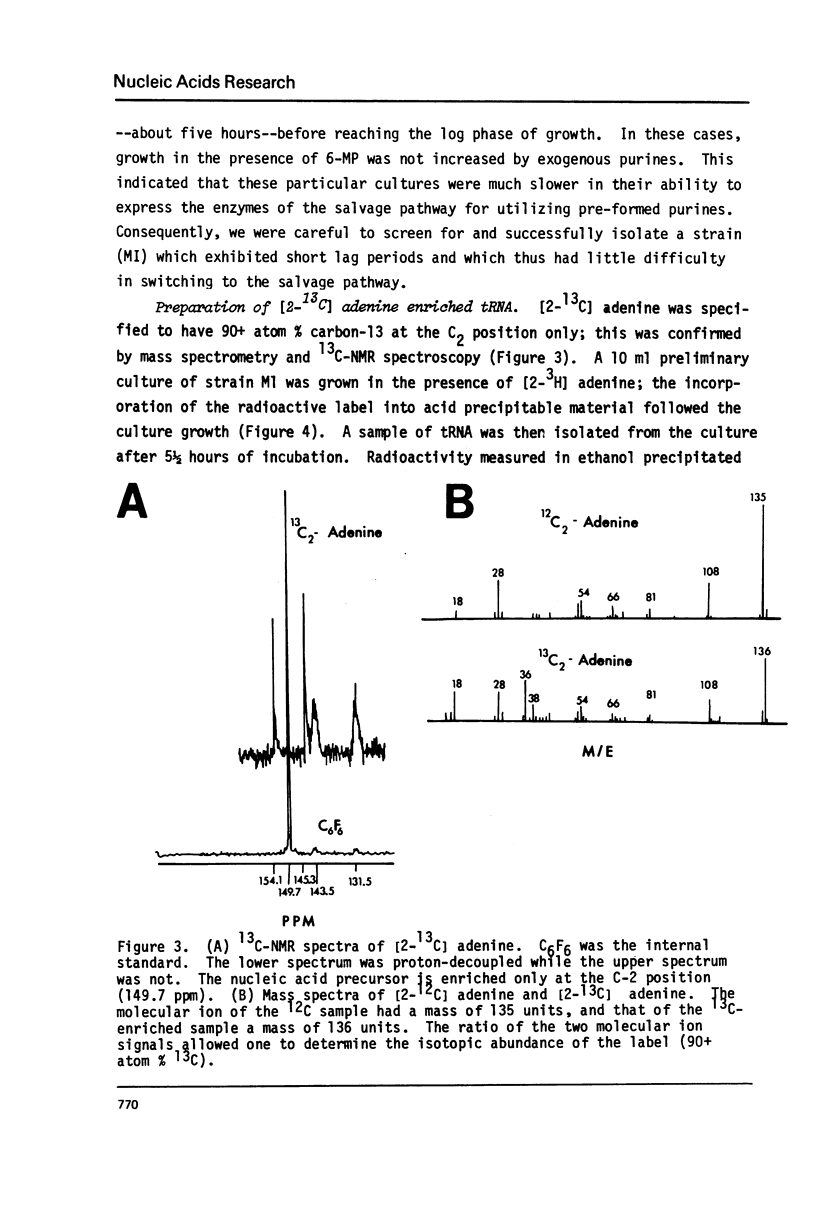
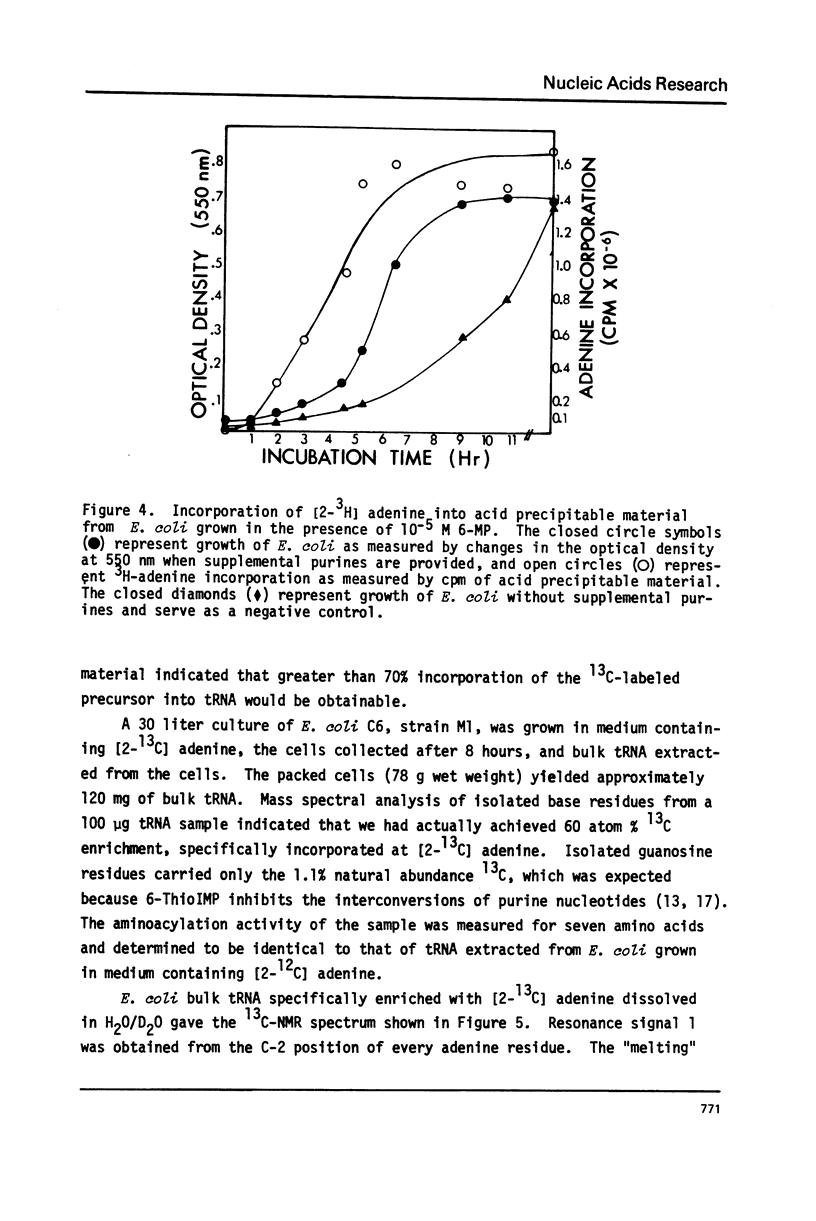
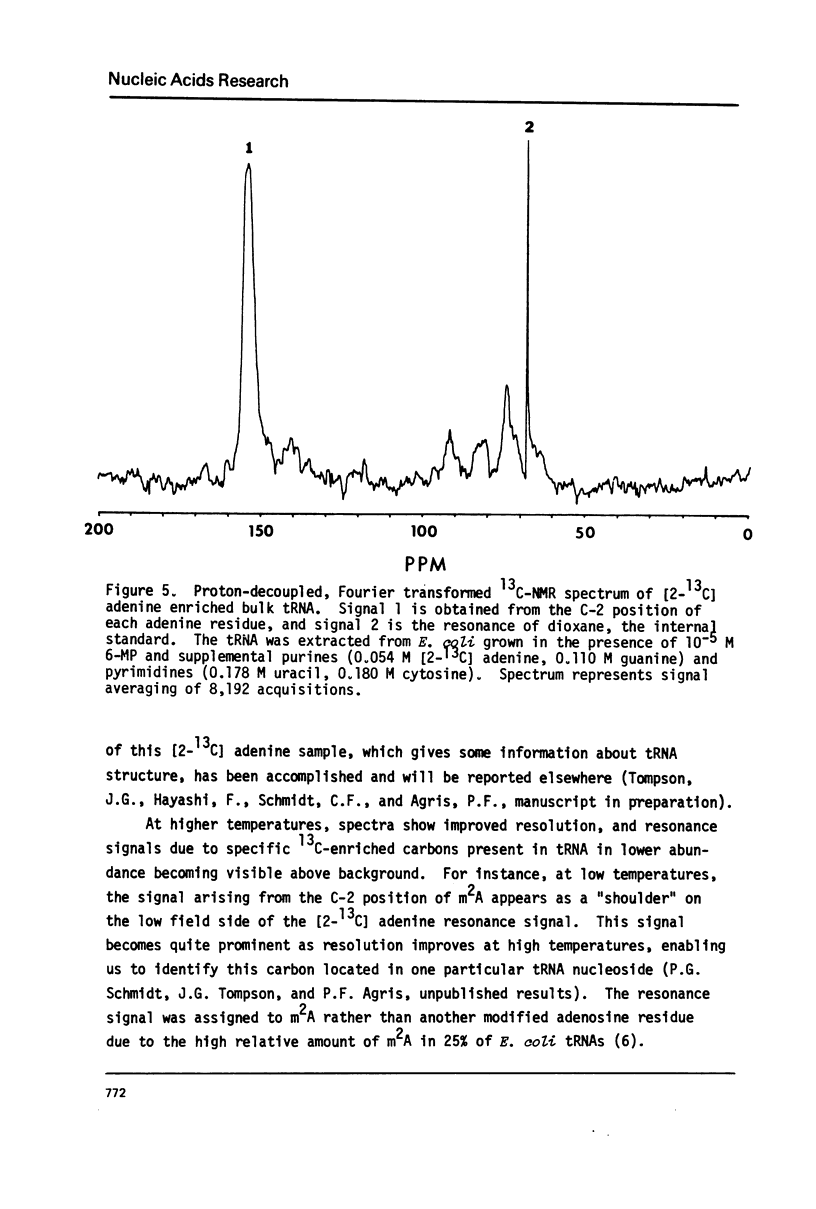
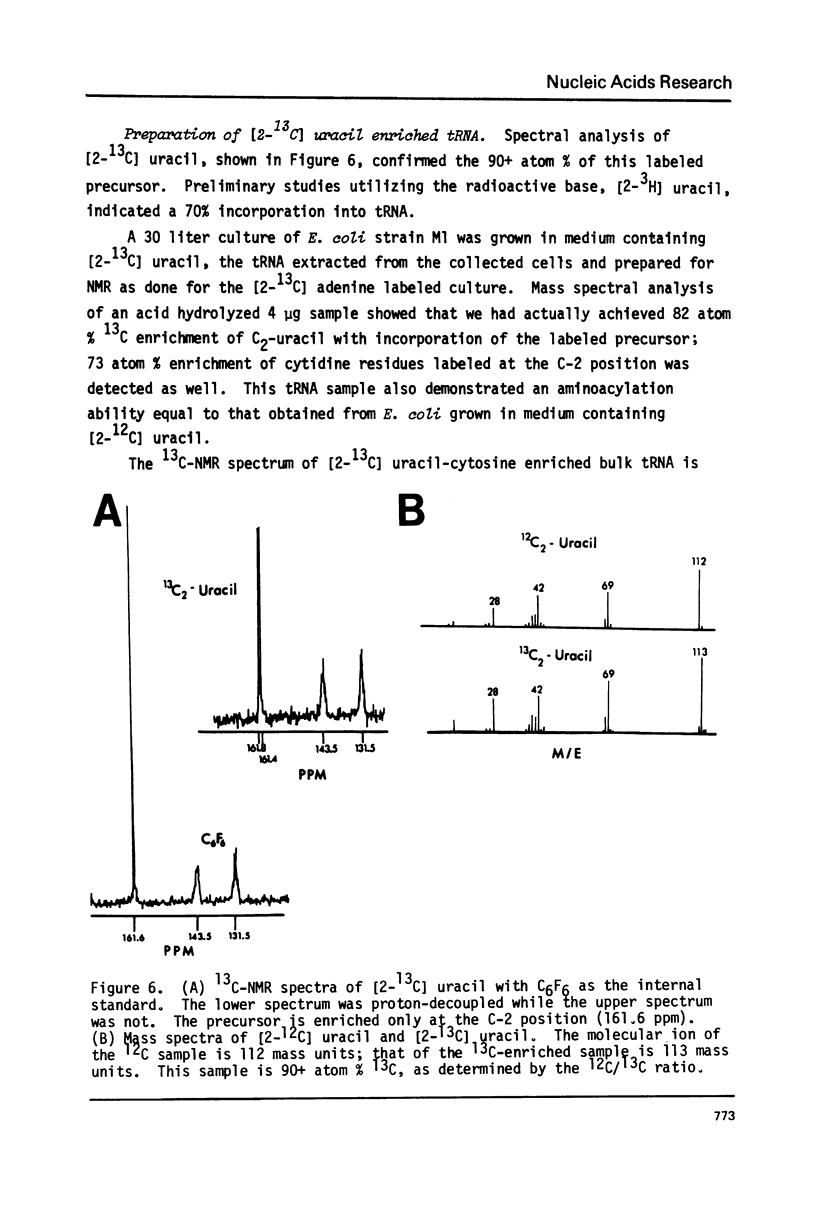
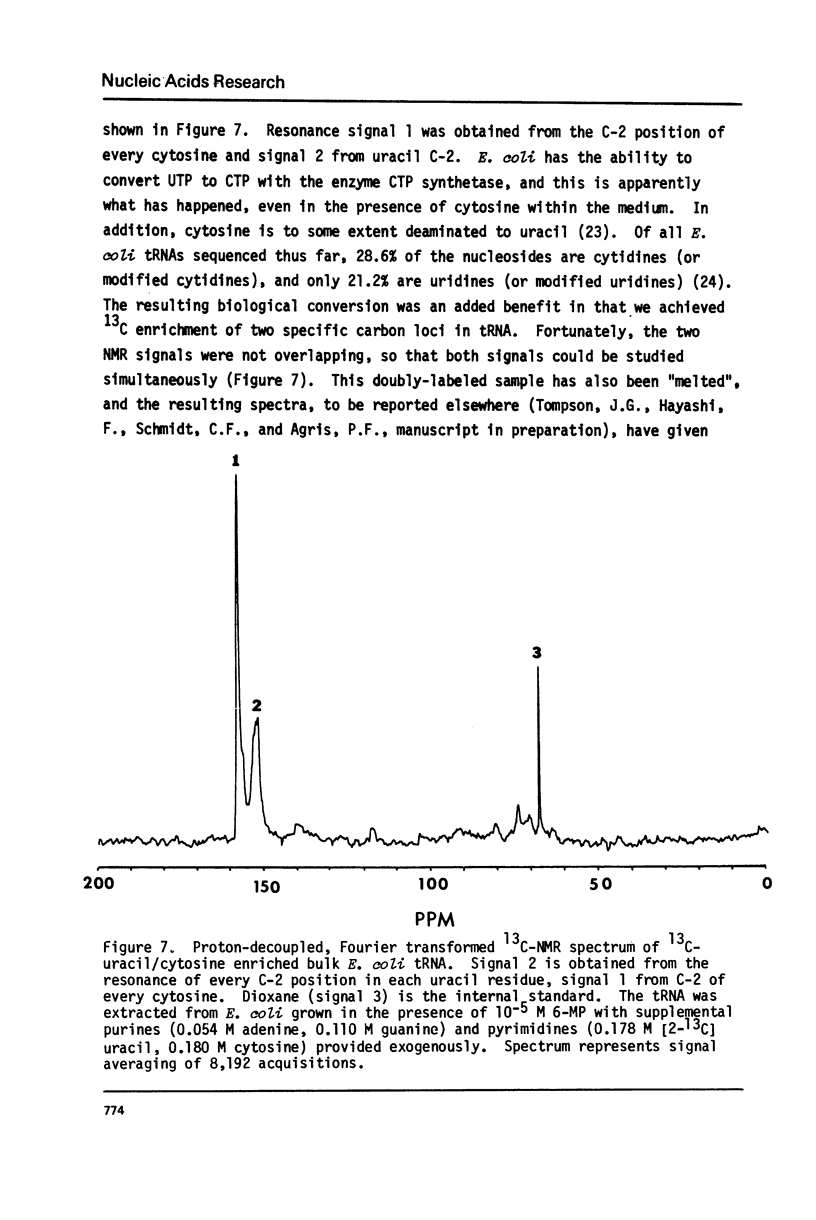
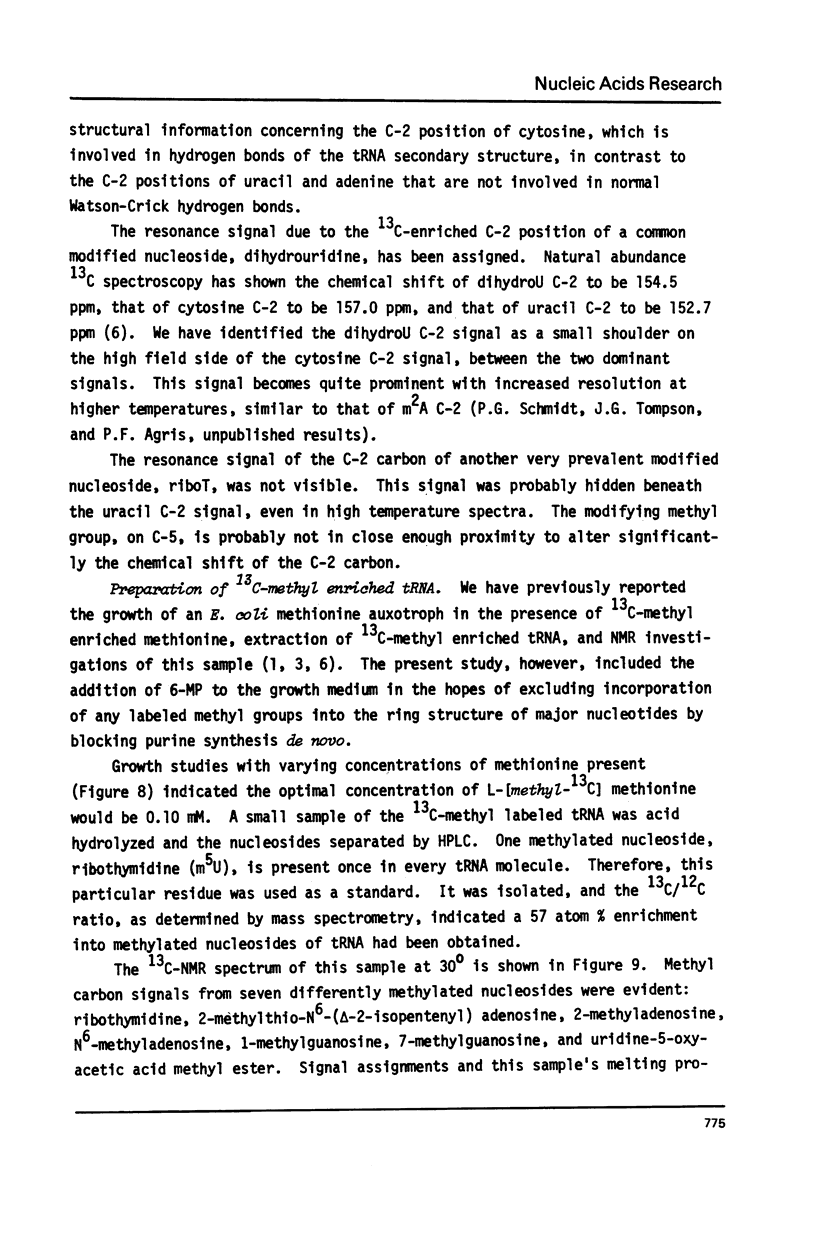
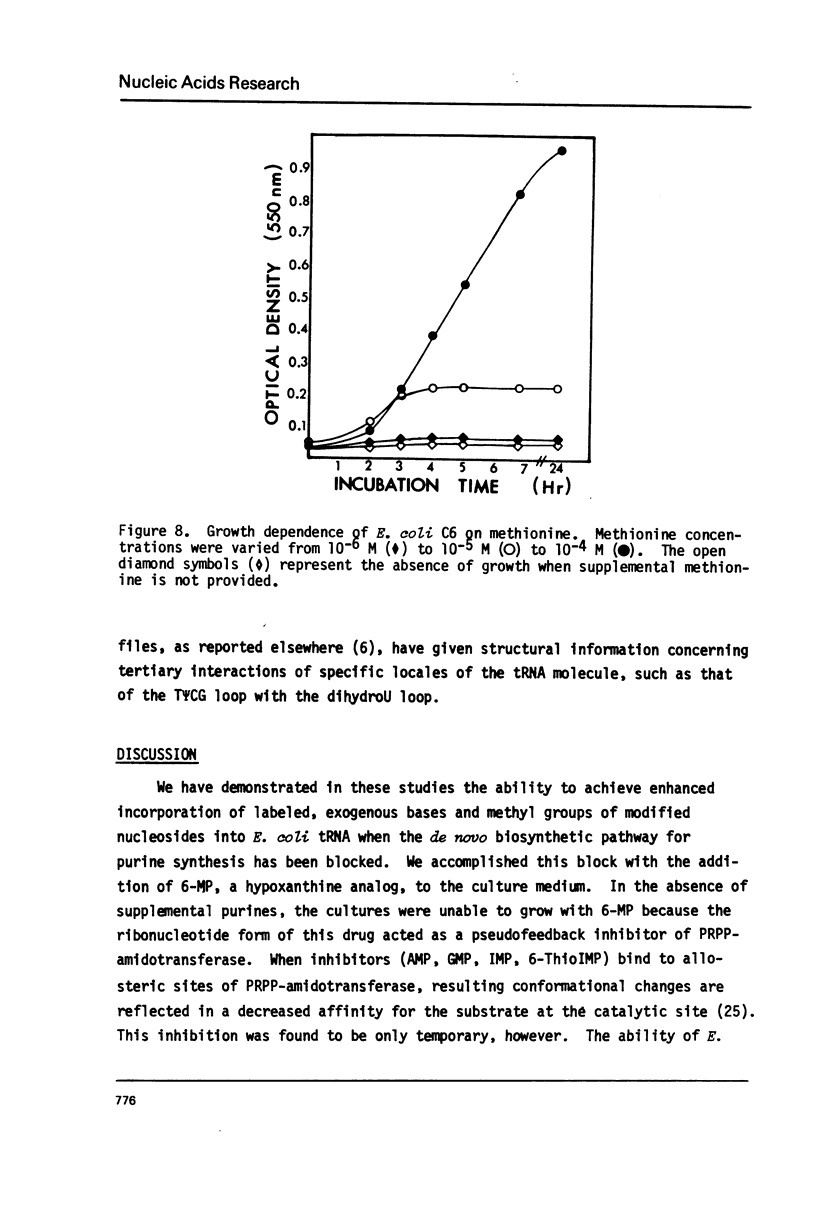
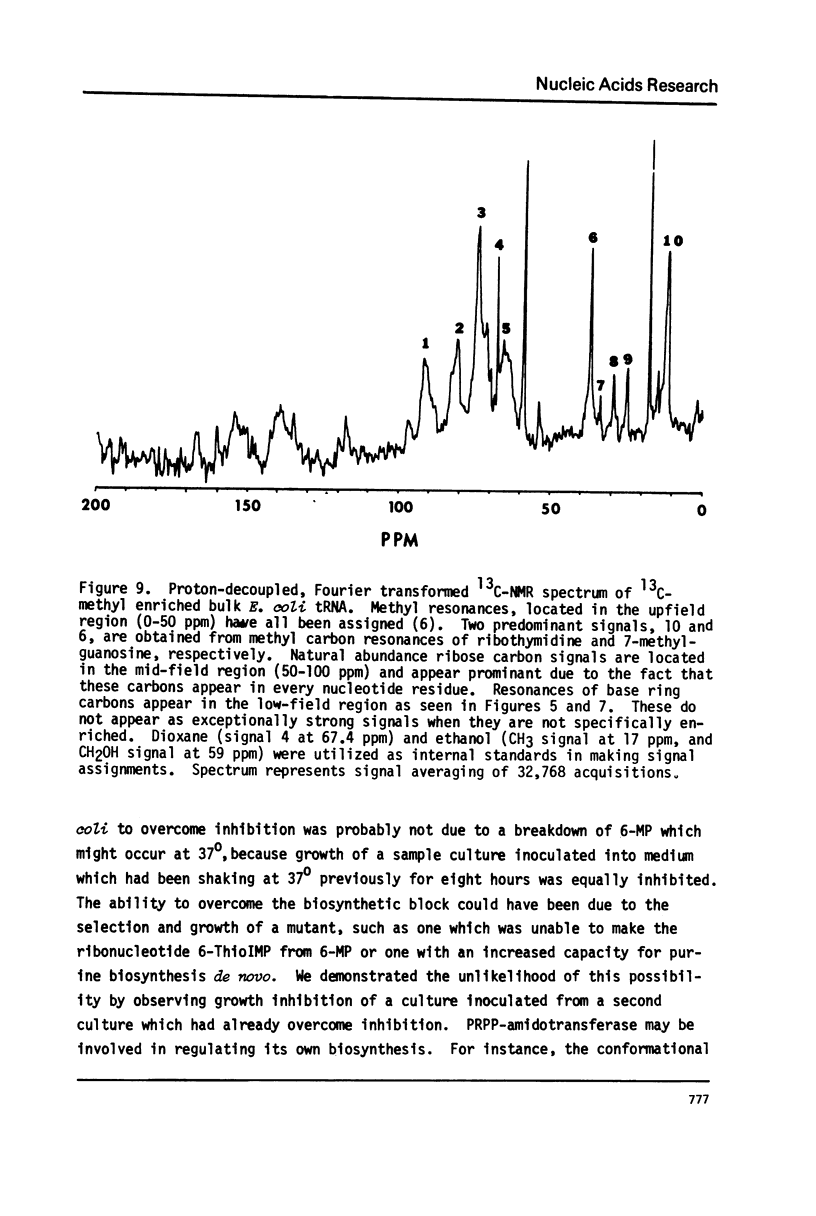
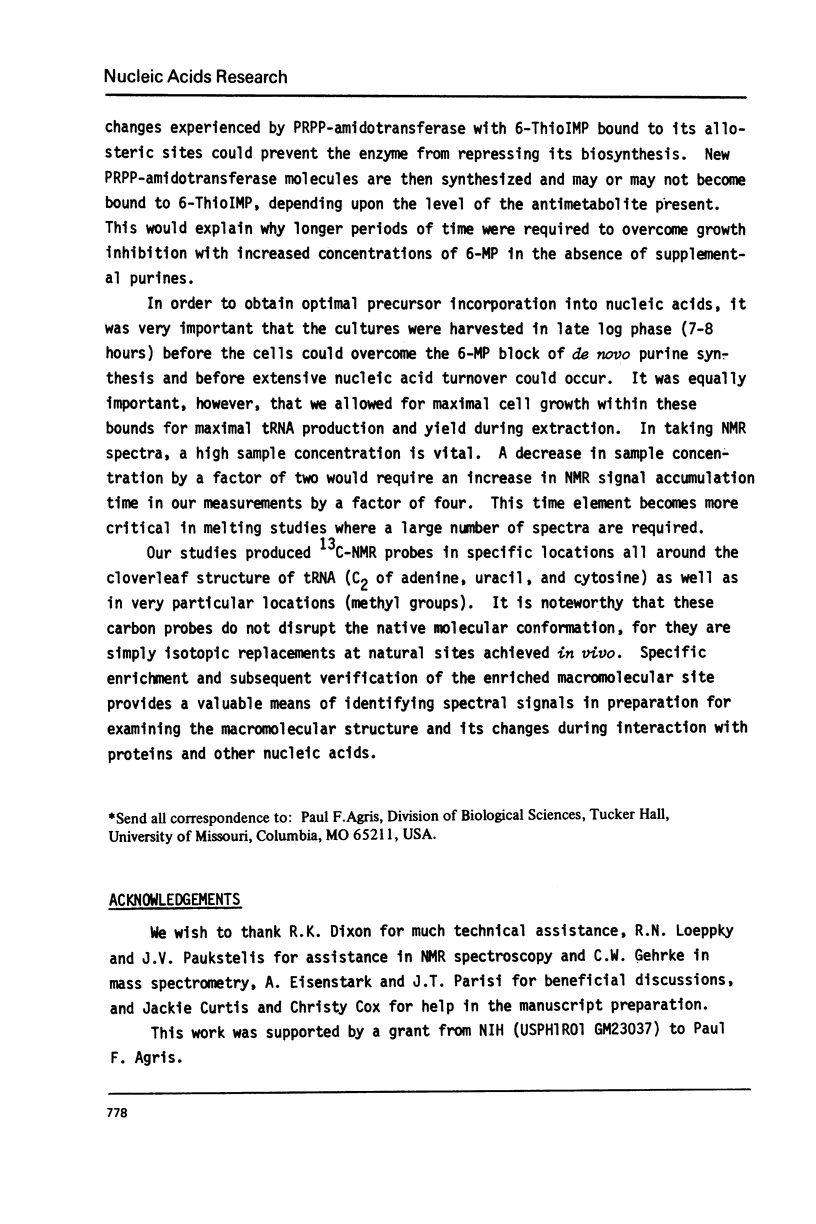
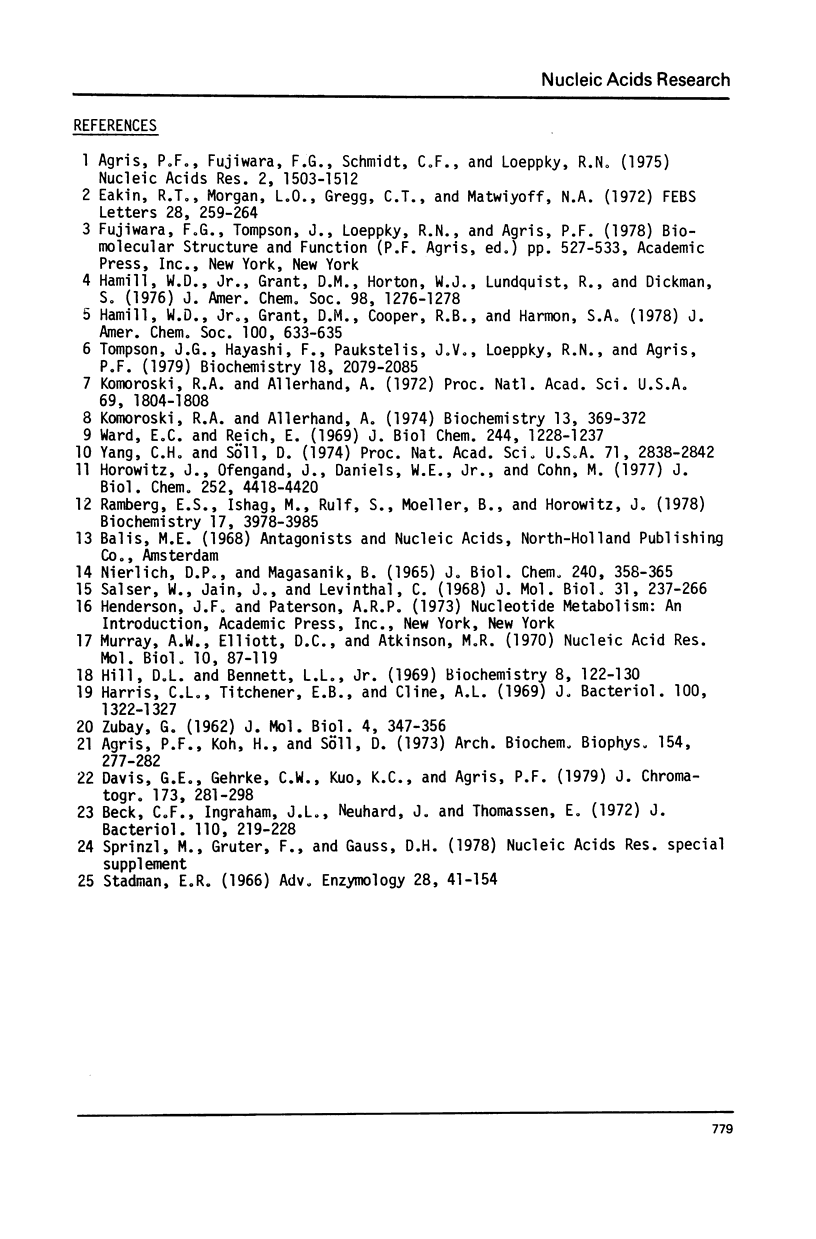
Selected References
These references are in PubMed. This may not be the complete list of references from this article.
- Agris P. F., Fujiwara F. G., Schmidt C. F., Loeppky R. N. Utilization of an Escherichia coli mutant for carbon-13 enrichment of tRNA for NMR studies. Nucleic Acids Res. 1975 Sep;2(9):1503–1512. doi: 10.1093/nar/2.9.1503. [DOI] [PMC free article] [PubMed] [Google Scholar]
- Agris P. F., Koh H., Söll D. The effect of growth temperatures on the in vivo ribose methylation of Bacillus stearothermophilus transfer RNA. Arch Biochem Biophys. 1973 Jan;154(1):277–282. doi: 10.1016/0003-9861(73)90058-1. [DOI] [PubMed] [Google Scholar]
- Beck C. F., Ingraham J. L., Neuhard J., Thomassen E. Metabolism of pyrimidines and pyrimidine nucleosides by Salmonella typhimurium. J Bacteriol. 1972 Apr;110(1):219–228. doi: 10.1128/jb.110.1.219-228.1972. [DOI] [PMC free article] [PubMed] [Google Scholar]
- Davis G. E., Gehrke C. W., Kuo K. C., Agris P. F. Major and modified nucleosides in tRNA hydrolysates by high-performance liquid chromatography. J Chromatogr. 1979 May 21;173(2):281–298. doi: 10.1016/s0021-9673(00)92297-0. [DOI] [PubMed] [Google Scholar]
- Eakin R. T., Morgan L. O., Gregg C. T., Matwiyoff N. A. Carbon-13 nuclear magnetic resonance spectroscopy of living cells and their metabolism of a specifically labeled 13C substrate. FEBS Lett. 1972 Dec 15;28(3):259–264. doi: 10.1016/0014-5793(72)80726-9. [DOI] [PubMed] [Google Scholar]
- Hamill W. D., Jr, Grant D. M., Horton W. J., Lundquist R., Dickman S. Letter: Magnetic resonance spectroscopy on carbon-13 labeled uracil in transfer ribonucleic acid. J Am Chem Soc. 1976 Mar 3;98(5):1276–1273. doi: 10.1021/ja00421a047. [DOI] [PubMed] [Google Scholar]
- Harris C. L., Titchener E. B., Cline A. L. Sulfur-deficient transfer ribonucleic acid in a cysteine-requiring, "relaxed" mutant of Escherichia coli. J Bacteriol. 1969 Dec;100(3):1322–1327. doi: 10.1128/jb.100.3.1322-1327.1969. [DOI] [PMC free article] [PubMed] [Google Scholar]
- Hill D. L., Bennett L. L., Jr Purification and properties of 5-phosphoribosyl pyrophosphate amidotransferase from adenocarcinoma 755 cells. Biochemistry. 1969 Jan;8(1):122–130. doi: 10.1021/bi00829a017. [DOI] [PubMed] [Google Scholar]
- Horowitz J., Ofengand J., Daniel W. E., Jr, Cohn M. 19F nuclear magnetic resonance of 5-fluorouridine-substituted tRNA1Val from Escherichia coli. J Biol Chem. 1977 Jun 25;252(12):4418–4420. [PubMed] [Google Scholar]
- Komoroski R. A., Allerhand A. Natural-abundance carbon-13 Fourier-transform nuclear magnetic resonance spectra and spin lattice relaxation times of unfractionated yeast transfer-FNA. Proc Natl Acad Sci U S A. 1972 Jul;69(7):1804–1808. doi: 10.1073/pnas.69.7.1804. [DOI] [PMC free article] [PubMed] [Google Scholar]
- Komoroski R. A., Allerhand A. Observation of resonances from some minor bases in the natural-abundance carbon-13 nuclear magnetic resonance spectrum of unfractionated yeast transfer ribonucleic acid. Evidence for fast internal motion of the dihydrouracil rings. Biochemistry. 1974 Jan 15;13(2):369–372. doi: 10.1021/bi00699a023. [DOI] [PubMed] [Google Scholar]
- Murray A. W., Elliott D. C., Atkinson M. R. Nucleotide biosynthesis from preformed purines in mammalian cells: regulatory mechanisms and biological significance. Prog Nucleic Acid Res Mol Biol. 1970;10:87–119. doi: 10.1016/s0079-6603(08)60562-0. [DOI] [PubMed] [Google Scholar]
- NIERLICH D. P., MAGASANIK B. REGULATION OF PURINE RIBONUCLEOTIDE SYNTHESIS BY END PRODUCT INHIBITION. THE EFFECT OF ADENINE AND GUANINE RIBONUCLEOTIDES ON THE 5'-PHOSPHORIBOSYL-PYROPHOSPHATE AMIDOTRANSFERASE OF AEROBACTER AEROGENES. J Biol Chem. 1965 Jan;240:358–365. [PubMed] [Google Scholar]
- Ramberg E. S., Ishaq M., Rulf S., Moeller B., Horowitz J. Inhibition of transfer RNA function by replacement of uridine and uridine-derived nucleosides with 5-fluorouridine. Biochemistry. 1978 Sep 19;17(19):3978–3985. doi: 10.1021/bi00612a016. [DOI] [PubMed] [Google Scholar]
- Salser W., Janin J., Levinthal C. Measurement of the unstable RNA in exponentially growing cultures of Bacillus subtilis and Escherichia coli. J Mol Biol. 1968 Jan 28;31(2):237–266. doi: 10.1016/0022-2836(68)90442-7. [DOI] [PubMed] [Google Scholar]
- Tompson J. G., Hayashi F., Paukstelis J. V., Loeppky R. N., Agris P. F. Complete nuclear magnetic resonance signal assignments and initial structural studies of [13C]methyl-enriched transfer ribonucleic acid. Biochemistry. 1979 May 15;18(10):2079–2085. doi: 10.1021/bi00577a037. [DOI] [PubMed] [Google Scholar]
- Ward D. C., Reich E., Stryer L. Fluorescence studies of nucleotides and polynucleotides. I. Formycin, 2-aminopurine riboside, 2,6-diaminopurine riboside, and their derivatives. J Biol Chem. 1969 Mar 10;244(5):1228–1237. [PubMed] [Google Scholar]
- Yang C. H., Söll D. Studies of transfer RNA tertiary structure of singlet-singlet energy transfer. Proc Natl Acad Sci U S A. 1974 Jul;71(7):2838–2842. doi: 10.1073/pnas.71.7.2838. [DOI] [PMC free article] [PubMed] [Google Scholar]