Abstract
Proteins associated with intestinal brush borders and their various fractions were solubilized with sodium dodecyl sulfate and β-mercaptoethanol, and separated by electrophoresis on acrylamide gels containing sodium dodecyl sulfate. Brush borders contain at least 15 proteins or subunits, ranging in molecular weight from 19,000 to 270,000. The largest proteins (170-270,000 mol wt), including the disaccharidases, are removed from the brush borders by papain. Proteins belonging to the remaining membrane, including alkaline phosphatase, have an intermediate size (53-140,000 mol wt). The proteins corresponding to the filamentous “core” of the microvilli are the smallest (19-45,000).
The relative rates of degradation of these proteins were studied by following the rate of decline of 14C-labeled leucine activity in specific proteins, and by the double isotope technique of Schimke in which leucine-14C was given to intact rats intraluminally 10 hr before an intraluminal dose of leucine-3H. Heterogeneity of 3H/14C ratios and thus of rates of turnover of brush border proteins was noted. In general, the largest proteins (including the disaccharidases), were turning over the fastest. Other membrane proteins (i.e. alkaline phosphatase) had an intermediate rate of degradation, and “core” proteins turned over slowly. Thus, there was a general correlation between relative degradation rate and size.
Full text
PDF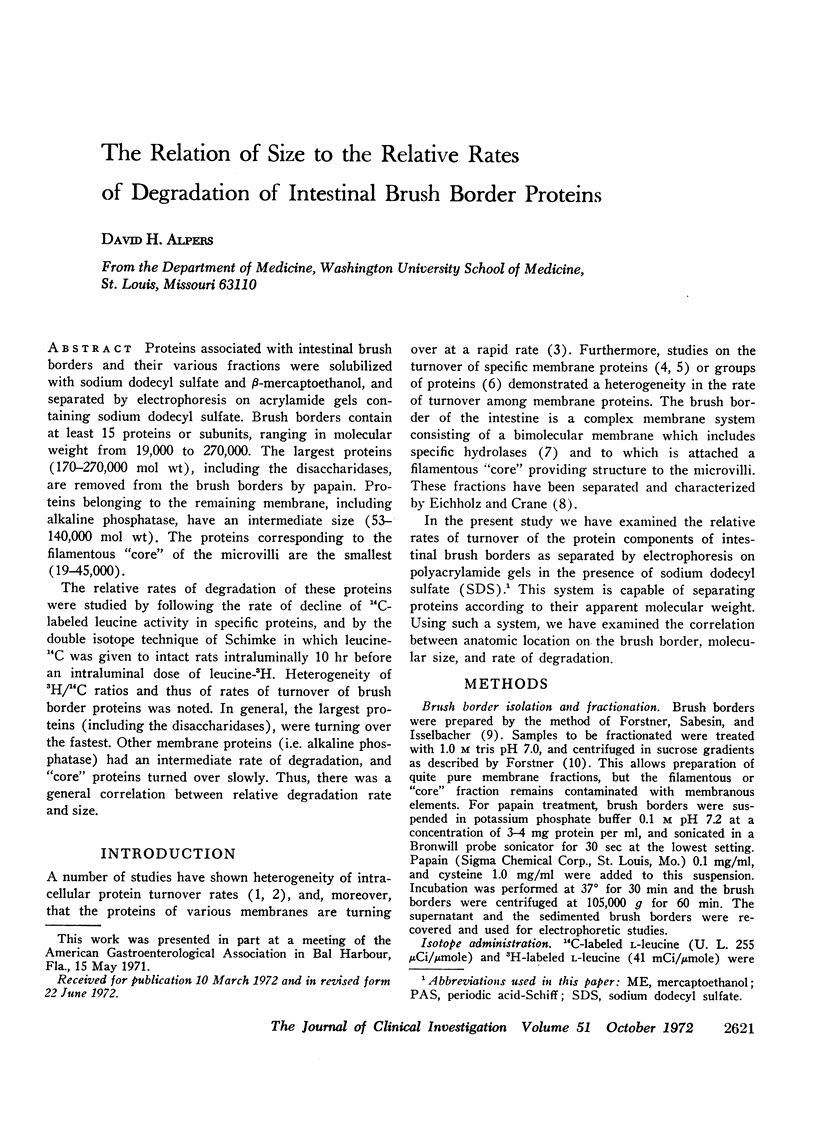
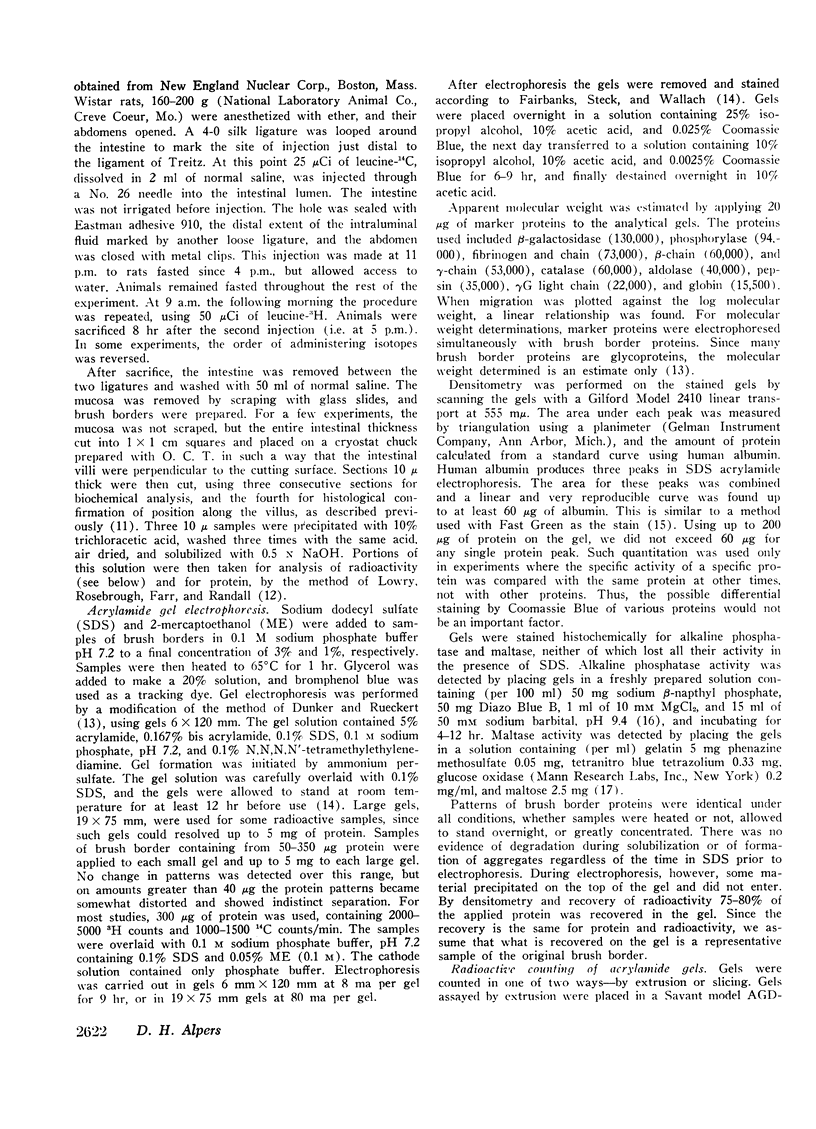
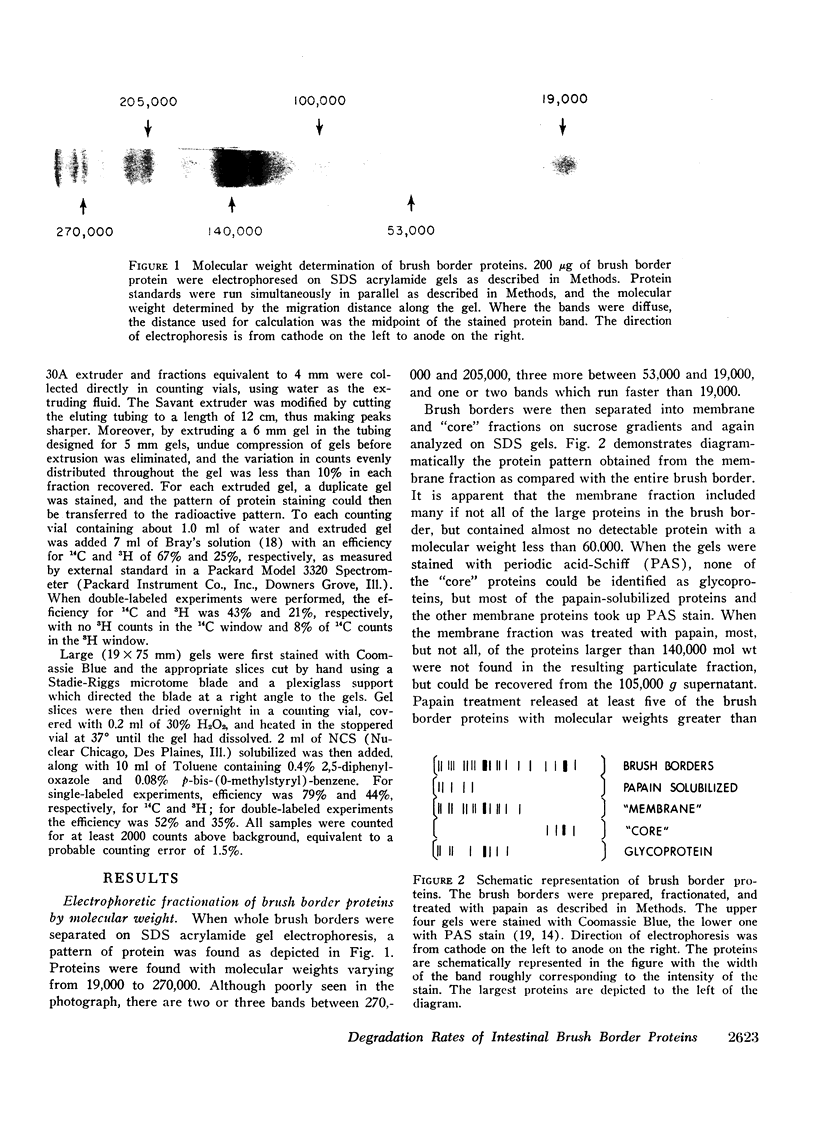
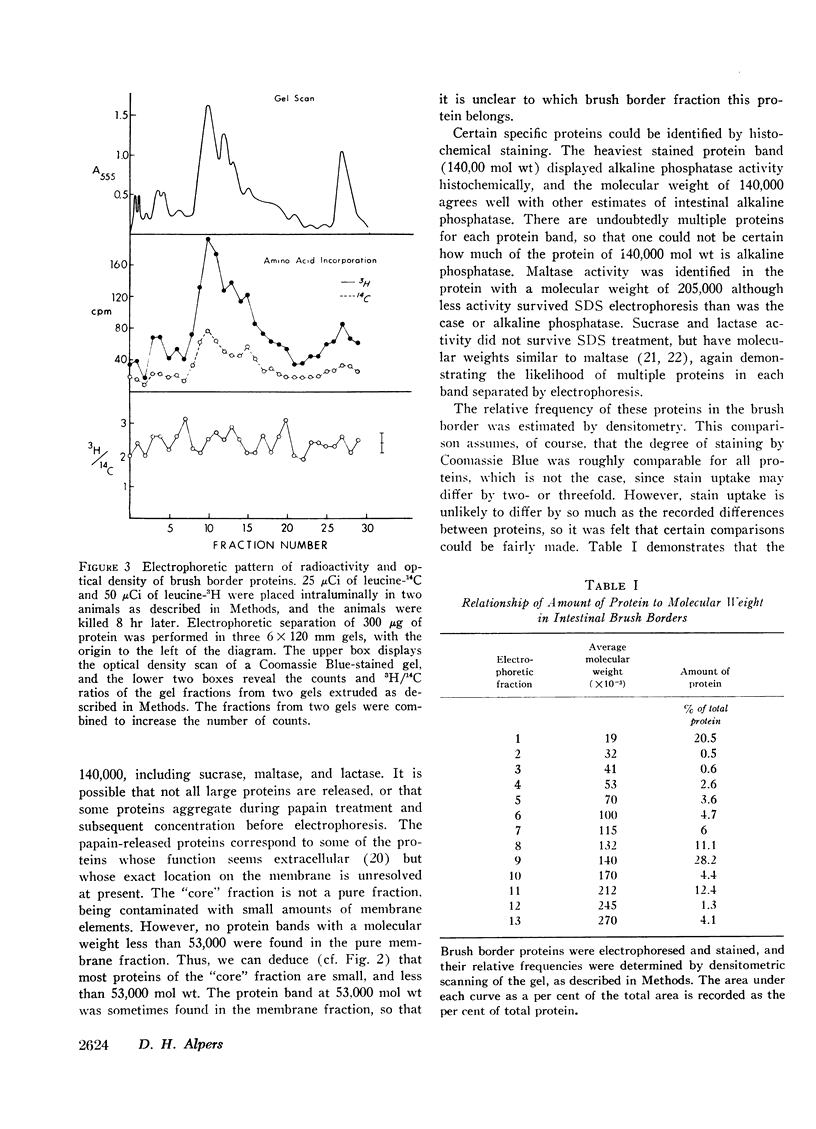
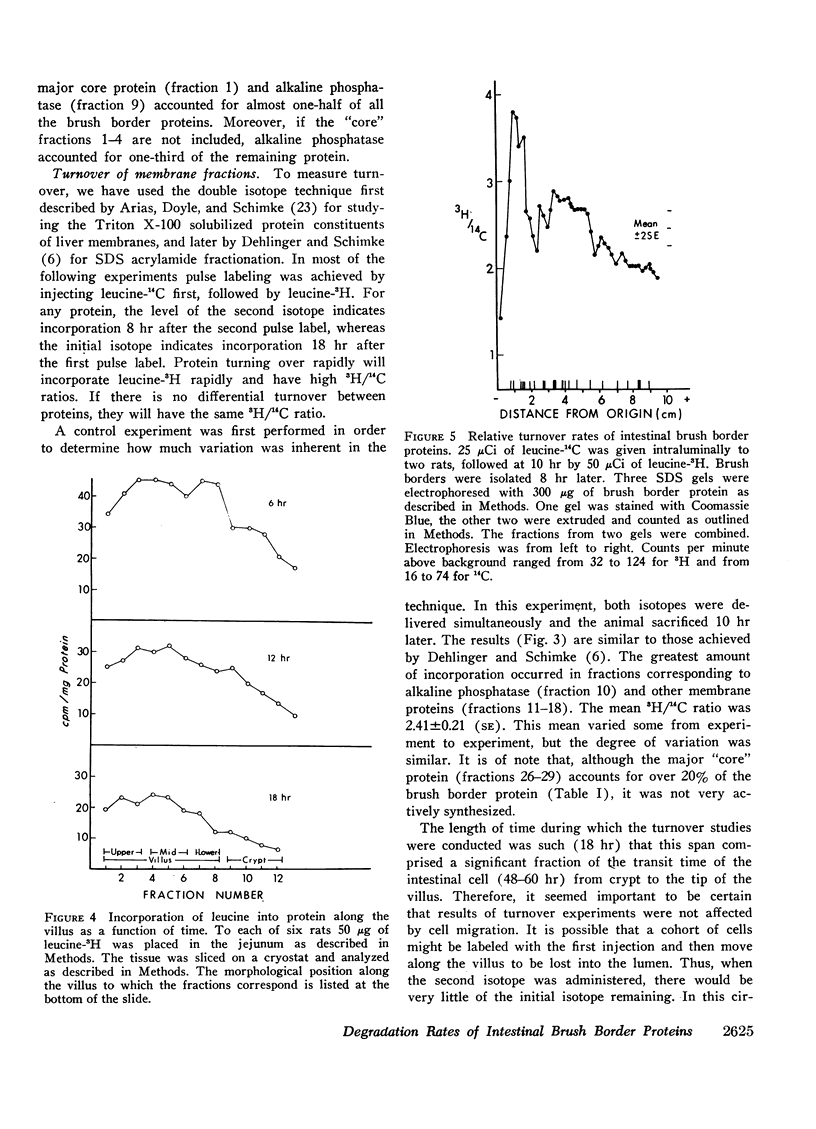
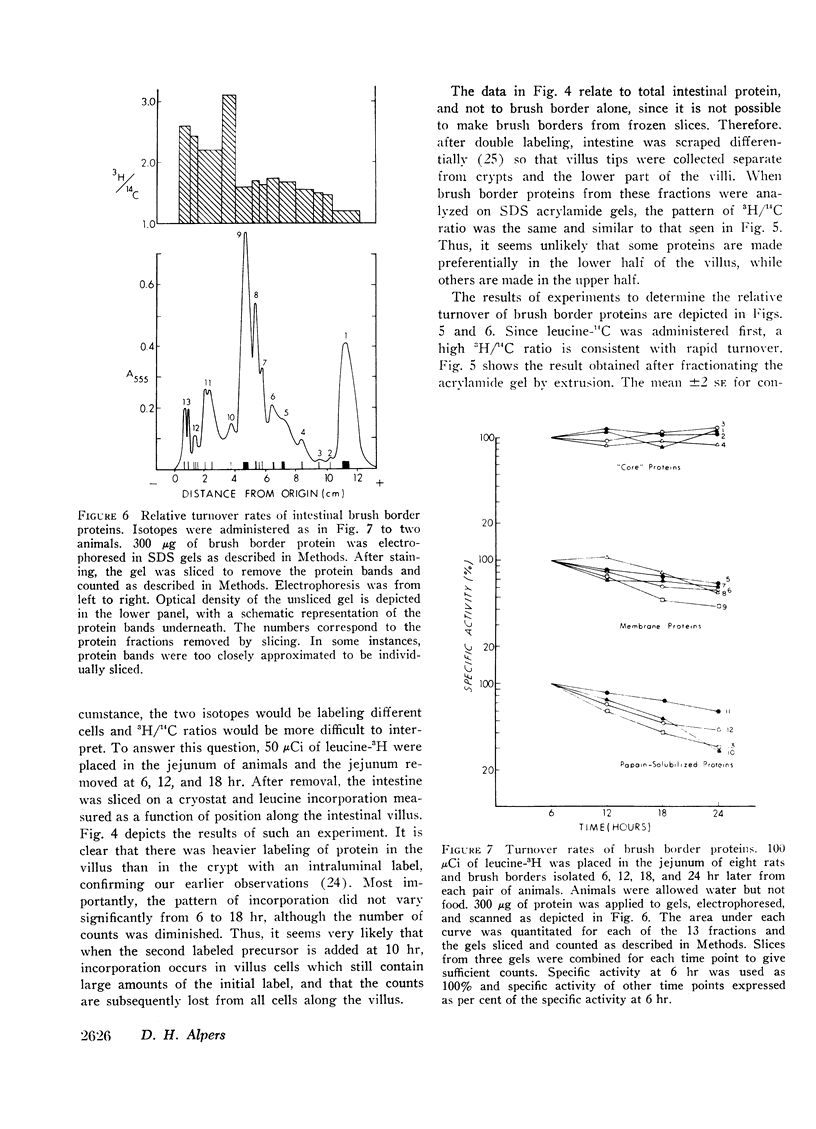
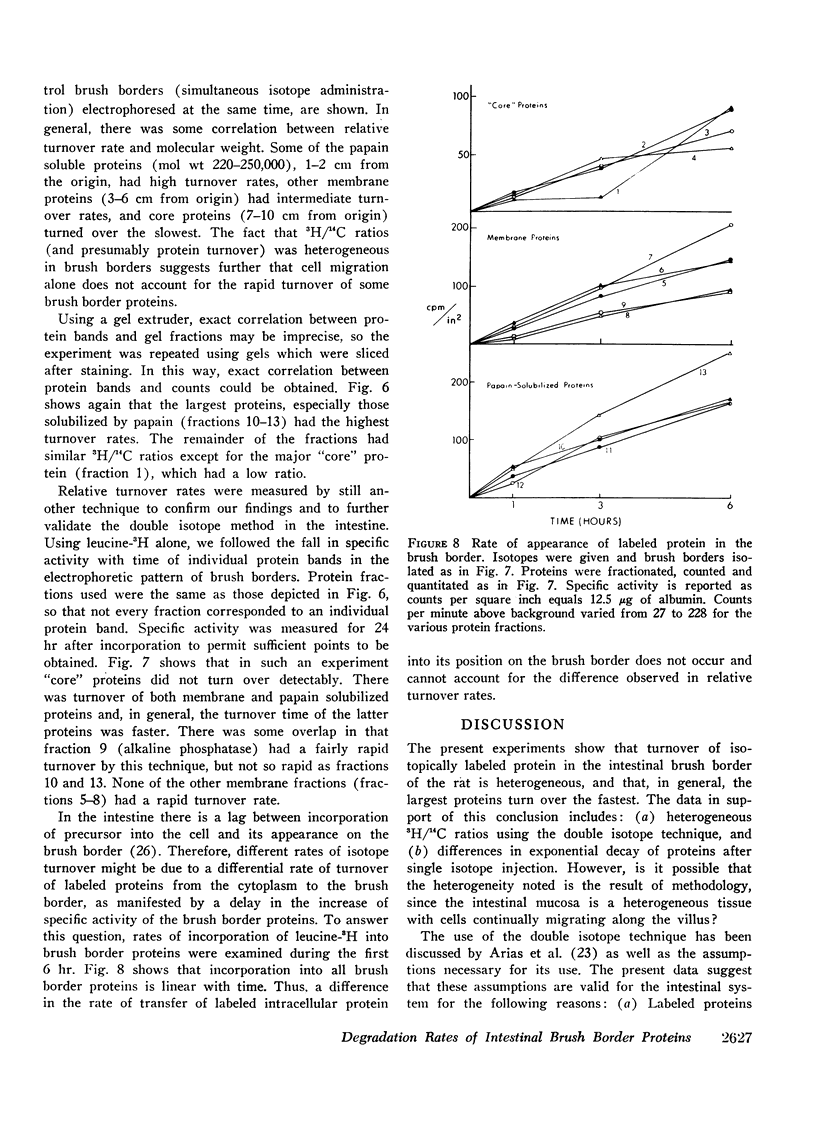
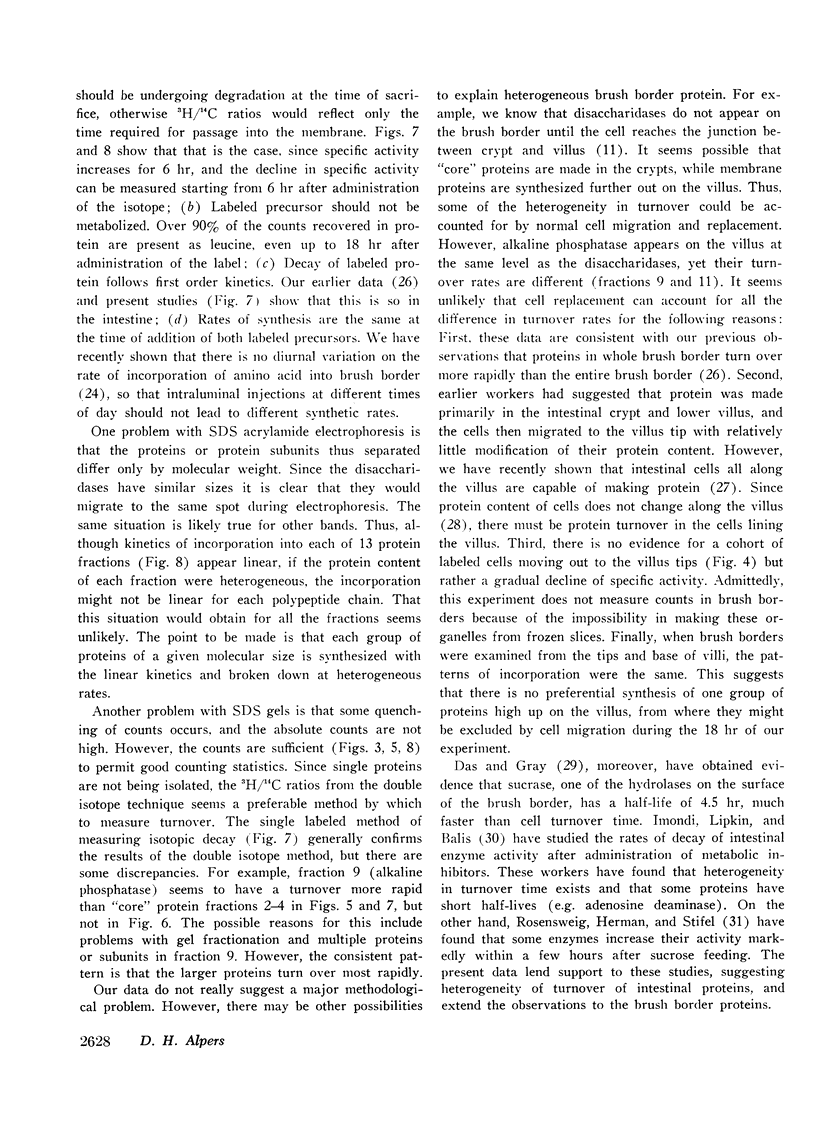
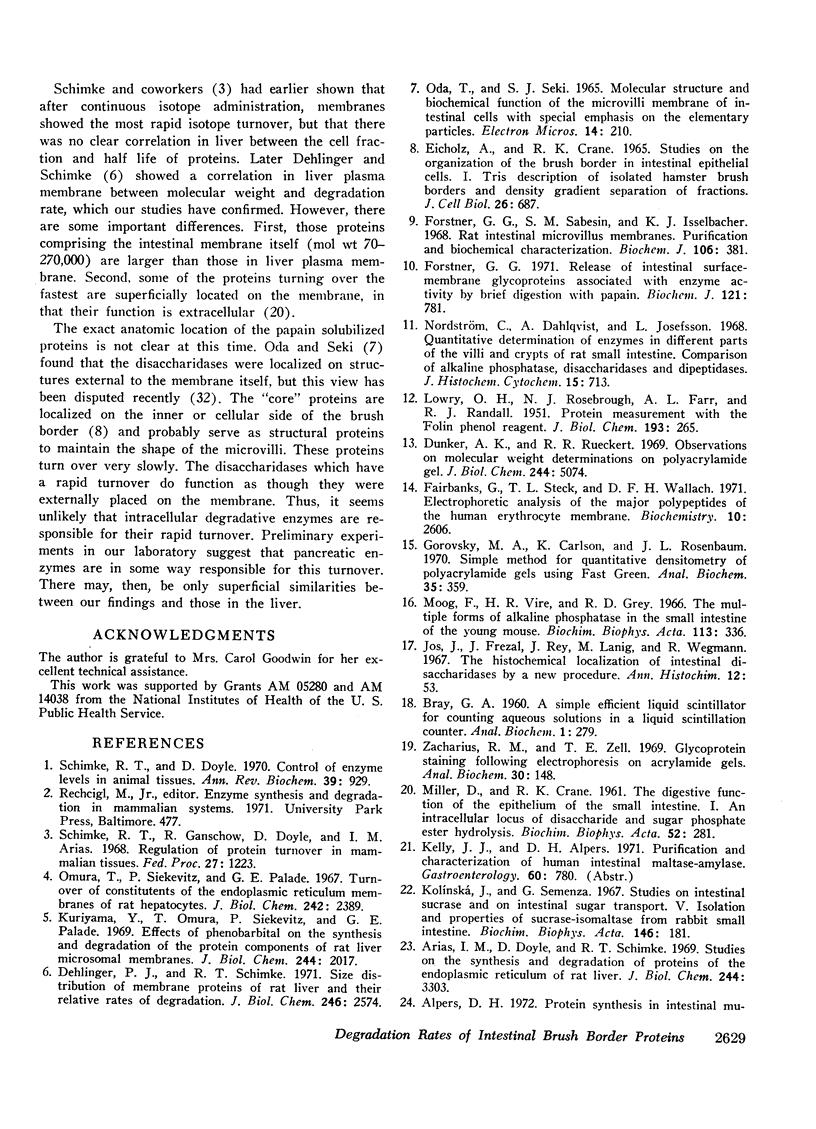
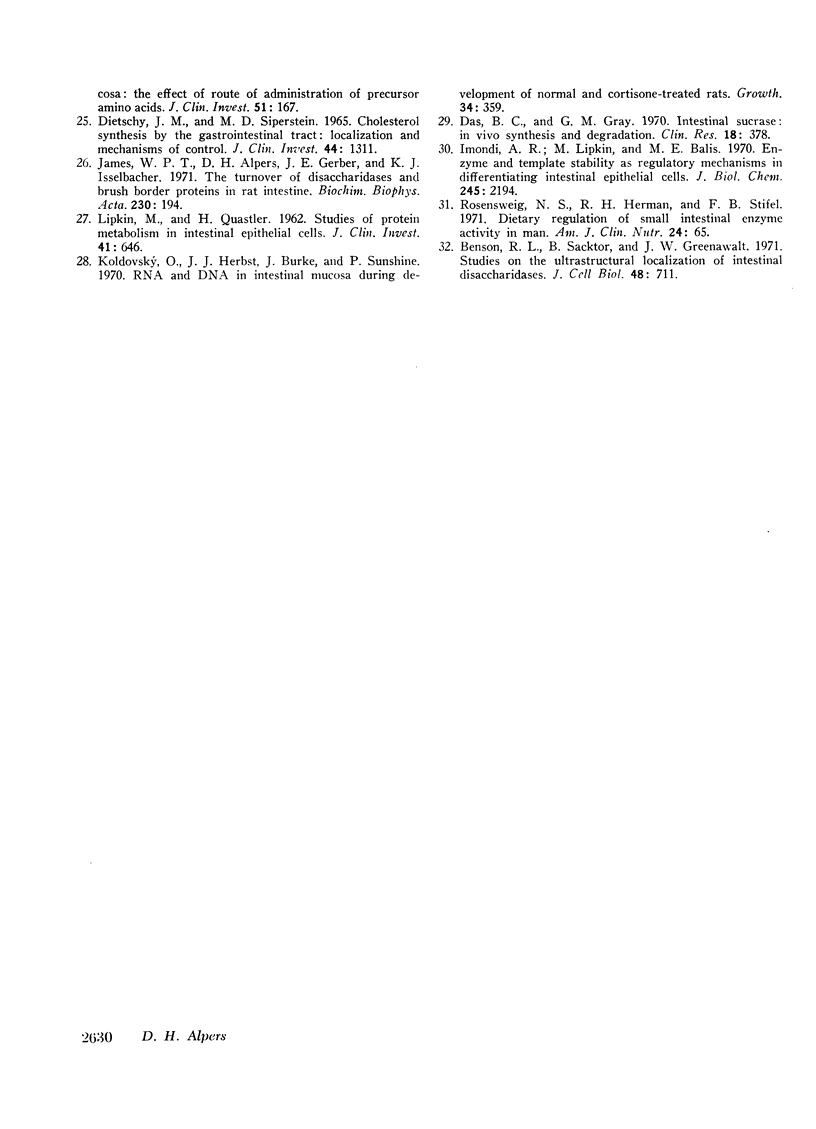
Images in this article
Selected References
These references are in PubMed. This may not be the complete list of references from this article.
- Alpers D. H. Protein synthesis in intestinal mucosa: the effect of route of administration of precursor amino acids. J Clin Invest. 1972 Jan;51(1):167–173. doi: 10.1172/JCI106788. [DOI] [PMC free article] [PubMed] [Google Scholar]
- Arias I. M., Doyle D., Schimke R. T. Studies on the synthesis and degradation of proteins of the endoplasmic reticulum of rat liver. J Biol Chem. 1969 Jun 25;244(12):3303–3315. [PubMed] [Google Scholar]
- Benson R. L., Sacktor B., Greenawalt J. W. Studies on the ultrastructural localization of intestinal disaccharidases. J Cell Biol. 1971 Mar;48(3):711–716. doi: 10.1083/jcb.48.3.711. [DOI] [PMC free article] [PubMed] [Google Scholar]
- Dehlinger P. J., Schimke R. T. Size distribution of membrane proteins of rat liver and their relative rates of degradation. J Biol Chem. 1971 Apr 25;246(8):2574–2583. [PubMed] [Google Scholar]
- Dietschy J. M., Siperstein M. D. Cholesterol synthesis by the gastrointestinal tract: localization and mechanisms of control. J Clin Invest. 1965 Aug;44(8):1311–1327. doi: 10.1172/JCI105237. [DOI] [PMC free article] [PubMed] [Google Scholar]
- Dunker A. K., Rueckert R. R. Observations on molecular weight determinations on polyacrylamide gel. J Biol Chem. 1969 Sep 25;244(18):5074–5080. [PubMed] [Google Scholar]
- Fairbanks G., Steck T. L., Wallach D. F. Electrophoretic analysis of the major polypeptides of the human erythrocyte membrane. Biochemistry. 1971 Jun 22;10(13):2606–2617. doi: 10.1021/bi00789a030. [DOI] [PubMed] [Google Scholar]
- Forstner G. G. Release of intestinal surface-membrane glycoproteins associated with enzyme activity by brief digestion with papain. Biochem J. 1971 Mar;121(5):781–789. doi: 10.1042/bj1210781. [DOI] [PMC free article] [PubMed] [Google Scholar]
- Forstner G. G., Sabesin S. M., Isselbacher K. J. Rat intestinal microvillus membranes. Purification and biochemical characterization. Biochem J. 1968 Jan;106(2):381–390. doi: 10.1042/bj1060381. [DOI] [PMC free article] [PubMed] [Google Scholar]
- Gorovsky M. A., Carlson K., Rosenbaum J. L. Simple method for quantitive densitometry of polyacrylamide gels using fast green. Anal Biochem. 1970 Jun;35(2):359–370. doi: 10.1016/0003-2697(70)90196-x. [DOI] [PubMed] [Google Scholar]
- Imondi A. R., Lipkin M., Balis M. E. Enzyme and template stability as regulatory mechanisms in differentiating intestinal epithelial cells. J Biol Chem. 1970 May 10;245(9):2194–2198. [PubMed] [Google Scholar]
- James W. P., Alpers D. H., Gerber J. E., Isselbacher K. J. The turnover of disaccharidases and brush border proteins in rat intestine. Biochim Biophys Acta. 1971 Feb 23;230(2):194–203. doi: 10.1016/0304-4165(71)90204-2. [DOI] [PubMed] [Google Scholar]
- Jos J., Frézal J., Rey J., Lamy M., Wegmann R. La localisation histochimique des disaccharidases intestinales par un nouveau procédé. Ann Histochim. 1967 Jan-Mar;12(1):53–61. [PubMed] [Google Scholar]
- Koldovský O., Herbst J. J., Burke J., Sunshine P. RNA and DNA in intestinal mucosa during development of normal and cortisone-treated rats. Growth. 1970 Dec;34(4):359–367. [PubMed] [Google Scholar]
- Kolínská J., Semenza G. Studies on intestinal sucrase and on intestinal sugar transport. V. Isolation and properties of sucrase-isomaltase from rabbit small intestine. Biochim Biophys Acta. 1967 Sep 12;146(1):181–195. doi: 10.1016/0005-2744(67)90085-x. [DOI] [PubMed] [Google Scholar]
- Kuriyama Y., Omura T., Siekevitz P., Palade G. E. Effects of phenobarbital on the synthesis and degradation of the protein components of rat liver microsomal membranes. J Biol Chem. 1969 Apr 25;244(8):2017–2026. [PubMed] [Google Scholar]
- LIPKIN M., QUASTLER H. Studies of protein metabolism in intestinal epithelial cells. J Clin Invest. 1962 Mar;41:646–653. doi: 10.1172/JCI104520. [DOI] [PMC free article] [PubMed] [Google Scholar]
- LOWRY O. H., ROSEBROUGH N. J., FARR A. L., RANDALL R. J. Protein measurement with the Folin phenol reagent. J Biol Chem. 1951 Nov;193(1):265–275. [PubMed] [Google Scholar]
- MILLER D., CRANE R. K. The digestive function of the epithelium of the small intestine. I. An intracellular locus of disaccharide and sugar phosphate ester hydrolysis. Biochim Biophys Acta. 1961 Sep 16;52:281–293. doi: 10.1016/0006-3002(61)90677-1. [DOI] [PubMed] [Google Scholar]
- Moog F., Vire H. R., Grey R. D. The multiple forms of alkaline phosphatase in the small intestine of the young mouse. Biochim Biophys Acta. 1966 Feb 14;113(2):336–349. doi: 10.1016/s0926-6593(66)80073-5. [DOI] [PubMed] [Google Scholar]
- Nordström C., Dahlqvist A., Josefsson L. Quantitative determination of enzymes in different parts of the villi and crypts of rat small intestine. Comparison of alkaline phosphatase, disaccharidases and dipepeptidases. J Histochem Cytochem. 1967 Dec;15(12):713–721. doi: 10.1177/15.12.713. [DOI] [PubMed] [Google Scholar]
- Omura T., Siekevitz P., Palade G. E. Turnover of constituents of the endoplasmic reticulum membranes of rat hepatocytes. J Biol Chem. 1967 May 25;242(10):2389–2396. [PubMed] [Google Scholar]
- Rosensweig N. S., Herman R. H., Stifel R. B. Dietary regulation of small intestinal enzyme activity in man. Am J Clin Nutr. 1971 Jan;24(1):65–69. doi: 10.1093/ajcn/24.1.65. [DOI] [PubMed] [Google Scholar]
- Schimke R. T., Doyle D. Control of enzyme levels in animal tissues. Annu Rev Biochem. 1970;39:929–976. doi: 10.1146/annurev.bi.39.070170.004433. [DOI] [PubMed] [Google Scholar]
- Schimke R. T., Ganschow R., Doyle D., Arias I. M. Regulation of protein turnover in mammalian tissues. Fed Proc. 1968 Sep-Oct;27(5):1223–1230. [PubMed] [Google Scholar]
- Zacharius R. M., Zell T. E., Morrison J. H., Woodlock J. J. Glycoprotein staining following electrophoresis on acrylamide gels. Anal Biochem. 1969 Jul;30(1):148–152. doi: 10.1016/0003-2697(69)90383-2. [DOI] [PubMed] [Google Scholar]