Abstract
The number of protons ejected during electron transport per pair of electrons per energy-conserving site (the H+/site ratio) was measured in rat liver mitochondria by three different methods under conditions in which transmembrane movements of endogenous phosphate were minized or eliminated. (1) In the Ca2+ pulse method, between 3.5 and 4.0 molecules of 3-hydroxybutyrate and 1.75 to 2.0 Ca2+ ions were accumulated per 2 e- per site during Ca2+ induced electron transport in the presence of rotenone, when measured under conditions in which movements of endogenous phosphate were negligible. Since entry of 3-hydroxybutyrate requires its protonation to the free acid these data correspond to an H+/site ratio of 3.5-4.0 (2) In the oxygen pulse method addition of known amounts of oxygen to anaerobic mitochondria in the presence of substrate yielded H+/site ratios of 3.0 when phosphate transport was eliminated by addition of N-ethylmaleimide or by anaerobic washing to remove endogenous phosphate. In the absence of such measures the observed H+/site ratio was 2.0. (3) In the reductant pulse method measurement of the initial steady rates of H+ ejection and oxygen consumption by mitochondria in an aerobic medium after addition of substrate gave H+/site near 4.0 in the presence of N-ethylmaleimide; in the absence of the inhibitor the observed ratio was only 2.0. These and other experiments reported indicate that the values of 2.0 earlier obtained for the H+/site ratio by Mitchell and Moyle [Biochem J. (1967) 105, 1147-1162] and others were underestimates due to the unrecognized masking of H+ ejection by movements of endogenous phosphate. The results presented here show that the H+/site ratio of mitochondrial electron transport is at least 3.0 and may be as high as 4.0.
Full text
PDF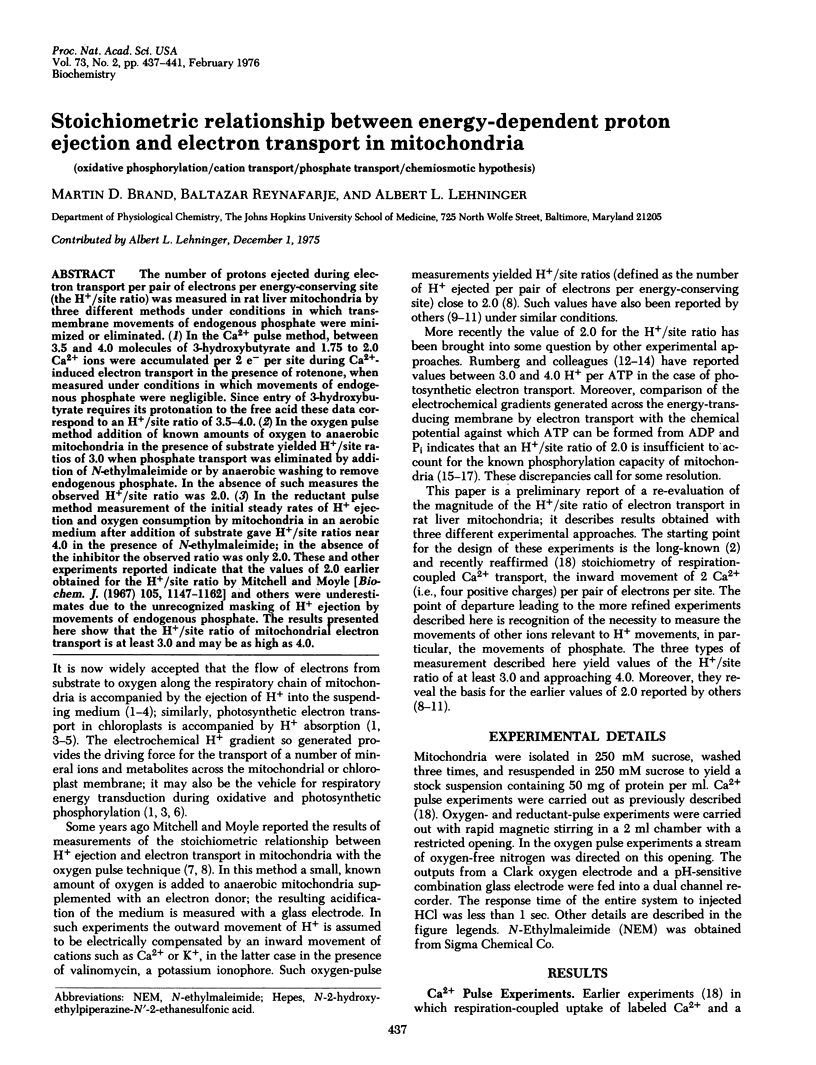
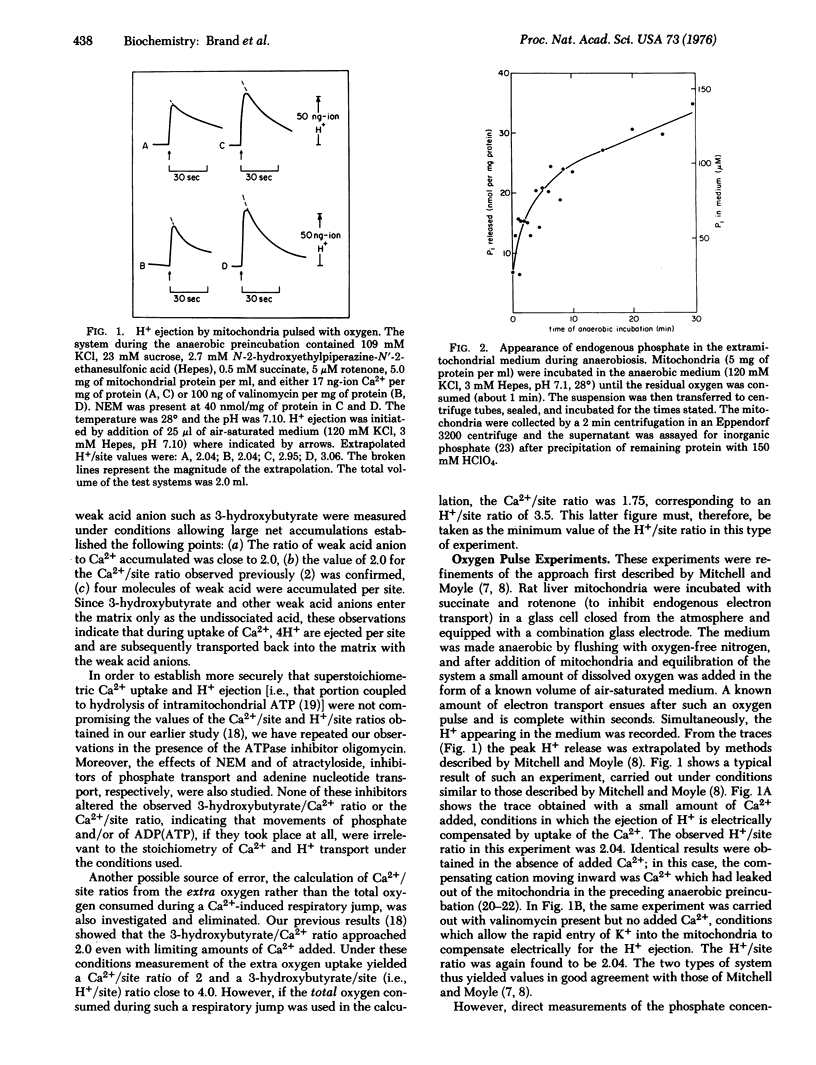
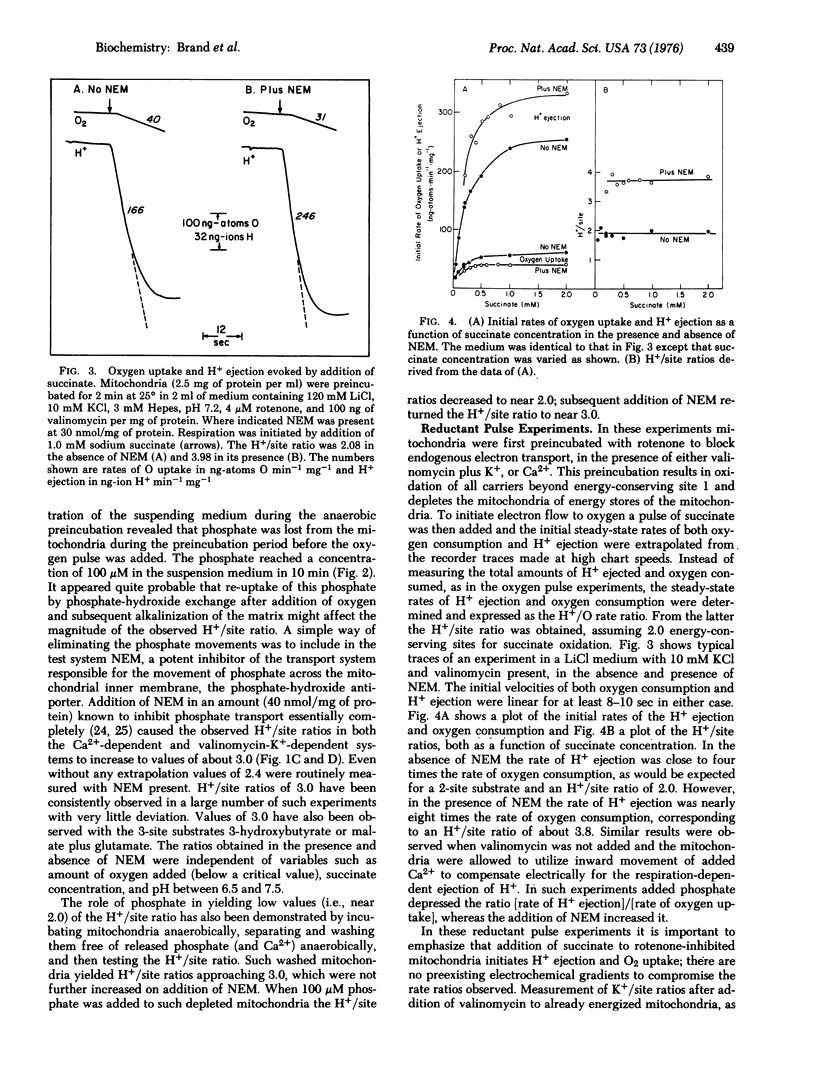
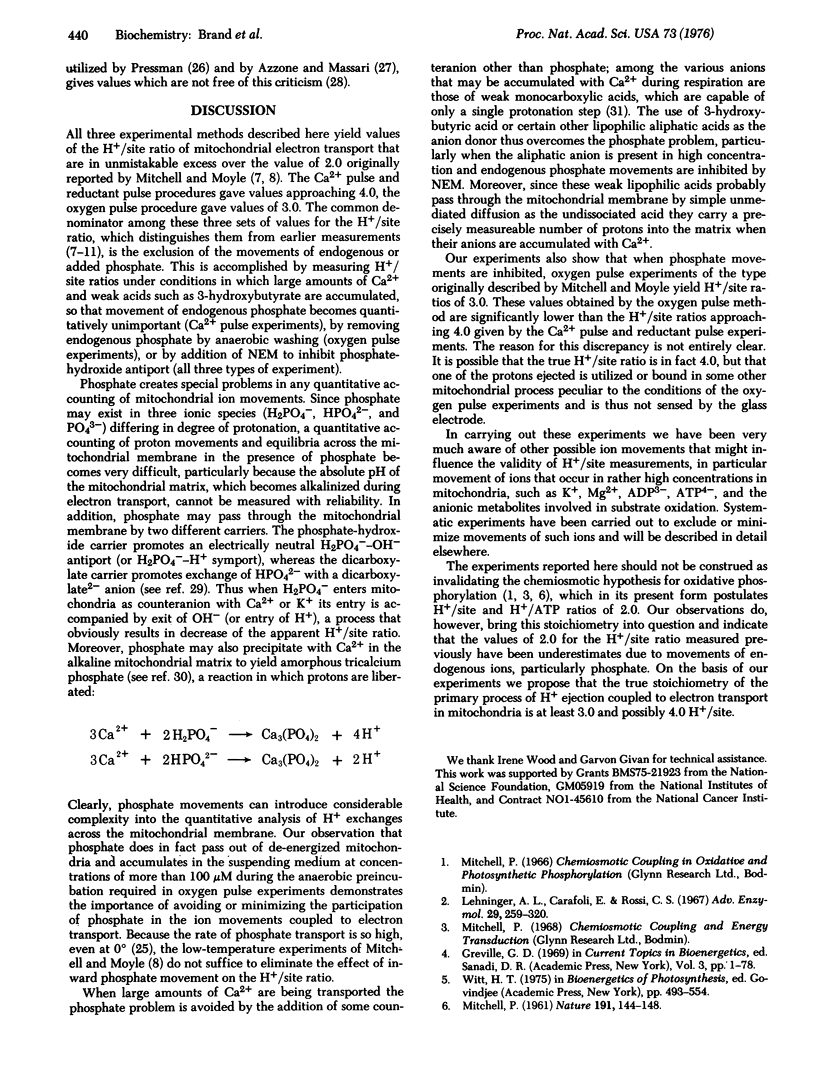
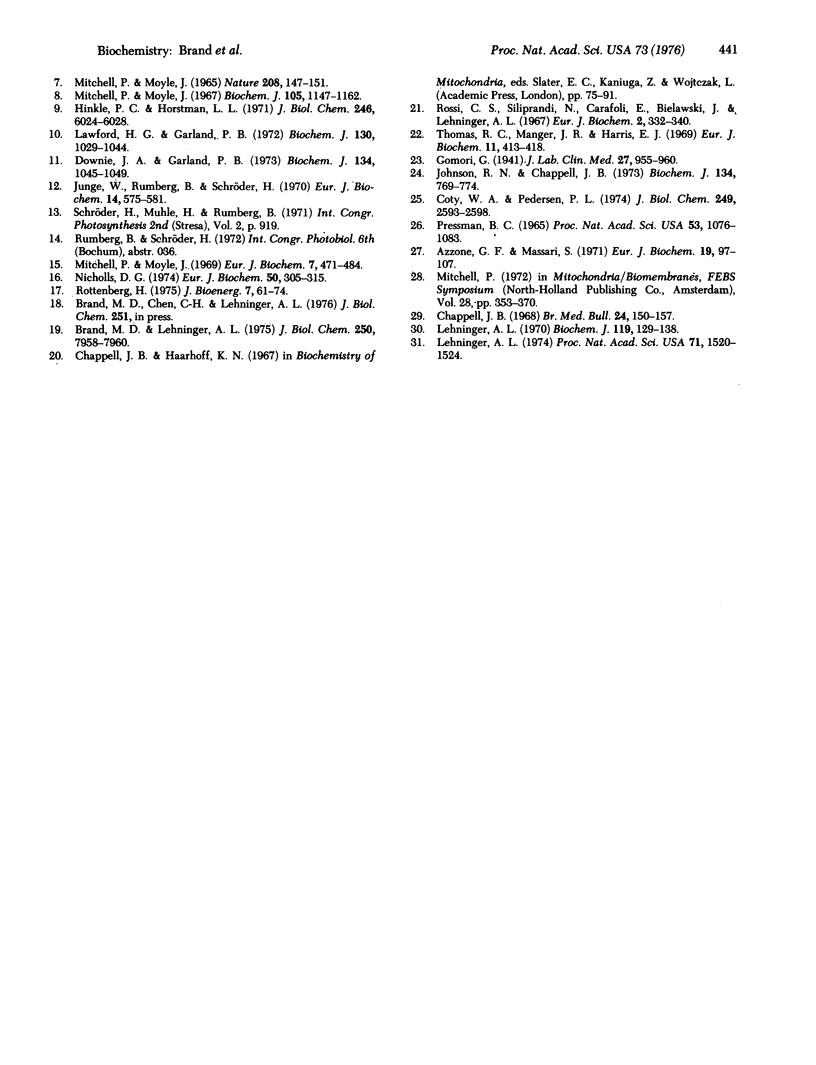
Selected References
These references are in PubMed. This may not be the complete list of references from this article.
- Azzone G. F., Massari S. Thermodynamic and kinetic aspects of the interconversion of chemical and osmotic energies in mitochondria. Eur J Biochem. 1971 Mar 1;19(1):97–107. doi: 10.1111/j.1432-1033.1971.tb01292.x. [DOI] [PubMed] [Google Scholar]
- Brand M. D., Lehninger A. L. Superstoichiometric Ca2+ uptake supported by hydrolysis of endogenous ATP in rat liver mitochondria. J Biol Chem. 1975 Oct 10;250(19):7958–7960. [PubMed] [Google Scholar]
- Chappell J. B. Systems used for the transport of substrates into mitochondria. Br Med Bull. 1968 May;24(2):150–157. doi: 10.1093/oxfordjournals.bmb.a070618. [DOI] [PubMed] [Google Scholar]
- Coty W. A., Pedersen P. L. Phosphate transport in rat liver mitochondria. Kinetics and energy requirements. J Biol Chem. 1974 Apr 25;249(8):2593–2598. [PubMed] [Google Scholar]
- Downie J. A., Garland P. B. Respiration-driven proton translocation by yeast mitochondria with differing efficiencies of oxidative phosphorylation. Biochem J. 1973 Aug;134(4):1045–1049. doi: 10.1042/bj1341045. [DOI] [PMC free article] [PubMed] [Google Scholar]
- Hinkle P. C., Horstman L. L. Respiration-driven proton transport in submitochondrial particles. J Biol Chem. 1971 Oct 10;246(19):6024–6028. [PubMed] [Google Scholar]
- Johnson R. N., Chappell J. B. The transport of inorganic phosphate by the mitochondrial dicarboxylate carrier. Biochem J. 1973 Jul;134(3):769–774. doi: 10.1042/bj1340769. [DOI] [PMC free article] [PubMed] [Google Scholar]
- Junge W., Rumberg B., Schröder H. The necessity of an electric potential difference and its use for photophosphorylation in short flash groups. Eur J Biochem. 1970 Jul;14(3):575–581. doi: 10.1111/j.1432-1033.1970.tb00326.x. [DOI] [PubMed] [Google Scholar]
- Lawford H. G., Garland P. B. Proton translocation coupled to quinone reduction by reduced nicotinamide--adenine dinucleotide in rat liver and ox heart mitochondria. Biochem J. 1972 Dec;130(4):1029–1044. doi: 10.1042/bj1301029. [DOI] [PMC free article] [PubMed] [Google Scholar]
- Lehninger A. L., Carafoli E., Rossi C. S. Energy-linked ion movements in mitochondrial systems. Adv Enzymol Relat Areas Mol Biol. 1967;29:259–320. doi: 10.1002/9780470122747.ch6. [DOI] [PubMed] [Google Scholar]
- Lehninger A. L. Mitochondria and calcium ion transport. Biochem J. 1970 Sep;119(2):129–138. doi: 10.1042/bj1190129. [DOI] [PMC free article] [PubMed] [Google Scholar]
- Lehninger A. L. Role of phosphate and other proton-donating anions in respiration-coupled transport of Ca2+ by mitochondria. Proc Natl Acad Sci U S A. 1974 Apr;71(4):1520–1524. doi: 10.1073/pnas.71.4.1520. [DOI] [PMC free article] [PubMed] [Google Scholar]
- MITCHELL P. Coupling of phosphorylation to electron and hydrogen transfer by a chemi-osmotic type of mechanism. Nature. 1961 Jul 8;191:144–148. doi: 10.1038/191144a0. [DOI] [PubMed] [Google Scholar]
- Mitchell P., Moyle J. Estimation of membrane potential and pH difference across the cristae membrane of rat liver mitochondria. Eur J Biochem. 1969 Feb;7(4):471–484. doi: 10.1111/j.1432-1033.1969.tb19633.x. [DOI] [PubMed] [Google Scholar]
- Mitchell P., Moyle J. Respiration-driven proton translocation in rat liver mitochondria. Biochem J. 1967 Dec;105(3):1147–1162. doi: 10.1042/bj1051147. [DOI] [PMC free article] [PubMed] [Google Scholar]
- Mitchell P., Moyle J. Stoichiometry of proton translocation through the respiratory chain and adenosine triphosphatase systems of rat liver mitochondria. Nature. 1965 Oct 9;208(5006):147–151. doi: 10.1038/208147a0. [DOI] [PubMed] [Google Scholar]
- Nicholls D. G. The influence of respiration and ATP hydrolysis on the proton-electrochemical gradient across the inner membrane of rat-liver mitochondria as determined by ion distribution. Eur J Biochem. 1974 Dec 16;50(1):305–315. doi: 10.1111/j.1432-1033.1974.tb03899.x. [DOI] [PubMed] [Google Scholar]
- Pressman B. C. Induced active transport of ions in mitochondria. Proc Natl Acad Sci U S A. 1965 May;53(5):1076–1083. doi: 10.1073/pnas.53.5.1076. [DOI] [PMC free article] [PubMed] [Google Scholar]
- Rossi C. S., Siliprandi N., Carafoli E., Bielawski J., Lehninger A. L. Proton movements across the mitochondrial membrane supported by hydrolysis of adenosine triphosphate. Eur J Biochem. 1967 Oct;2(3):332–340. doi: 10.1111/j.1432-1033.1967.tb00143.x. [DOI] [PubMed] [Google Scholar]
- Rottenberg H. The measurement of transmembrane electrochemical proton gradients. J Bioenerg. 1975 May;7(2):61–74. doi: 10.1007/BF01558427. [DOI] [PubMed] [Google Scholar]
- Thomas R. C., Manger J. R., Harris E. J. Cation uptake as the basis for production of proton pulses by mitochondria at the anaerobic-aerobic transition. Eur J Biochem. 1969 Dec;11(3):413–418. doi: 10.1111/j.1432-1033.1969.tb00789.x. [DOI] [PubMed] [Google Scholar]