Abstract
The mechanism for active transport of ions across a membrane probably involves two distinct conformational states of the transport protein, in which the binding sites for the transported ion face opposite sides of the membrane. It is likely that the binding affinity for the ion changes in synchrony with the change in site orientation, such that the affinity is high on the uptake side of the membrane and low on the discharge side. A structural model is proposed for the transmembrane portion of such a protein, based on the known multihelical structure of bacteriorhodopsin. This structure is well adapted to a cyclical alternation between two conformations that differ simultaneously in orientation and binding affinity. No unfolding of the helices or other significant alterations in secondary structure is required. The model is explicitly intended as a hypothetical representation of the E1 and E2 states of ATP-driven Na+,K+ and Ca2+ pumps.
Full text
PDF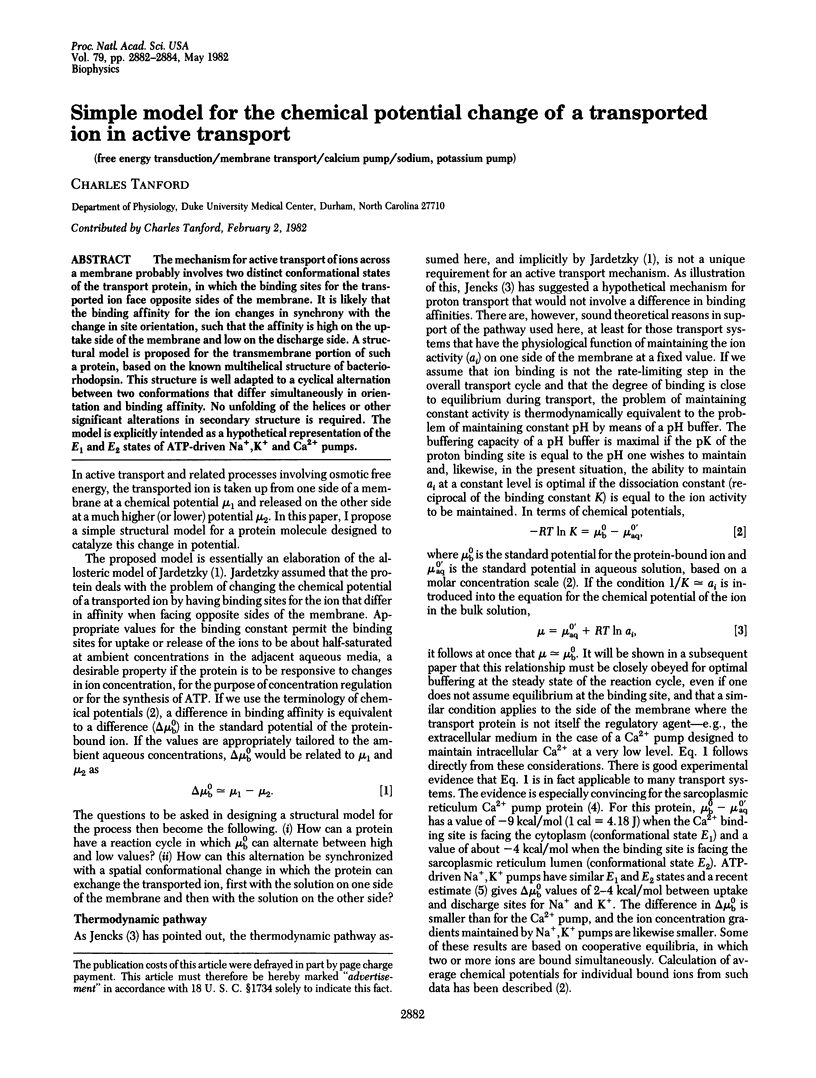
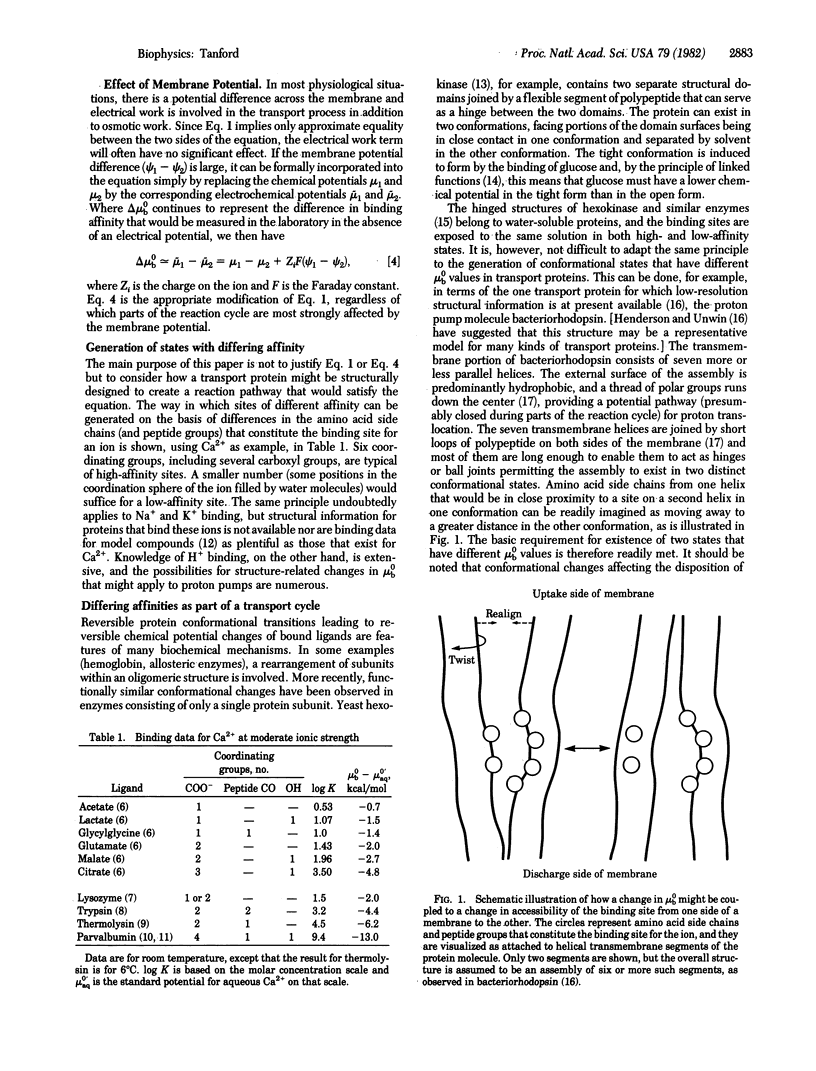
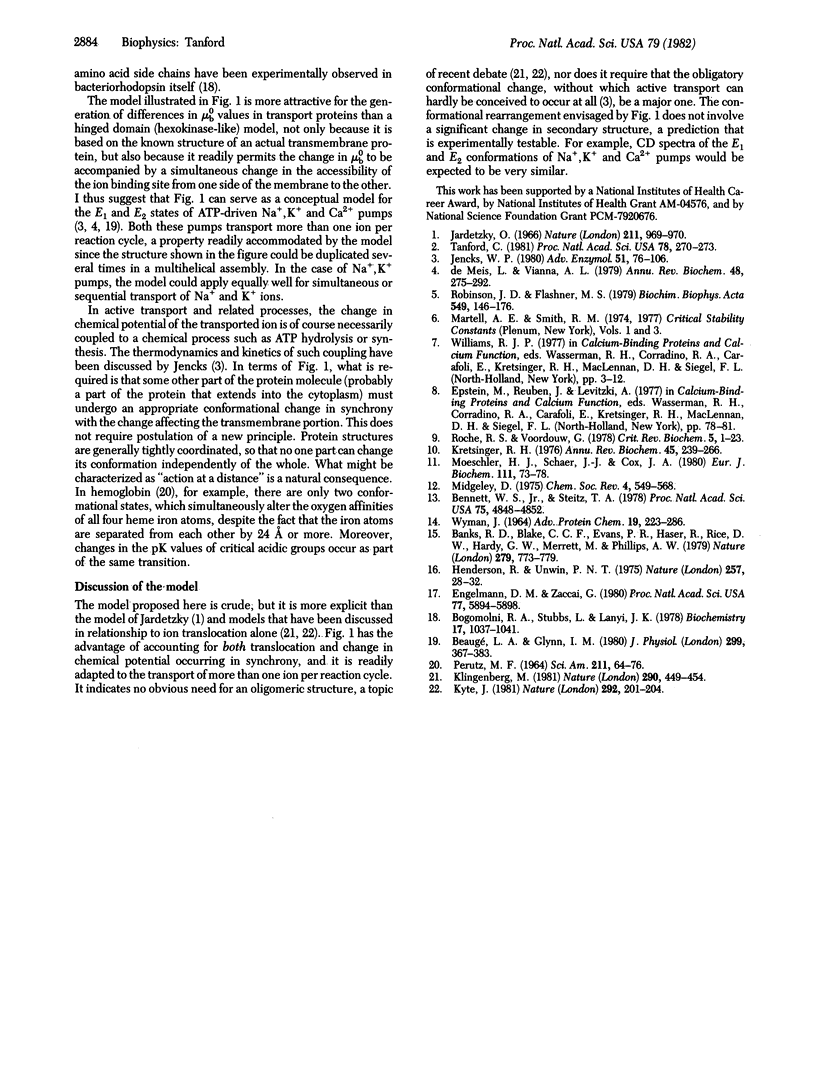
Selected References
These references are in PubMed. This may not be the complete list of references from this article.
- Banks R. D., Blake C. C., Evans P. R., Haser R., Rice D. W., Hardy G. W., Merrett M., Phillips A. W. Sequence, structure and activity of phosphoglycerate kinase: a possible hinge-bending enzyme. Nature. 1979 Jun 28;279(5716):773–777. doi: 10.1038/279773a0. [DOI] [PubMed] [Google Scholar]
- Beaugé L. A., Glynn I. M. The equilibrium between different conformations of the unphosphorylated sodium pump: effects of ATP and of potassium ions, and their relevance to potassium transport. J Physiol. 1980 Feb;299:367–383. doi: 10.1113/jphysiol.1980.sp013130. [DOI] [PMC free article] [PubMed] [Google Scholar]
- Bennett W. S., Jr, Steitz T. A. Glucose-induced conformational change in yeast hexokinase. Proc Natl Acad Sci U S A. 1978 Oct;75(10):4848–4852. doi: 10.1073/pnas.75.10.4848. [DOI] [PMC free article] [PubMed] [Google Scholar]
- Bogomolni R. A., Stubbs L., Lanyi J. K. Illumination-dependent changes in the intrinsic fluorescence of bacteriorhodopsin. Biochemistry. 1978 Mar 21;17(6):1037–1041. doi: 10.1021/bi00599a015. [DOI] [PubMed] [Google Scholar]
- Engelman D. M., Zaccai G. Bacteriorhodopsin is an inside-out protein. Proc Natl Acad Sci U S A. 1980 Oct;77(10):5894–5898. doi: 10.1073/pnas.77.10.5894. [DOI] [PMC free article] [PubMed] [Google Scholar]
- Henderson R., Unwin P. N. Three-dimensional model of purple membrane obtained by electron microscopy. Nature. 1975 Sep 4;257(5521):28–32. doi: 10.1038/257028a0. [DOI] [PubMed] [Google Scholar]
- Jardetzky O. Simple allosteric model for membrane pumps. Nature. 1966 Aug 27;211(5052):969–970. doi: 10.1038/211969a0. [DOI] [PubMed] [Google Scholar]
- Jencks W. P. The utilization of binding energy in coupled vectorial processes. Adv Enzymol Relat Areas Mol Biol. 1980;51:75–106. doi: 10.1002/9780470122969.ch2. [DOI] [PubMed] [Google Scholar]
- Klingenberg M. Membrane protein oligomeric structure and transport function. Nature. 1981 Apr 9;290(5806):449–454. doi: 10.1038/290449a0. [DOI] [PubMed] [Google Scholar]
- Kretsinger R. H. Calcium-binding proteins. Annu Rev Biochem. 1976;45:239–266. doi: 10.1146/annurev.bi.45.070176.001323. [DOI] [PubMed] [Google Scholar]
- Kyte J. Molecular considerations relevant to the mechanism of active transport. Nature. 1981 Jul 16;292(5820):201–204. doi: 10.1038/292201a0. [DOI] [PubMed] [Google Scholar]
- Moeschler H. J., Schaer J. J., Cox J. A. A thermodynamic analysis of the binding of calcium and magnesium ions to parvalbumin. Eur J Biochem. 1980 Oct;111(1):73–78. doi: 10.1111/j.1432-1033.1980.tb06076.x. [DOI] [PubMed] [Google Scholar]
- PERUTZ M. F. THE HEMOGLOBIN MOLECULE. Sci Am. 1964 Nov;211:64–76. doi: 10.1038/scientificamerican1164-64. [DOI] [PubMed] [Google Scholar]
- Robinson J. D., Flashner M. S. The (Na+ + K+)-activated ATPase. Enzymatic and transport properties. Biochim Biophys Acta. 1979 Aug 17;549(2):145–176. doi: 10.1016/0304-4173(79)90013-2. [DOI] [PubMed] [Google Scholar]
- Roche R. S., Voordouw G. The structural and functional roles of metal ions in thermolysin. CRC Crit Rev Biochem. 1978;5(1):1–23. doi: 10.3109/10409237809177138. [DOI] [PubMed] [Google Scholar]
- Tanford C. Chemical potential of bound ligand, an important parameter for free energy transduction. Proc Natl Acad Sci U S A. 1981 Jan;78(1):270–273. doi: 10.1073/pnas.78.1.270. [DOI] [PMC free article] [PubMed] [Google Scholar]
- WYMAN J., Jr LINKED FUNCTIONS AND RECIPROCAL EFFECTS IN HEMOGLOBIN: A SECOND LOOK. Adv Protein Chem. 1964;19:223–286. doi: 10.1016/s0065-3233(08)60190-4. [DOI] [PubMed] [Google Scholar]
- de Meis L., Vianna A. L. Energy interconversion by the Ca2+-dependent ATPase of the sarcoplasmic reticulum. Annu Rev Biochem. 1979;48:275–292. doi: 10.1146/annurev.bi.48.070179.001423. [DOI] [PubMed] [Google Scholar]