Abstract
Diastolic depolarization in cardiac muscle is due to a decline in potassium permeability that has been ascribed to removal of intracellular free calcium. A continued decline in tension during the pacemaker potential might therefore occur. In this study, contractile responses of chicken embryonic heart cell aggregates are recorded with a photodiode. Photodiode output is well correlated with the position of the aggregate's edge. Movements of different edges are synchronous, and their amplitude and duration vary appropriately during experimental maneuvers that alter the magnitude and duration of contractile force. Edge movement during relaxation has two phases, a rapid phase lasting about 100 msec and a slow phase that may last over 10 sec. The slow phase is not due to viscoelasticity because its time course does not depend on the magnitude or duration of the initial deformation. The rate of relaxation is correlated with the rate of depolarization during the pacemaker potential. Reduction in automaticity during cooling, spontaneous variation, and overdrive pacing are associated with impairment of the slow component of relaxation. Electrophysiological evidence suggests that the diastolic potassium permeability of the aggregates is controlled by intracellular calcium. A possible explanation for the correlation between the slope of the pacemaker potential and the slow component of relaxation is that both phenomena reflect a common physiological process—i.e., the removal of free calcium from the cytoplasm.
Keywords: cardiac relaxation, potassium permeability, pacemaker physiology, intracellular calcium
Full text
PDF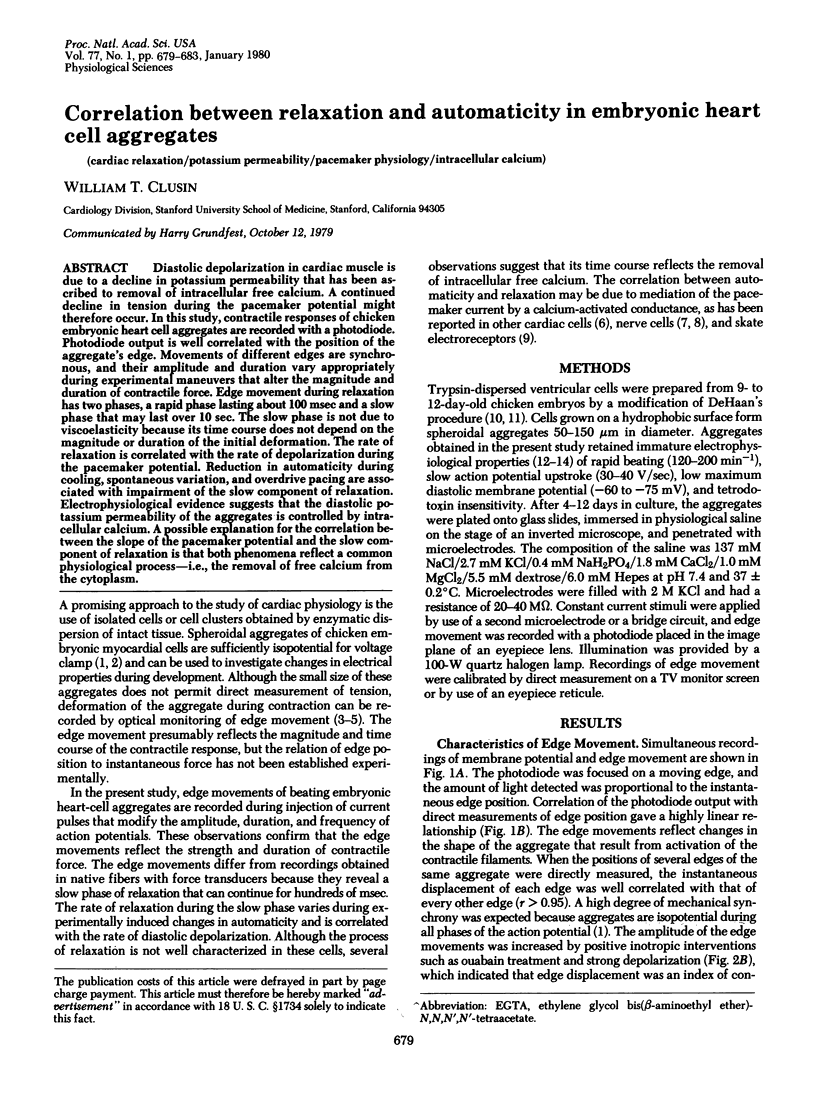
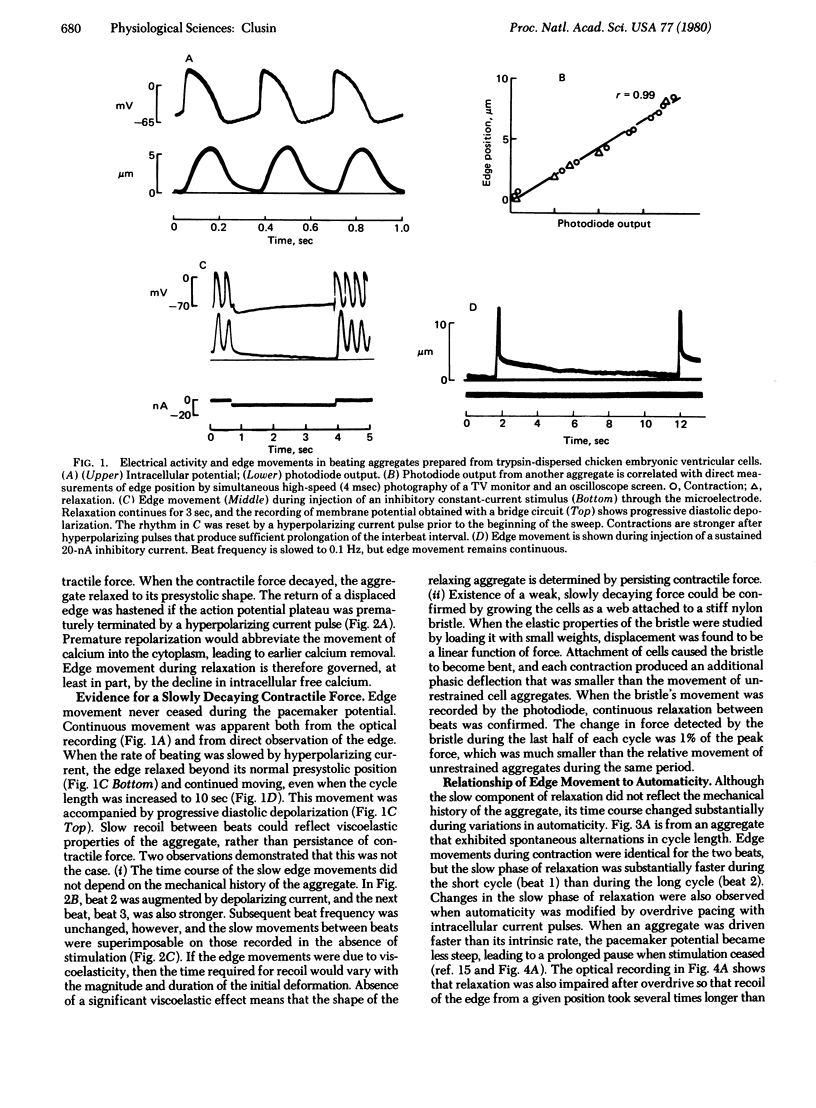
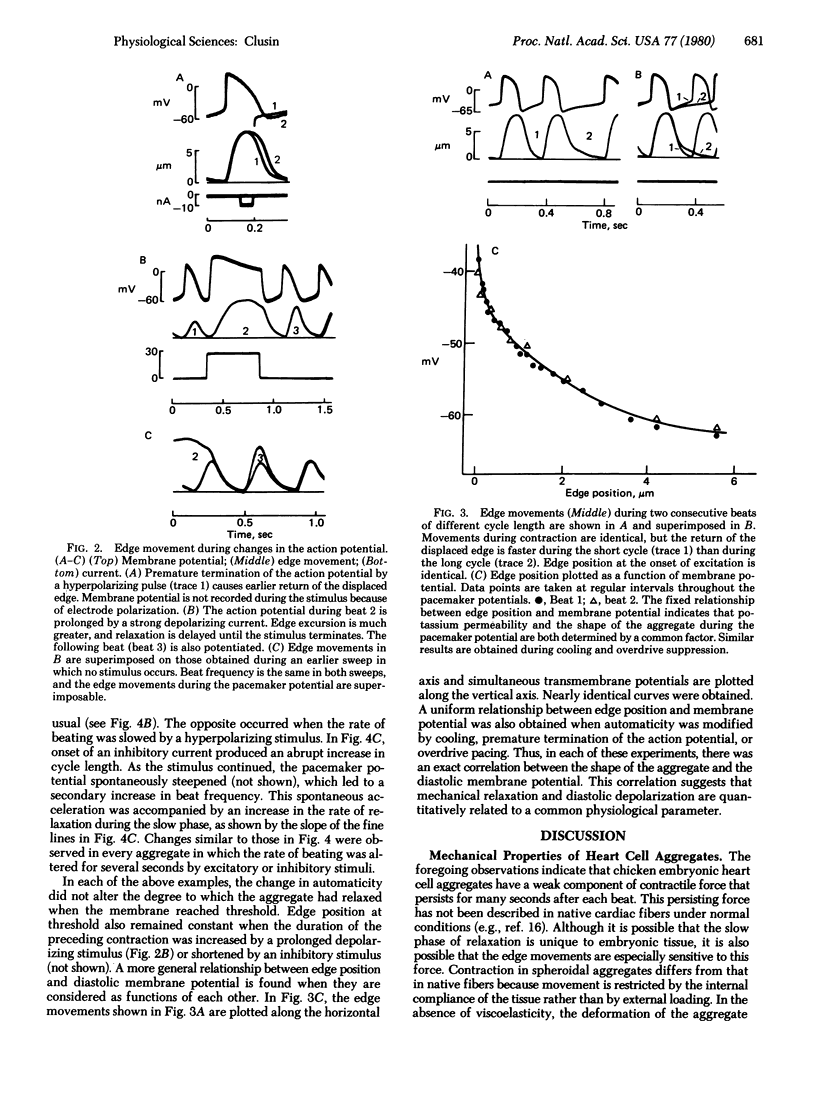
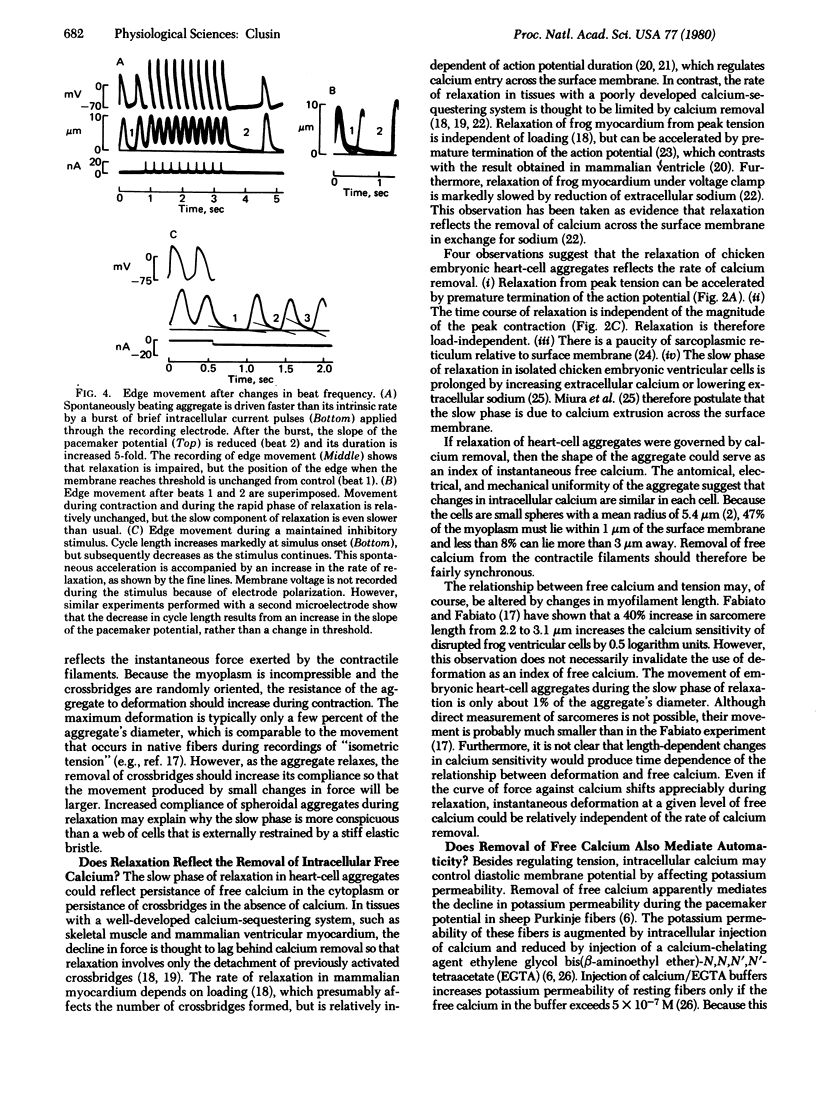
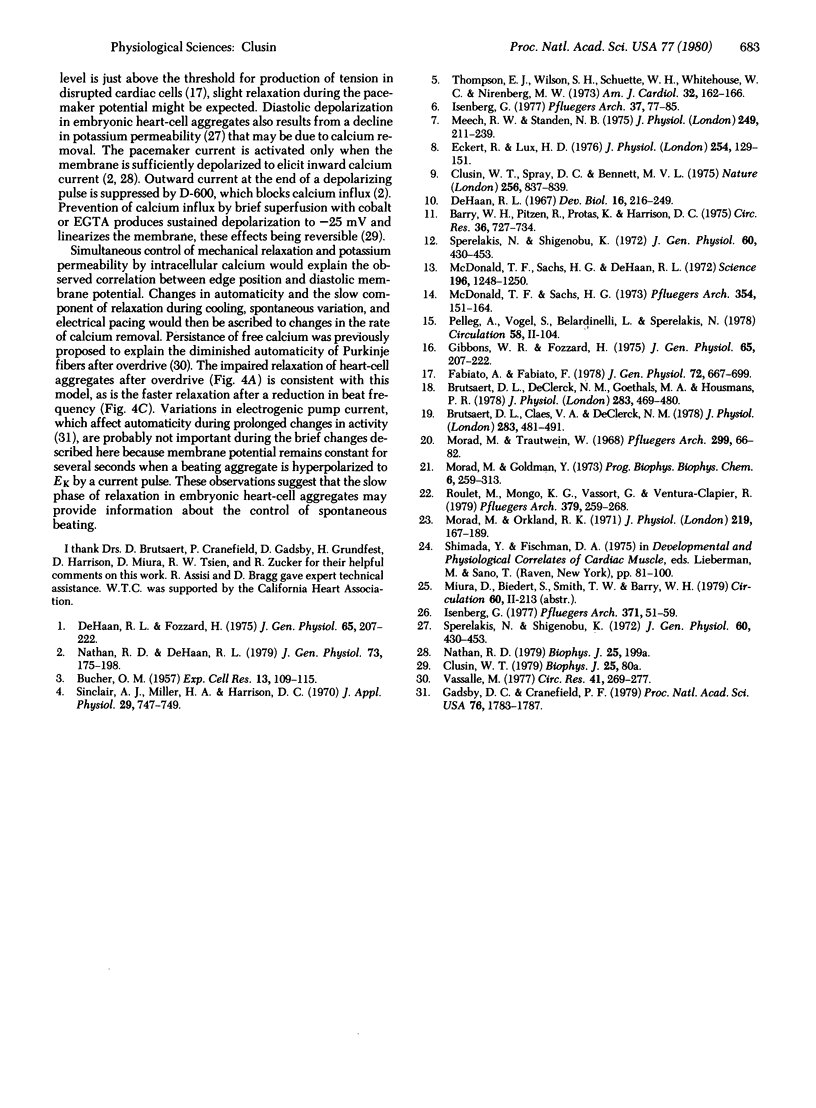
Selected References
These references are in PubMed. This may not be the complete list of references from this article.
- BUCHER O. M. A photoelectric recording set for pulsation curves of heart muscle cultures in vitro. Exp Cell Res. 1957 Aug;13(1):109–115. doi: 10.1016/0014-4827(57)90050-2. [DOI] [PubMed] [Google Scholar]
- Barry W. H., Pitzen R., Protas K., Harrison D. C. Inotropic effects of different calcium ion concentration on the embryonic chick ventricle. Comparison of single cultured cells and intact muscle strips. Circ Res. 1975 Jun;36(6):727–734. doi: 10.1161/01.res.36.6.727. [DOI] [PubMed] [Google Scholar]
- Brutsaert D. L., Claes V. A., De Clerck N. M. Relaxation of mammalian single cardiac cells after pretreatment with the detergent Brij-58. J Physiol. 1978 Oct;283:481–491. doi: 10.1113/jphysiol.1978.sp012514. [DOI] [PMC free article] [PubMed] [Google Scholar]
- Brutsaert D. L., de Clerck N. M., Goethals M. A., Housmans P. R. Relaxation of ventricular cardiac muscle. J Physiol. 1978 Oct;283:469–480. doi: 10.1113/jphysiol.1978.sp012513. [DOI] [PMC free article] [PubMed] [Google Scholar]
- DeHann R. L. Regulation of spontaneous activity and growth of embryonic chick heart cells in tissue culture. Dev Biol. 1967 Sep;16(3):216–249. doi: 10.1016/0012-1606(67)90025-5. [DOI] [PubMed] [Google Scholar]
- Dehaan R. L., Fozzard H. A. Membrane response to current pulses in spheroidal aggregates of embryonic heart cells. J Gen Physiol. 1975 Feb;65(2):207–222. doi: 10.1085/jgp.65.2.207. [DOI] [PMC free article] [PubMed] [Google Scholar]
- Dehaan R. L., Fozzard H. A. Membrane response to current pulses in spheroidal aggregates of embryonic heart cells. J Gen Physiol. 1975 Feb;65(2):207–222. doi: 10.1085/jgp.65.2.207. [DOI] [PMC free article] [PubMed] [Google Scholar]
- Eckert R., Lux H. D. A voltage-sensitive persistent calcium conductance in neuronal somata of Helix. J Physiol. 1976 Jan;254(1):129–151. doi: 10.1113/jphysiol.1976.sp011225. [DOI] [PMC free article] [PubMed] [Google Scholar]
- Fabiato A., Fabiato F. Myofilament-generated tension oscillations during partial calcium activation and activation dependence of the sarcomere length-tension relation of skinned cardiac cells. J Gen Physiol. 1978 Nov;72(5):667–699. doi: 10.1085/jgp.72.5.667. [DOI] [PMC free article] [PubMed] [Google Scholar]
- Gadsby D. C., Cranefield P. F. Direct measurement of changes in sodium pump current in canine cardiac Purkinje fibers. Proc Natl Acad Sci U S A. 1979 Apr;76(4):1783–1787. doi: 10.1073/pnas.76.4.1783. [DOI] [PMC free article] [PubMed] [Google Scholar]
- Isenberg G. Cardiac Purkinje fibres: [Ca2+]i controls the potassium permeability via the conductance components gK1 and gK2. Pflugers Arch. 1977 Oct 19;371(1-2):77–85. doi: 10.1007/BF00580775. [DOI] [PubMed] [Google Scholar]
- Isenberg G. Cardiac Purkinje fibres: resting, action, and pacemaker potential under the influence of [Ca2+]i as modified by intracellular injection techniques. Pflugers Arch. 1977 Oct 19;371(1-2):51–59. doi: 10.1007/BF00580772. [DOI] [PubMed] [Google Scholar]
- McDonald T. F., Sachs H. G., DeHaan R. L. Development of sensitivity to tetrodotoxin in beating chick embryo hearts, single cells, and aggregates. Science. 1972 Jun 16;176(4040):1248–1250. doi: 10.1126/science.176.4040.1248. [DOI] [PubMed] [Google Scholar]
- McDonald T. F., Sachs H. G. Electrical activity in embryonic heart cell aggregates. Developmental aspects. Pflugers Arch. 1975;354(2):151–164. doi: 10.1007/BF00579945. [DOI] [PubMed] [Google Scholar]
- Meech R. W., Standen N. B. Potassium activation in Helix aspersa neurones under voltage clamp: a component mediated by calcium influx. J Physiol. 1975 Jul;249(2):211–239. doi: 10.1113/jphysiol.1975.sp011012. [DOI] [PMC free article] [PubMed] [Google Scholar]
- Morad M., Orkand R. K. Excitation-concentration coupling in frog ventricle: evidence from voltage clamp studies. J Physiol. 1971 Dec;219(1):167–189. doi: 10.1113/jphysiol.1971.sp009656. [DOI] [PMC free article] [PubMed] [Google Scholar]
- Morad M., Trautwein W. The effect of the duration of the action potential on contraction in the mammalian heart muscle. Pflugers Arch Gesamte Physiol Menschen Tiere. 1968;299(1):66–82. doi: 10.1007/BF00362542. [DOI] [PubMed] [Google Scholar]
- Nathan R. D., DeHaan R. L. Voltage clamp analysis of embryonic heart cell aggregates. J Gen Physiol. 1979 Feb;73(2):175–198. doi: 10.1085/jgp.73.2.175. [DOI] [PMC free article] [PubMed] [Google Scholar]
- Roulet M. J., Mongo K. G., Vassort G., Ventura-Clapier R. The dependence of twitch relaxation on sodium ions and on internal Ca2+ stores in voltage clamped frog atrial fibres. Pflugers Arch. 1979 Apr 30;379(3):259–268. doi: 10.1007/BF00581430. [DOI] [PubMed] [Google Scholar]
- Sinclair A. J., Miller H. A., Harrison D. C. An electrooptical monitoring technique for heart cells in tissue culture. J Appl Physiol. 1970 Nov;29(5):747–749. doi: 10.1152/jappl.1970.29.5.747. [DOI] [PubMed] [Google Scholar]
- Sperelakis N., Shigenobu K. Changes in membrane properties of chick embryonic hearts during development. J Gen Physiol. 1972 Oct;60(4):430–453. doi: 10.1085/jgp.60.4.430. [DOI] [PMC free article] [PubMed] [Google Scholar]
- Sperelakis N., Shigenobu K. Changes in membrane properties of chick embryonic hearts during development. J Gen Physiol. 1972 Oct;60(4):430–453. doi: 10.1085/jgp.60.4.430. [DOI] [PMC free article] [PubMed] [Google Scholar]
- Thompson E. J., Wilson S. H., Schuette W. H., Whitehouse W. C., Nirenberg M. W. Measurement of the rate and velocity of movement by single heart cells in culture. Am J Cardiol. 1973 Aug;32(2):162–166. doi: 10.1016/s0002-9149(73)80115-8. [DOI] [PubMed] [Google Scholar]
- Vassalle M. The relationship among cardiac pacemakers. Overdrive suppression. Circ Res. 1977 Sep;41(3):269–277. doi: 10.1161/01.res.41.3.269. [DOI] [PubMed] [Google Scholar]