Abstract
Band 3, the major intrinsic protein of the human erythrocyte membrane, was specifically labeled with the covalent fluorescent probe eosin isothiocyanate. The lateral mobility of labeled band 3 in the plane of the membrane under various conditions of ionic strength and temperature was examined by using the fluorescence photobleaching recovery technique. Low temperature (21°C) and high ionic strength (46 mM NaPO4) favored immobilization of band 3(10% mobile) as well as slow diffusion of the mobile fraction (diffusion coefficient D = 4 × 10-11 cm2sec-1). Increasing temperature (37°C) and decreasing ionic strength (13 mM NaPO4) led to an increase in the fraction of mobile band 3(90% mobile) and a reversible increase in the diffusion rate of the mobile fraction (D = 200 × 10-11 cm2sec-1). The increase in the fraction of mobile band 3 was markedly dissociated, however, from the increase in the diffusion rate of the mobile fraction. Thus, the fraction of mobile band 3 always increased at higher ionic strength and lower temperature than the ionic strength and temperature at which the diffusion rate increased. This dissociation was manifested kinetically on prolonged incubation of ghosts at constant ionic strength and temperature: the diffusion rate of the mobile fraction increased slowly at first and much more rapidly after the initial lag period, whereas the fraction of mobile band 3 increased almost immediately to 90% and remained maximal for the duration of the experiment. Further, changes in diffusion rate with temperature were promptly and totally reversible, whereas increases in the mobile fraction were only slowly and partially reversible. These effects were shown not to be due to complete dissociation of spectrin, the major protein of the erythrocyte cytoskeleton, from the membrane. This evidence suggests control of band 3 lateral mobility by at least two separate processes. The process that determines the diffusion coefficient of the mobile band 3 is completely reversible, and it probably involves a metastable state of cytoskeleton structure intermediate between tight binding to the membrane and complete dissociation from it.
Keywords: lateral diffusion, spectrin
Full text
PDF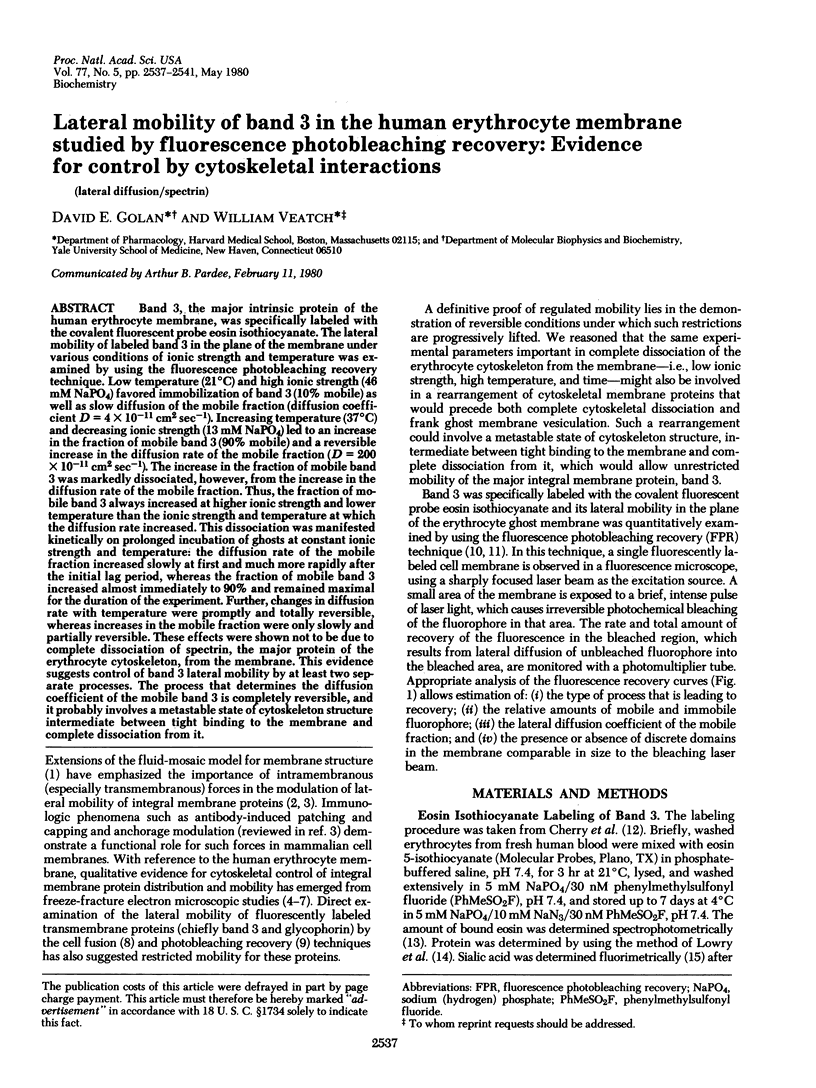
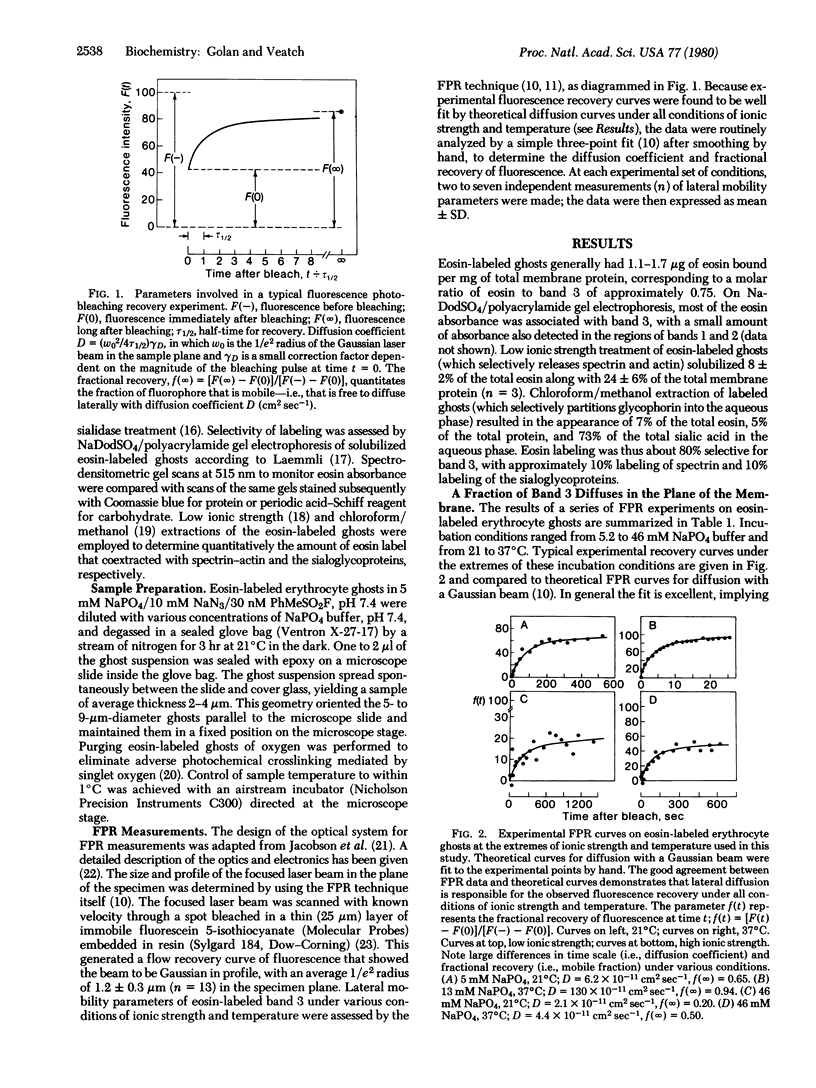
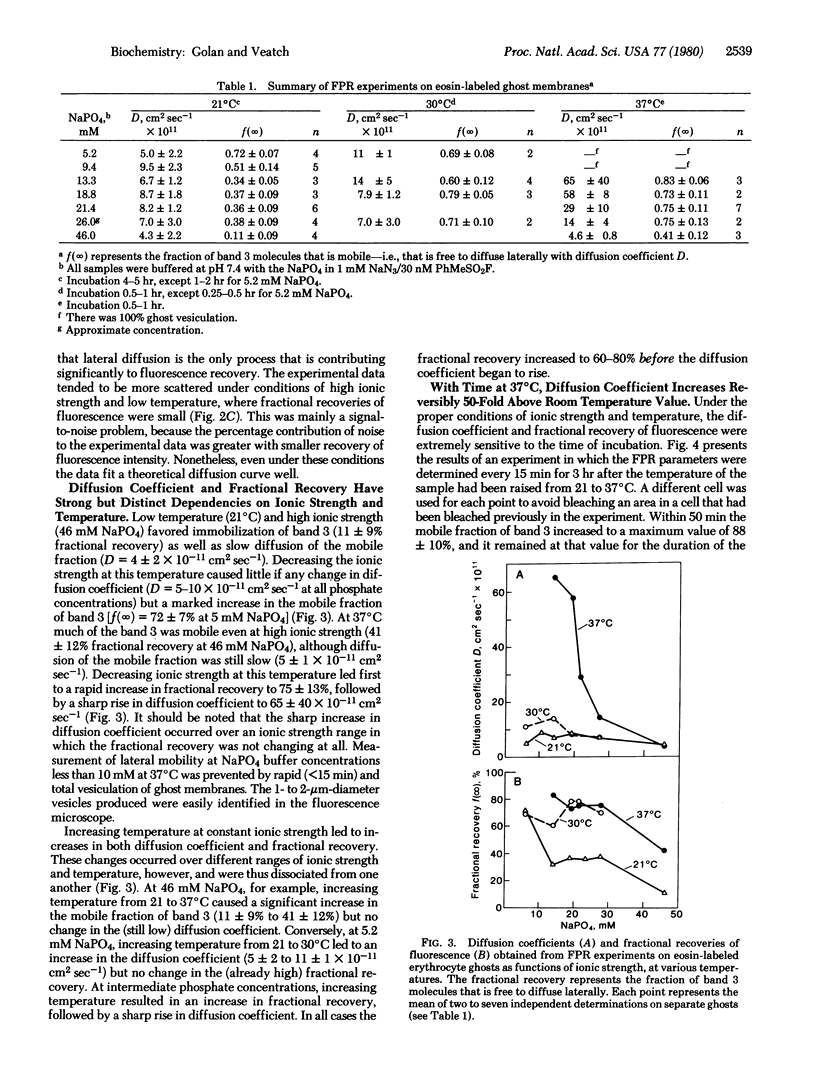
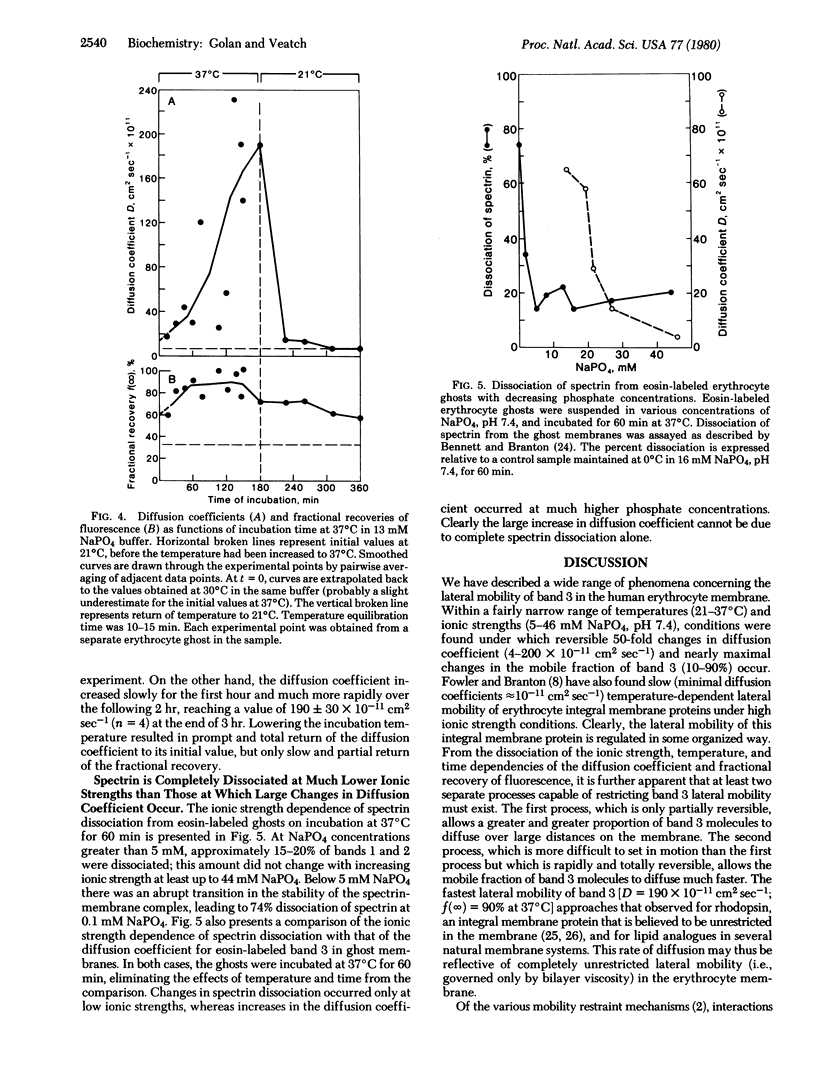
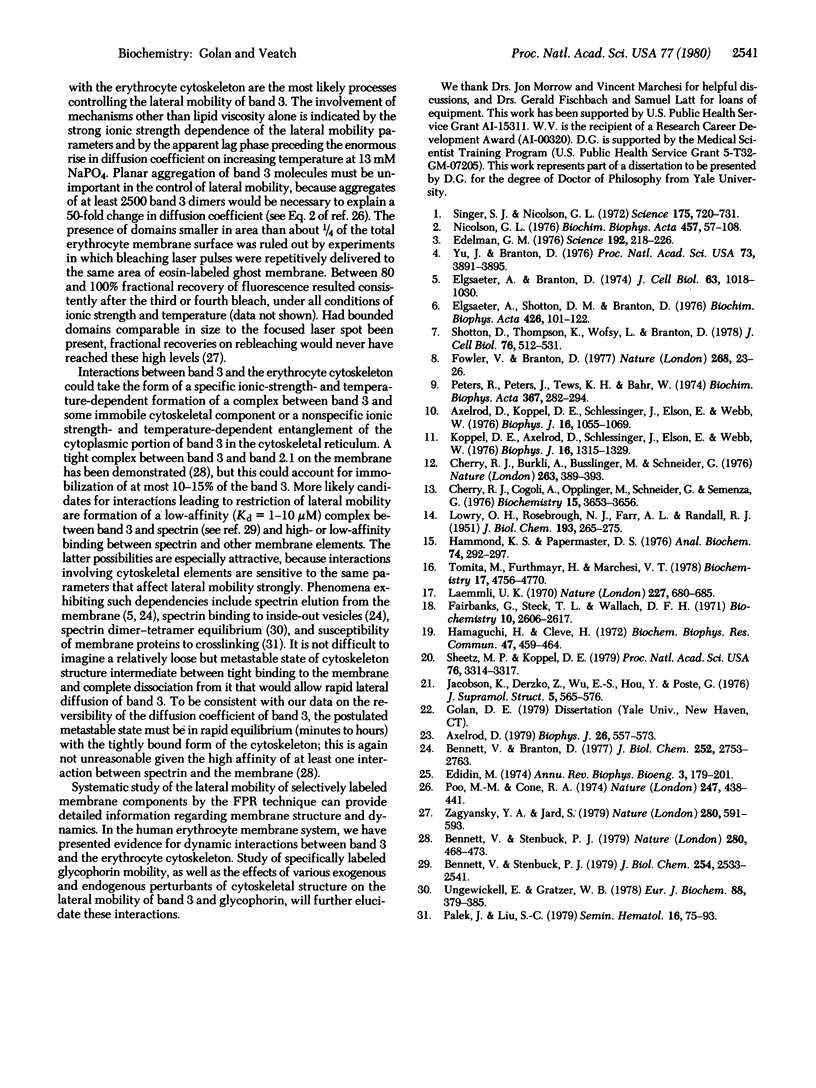
Selected References
These references are in PubMed. This may not be the complete list of references from this article.
- Axelrod D. Carbocyanine dye orientation in red cell membrane studied by microscopic fluorescence polarization. Biophys J. 1979 Jun;26(3):557–573. doi: 10.1016/S0006-3495(79)85271-6. [DOI] [PMC free article] [PubMed] [Google Scholar]
- Axelrod D., Koppel D. E., Schlessinger J., Elson E., Webb W. W. Mobility measurement by analysis of fluorescence photobleaching recovery kinetics. Biophys J. 1976 Sep;16(9):1055–1069. doi: 10.1016/S0006-3495(76)85755-4. [DOI] [PMC free article] [PubMed] [Google Scholar]
- Bennett V., Branton D. Selective association of spectrin with the cytoplasmic surface of human erythrocyte plasma membranes. Quantitative determination with purified (32P)spectrin. J Biol Chem. 1977 Apr 25;252(8):2753–2763. [PubMed] [Google Scholar]
- Bennett V., Stenbuck P. J. Identification and partial purification of ankyrin, the high affinity membrane attachment site for human erythrocyte spectrin. J Biol Chem. 1979 Apr 10;254(7):2533–2541. [PubMed] [Google Scholar]
- Bennett V., Stenbuck P. J. The membrane attachment protein for spectrin is associated with band 3 in human erythrocyte membranes. Nature. 1979 Aug 9;280(5722):468–473. doi: 10.1038/280468a0. [DOI] [PubMed] [Google Scholar]
- Cherry R. J., Bürkli A., Busslinger M., Schneider G., Parish G. R. Rotational diffusion of band 3 proteins in the human erythrocyte membrane. Nature. 1976 Sep 30;263(5576):389–393. doi: 10.1038/263389a0. [DOI] [PubMed] [Google Scholar]
- Cherry R. J., Cogoli A., Oppliger M., Schneider G., Semenza G. A spectroscopic technique for measuring slow rotational diffusion of macromolecules. 1: Preparation and properties of a triplet probe. Biochemistry. 1976 Aug 24;15(17):3653–3656. doi: 10.1021/bi00662a001. [DOI] [PubMed] [Google Scholar]
- Edelman G. M. Surface modulation in cell recognition and cell growth. Science. 1976 Apr 16;192(4236):218–226. doi: 10.1126/science.769162. [DOI] [PubMed] [Google Scholar]
- Edidin M. Rotational and translational diffusion in membranes. Annu Rev Biophys Bioeng. 1974;3(0):179–201. doi: 10.1146/annurev.bb.03.060174.001143. [DOI] [PubMed] [Google Scholar]
- Elgsaeter A., Branton D. Intramembrane particle aggregation in erythrocyte ghosts. I. The effects of protein removal. J Cell Biol. 1974 Dec;63(3):1018–1036. doi: 10.1083/jcb.63.3.1018. [DOI] [PMC free article] [PubMed] [Google Scholar]
- Elgsaeter A., Shotton D. M., Branton D. Intramembrane particle aggregation in erythrocyte ghosts. II. The influence of spectrin aggregation. Biochim Biophys Acta. 1976 Feb 19;426(1):101–122. doi: 10.1016/0005-2736(76)90433-8. [DOI] [PubMed] [Google Scholar]
- Fairbanks G., Steck T. L., Wallach D. F. Electrophoretic analysis of the major polypeptides of the human erythrocyte membrane. Biochemistry. 1971 Jun 22;10(13):2606–2617. doi: 10.1021/bi00789a030. [DOI] [PubMed] [Google Scholar]
- Fowler V., Branton D. Lateral mobility of human erythrocyte integral membrane proteins. Nature. 1977 Jul 7;268(5615):23–26. doi: 10.1038/268023a0. [DOI] [PubMed] [Google Scholar]
- Hamaguchi H., Cleve H. Solubilization and comparative analysis of mammalian erythrocyte membrane glycoproteins. Biochem Biophys Res Commun. 1972 Apr 28;47(2):459–464. doi: 10.1016/0006-291x(72)90736-x. [DOI] [PubMed] [Google Scholar]
- Hammond K. S., Papermaster D. S. Fluorometric assay of sialic acid in the picomole range: a modification of the thiobarbituric acid assay. Anal Biochem. 1976 Aug;74(2):292–297. doi: 10.1016/0003-2697(76)90210-4. [DOI] [PubMed] [Google Scholar]
- Jacobson K., Derzko Z., Wu E. S., Hou Y., Poste G. Measurement of the lateral mobility of cell surface components in single, living cells by fluorescence recovery after photobleaching. J Supramol Struct. 1976;5(4):565(417)–576(428). doi: 10.1002/jss.400050411. [DOI] [PubMed] [Google Scholar]
- Koppel D. E., Axelrod D., Schlessinger J., Elson E. L., Webb W. W. Dynamics of fluorescence marker concentration as a probe of mobility. Biophys J. 1976 Nov;16(11):1315–1329. doi: 10.1016/S0006-3495(76)85776-1. [DOI] [PMC free article] [PubMed] [Google Scholar]
- LOWRY O. H., ROSEBROUGH N. J., FARR A. L., RANDALL R. J. Protein measurement with the Folin phenol reagent. J Biol Chem. 1951 Nov;193(1):265–275. [PubMed] [Google Scholar]
- Laemmli U. K. Cleavage of structural proteins during the assembly of the head of bacteriophage T4. Nature. 1970 Aug 15;227(5259):680–685. doi: 10.1038/227680a0. [DOI] [PubMed] [Google Scholar]
- Nicolson G. L. Transmembrane control of the receptors on normal and tumor cells. I. Cytoplasmic influence over surface components. Biochim Biophys Acta. 1976 Apr 13;457(1):57–108. doi: 10.1016/0304-4157(76)90014-9. [DOI] [PubMed] [Google Scholar]
- Palek J., Liu S. C. Dependence of spectrin organization in red blood cell membranes on cell metabolism: implications for control of red cell shape, deformability, and surface area. Semin Hematol. 1979 Jan;16(1):75–93. [PubMed] [Google Scholar]
- Peters R., Peters J., Tews K. H., Bähr W. A microfluorimetric study of translational diffusion in erythrocyte membranes. Biochim Biophys Acta. 1974 Nov 15;367(3):282–294. doi: 10.1016/0005-2736(74)90085-6. [DOI] [PubMed] [Google Scholar]
- Poo M., Cone R. A. Lateral diffusion of rhodopsin in the photoreceptor membrane. Nature. 1974 Feb 15;247(5441):438–441. doi: 10.1038/247438a0. [DOI] [PubMed] [Google Scholar]
- Sheetz M. P., Koppel D. E. Membrane damage caused by irradiation of fluorescent concanavalin A. Proc Natl Acad Sci U S A. 1979 Jul;76(7):3314–3317. doi: 10.1073/pnas.76.7.3314. [DOI] [PMC free article] [PubMed] [Google Scholar]
- Shotton D., Thompson K., Wofsy L., Branton D. Appearance and distribution of surface proteins of the human erythrocyte membrane. An electron microscope and immunochemical labeling study. J Cell Biol. 1978 Feb;76(2):512–531. doi: 10.1083/jcb.76.2.512. [DOI] [PMC free article] [PubMed] [Google Scholar]
- Singer S. J., Nicolson G. L. The fluid mosaic model of the structure of cell membranes. Science. 1972 Feb 18;175(4023):720–731. doi: 10.1126/science.175.4023.720. [DOI] [PubMed] [Google Scholar]
- Tomita M., Furthmayr H., Marchesi V. T. Primary structure of human erythrocyte glycophorin A. Isolation and characterization of peptides and complete amino acid sequence. Biochemistry. 1978 Oct 31;17(22):4756–4770. doi: 10.1021/bi00615a025. [DOI] [PubMed] [Google Scholar]
- Ungewickell E., Gratzer W. Self-association of human spectrin. A thermodynamic and kinetic study. Eur J Biochem. 1978 Aug 1;88(2):379–385. doi: 10.1111/j.1432-1033.1978.tb12459.x. [DOI] [PubMed] [Google Scholar]
- Yu J., Branton D. Reconstitution of intramembrane particles in recombinants of erythrocyte protein band 3 and lipid: effects of spectrin-actin association. Proc Natl Acad Sci U S A. 1976 Nov;73(11):3891–3895. doi: 10.1073/pnas.73.11.3891. [DOI] [PMC free article] [PubMed] [Google Scholar]
- Zagyansky Y. A., Jard S. Does lectin-receptor complex formation produce zones of restricted mobility within the membrane? Nature. 1979 Aug 16;280(5723):591–593. doi: 10.1038/280591a0. [DOI] [PubMed] [Google Scholar]