Abstract
Regulation of D-glucose transport in the porcine kidney epithelial cell line LLC-PK1 was examined. To identify the sodium-coupled glucose transporter (SGLT), we cloned and sequenced several partial cDNAs homologous to SGLT1 from rabbit small intestine (M. A. Hediger, M. J. Coady, T. S. Ikeda, and E. M. Wright, Nature (London) 330:379-381, 1987). The extensive homology of the two sequences leads us to suggest that the high-affinity SGLT expressed by LLC-PK1 cells is SGLT1. SGLT1 mRNA levels were highest when the D-glucose concentration in the culture medium was 5 to 10 mM. Addition of D-mannose or D-fructose, but not D-galactose, in the presence of 5 mM D-glucose suppressed SGLT1 mRNA levels. SGLT1 activity, measured by methyl alpha-D-glucopyranoside uptake, paralleled message levels except in cultures containing D-galactose. Therefore, SGLT1 gene expression may respond either to the cellular energy status or to the concentration of a hexose metabolite(s). By isolating several cDNAs homologous to rat GLUT-1, we identified the facilitated glucose transporter in LLC-PK1 cells as the erythroid/brain type GLUT-1. High-stringency hybridization of a single mRNA transcript to the rat GLUT-1 cDNA probe and failure to observe additional transcripts hybridizing either to GLUT-1 or to GLUT-2 probes at low stringency provide evidence that GLUT-1 is the major facilitated glucose transporter in this cell line. LLC-PK1 GLUT-1 mRNAs were highest at medium D-glucose concentrations of less than or equal to 2 mM. D-Fructose, D-mannose, and to a lesser extent D-galactose all suppressed GLUT-1 mRNA levels. Since the pattern of SGLT1 and GLUT-1 expression differed, particularly in low D-glucose or in the presence of D-galactose, we suggest that the two transporters are regulated independently.
Full text
PDF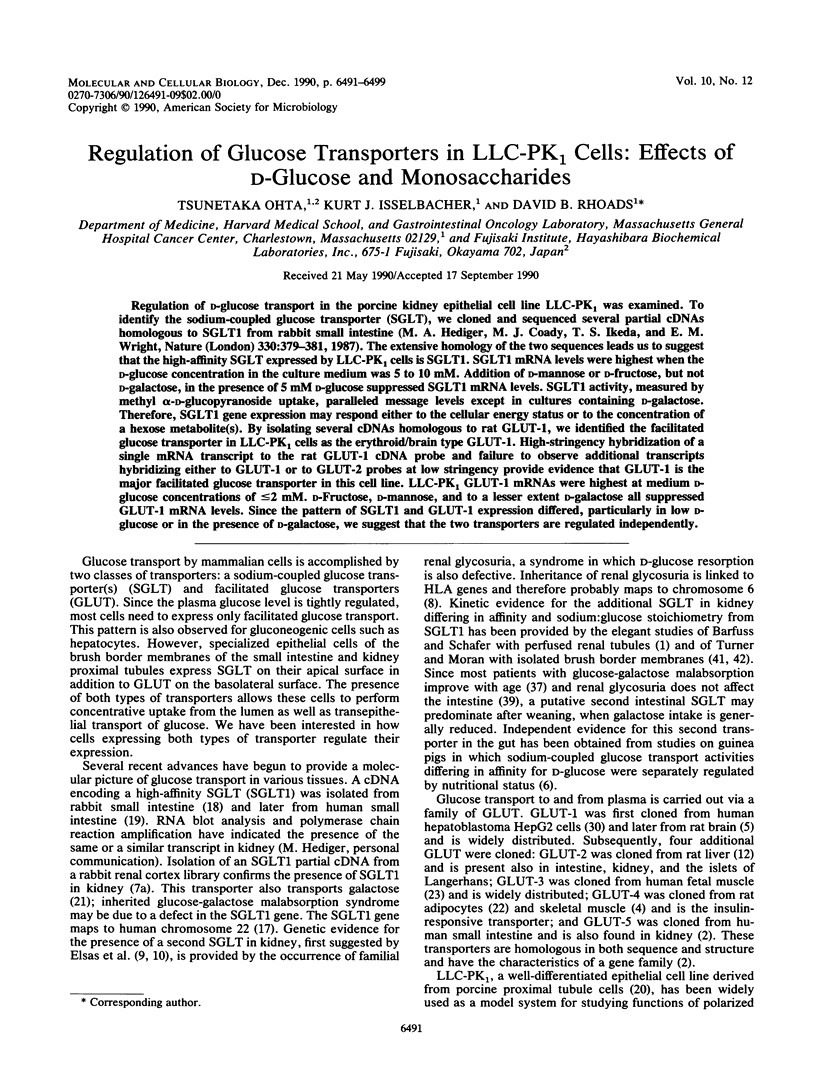
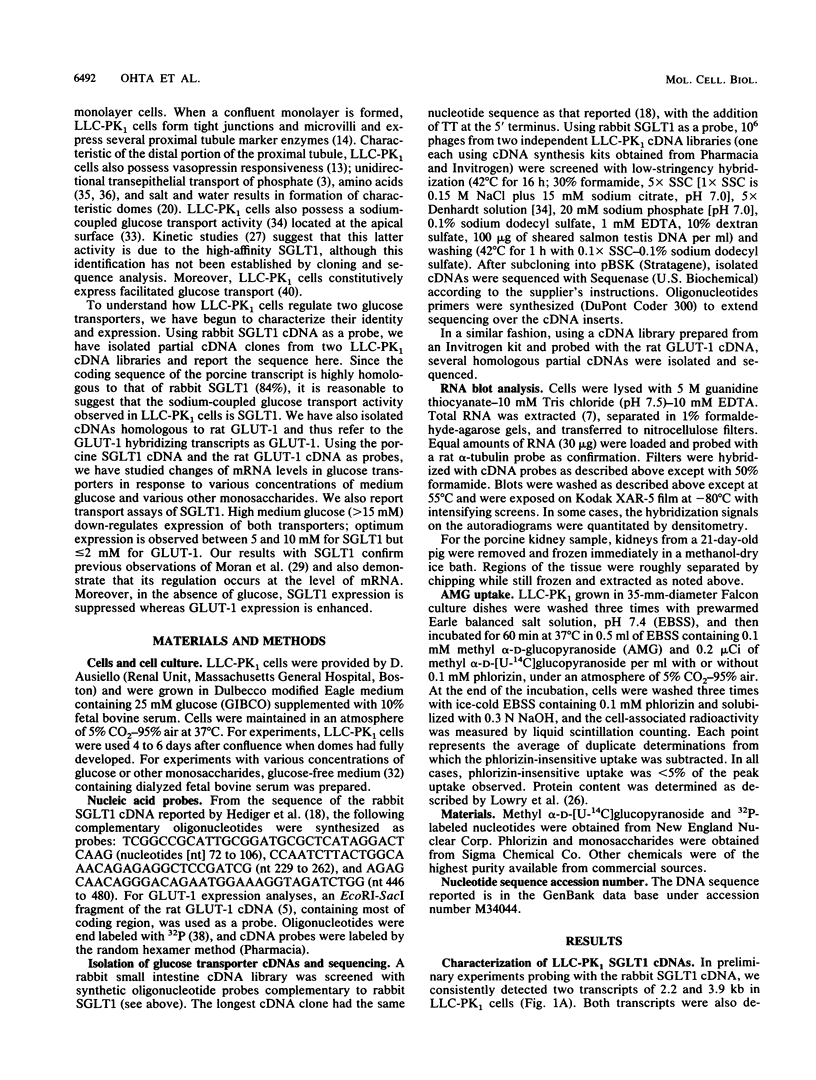
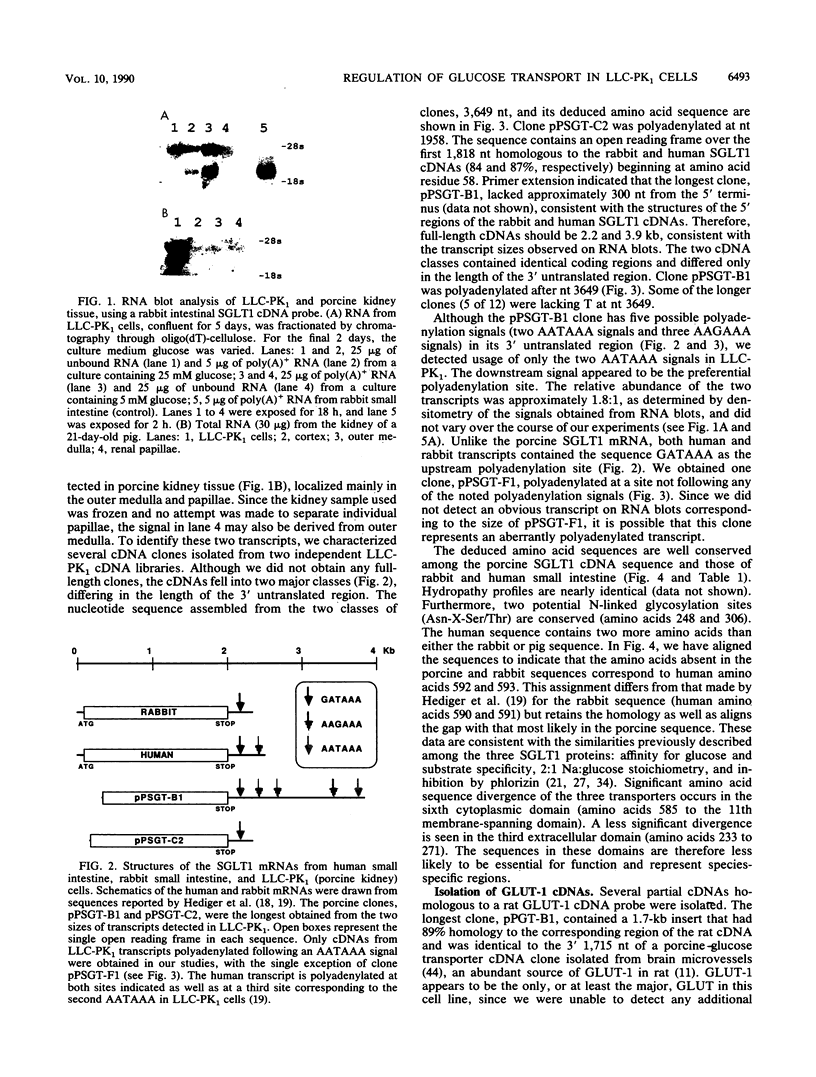
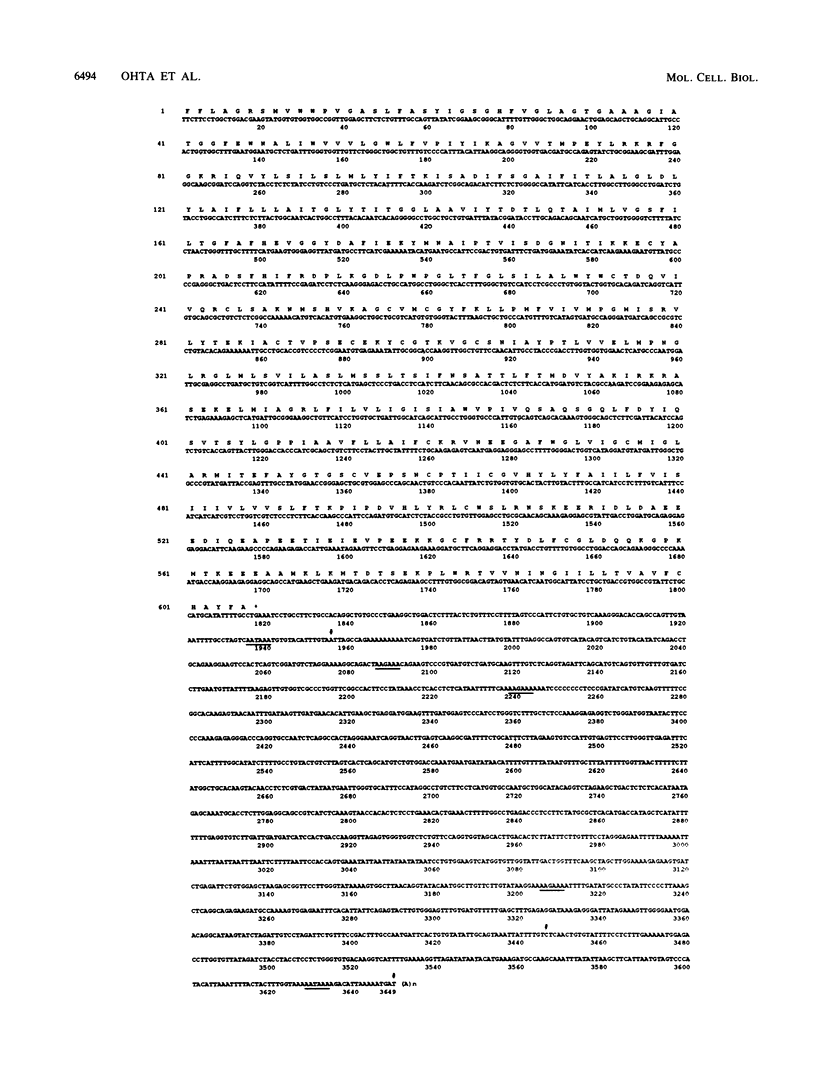
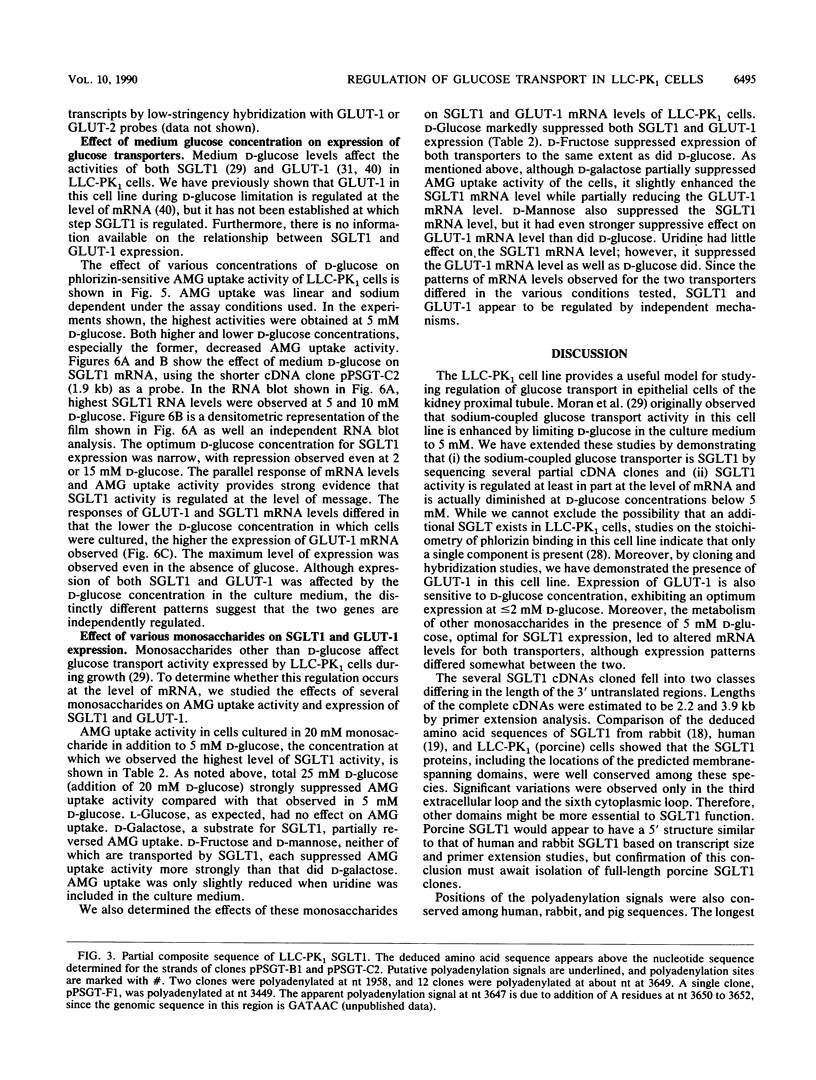
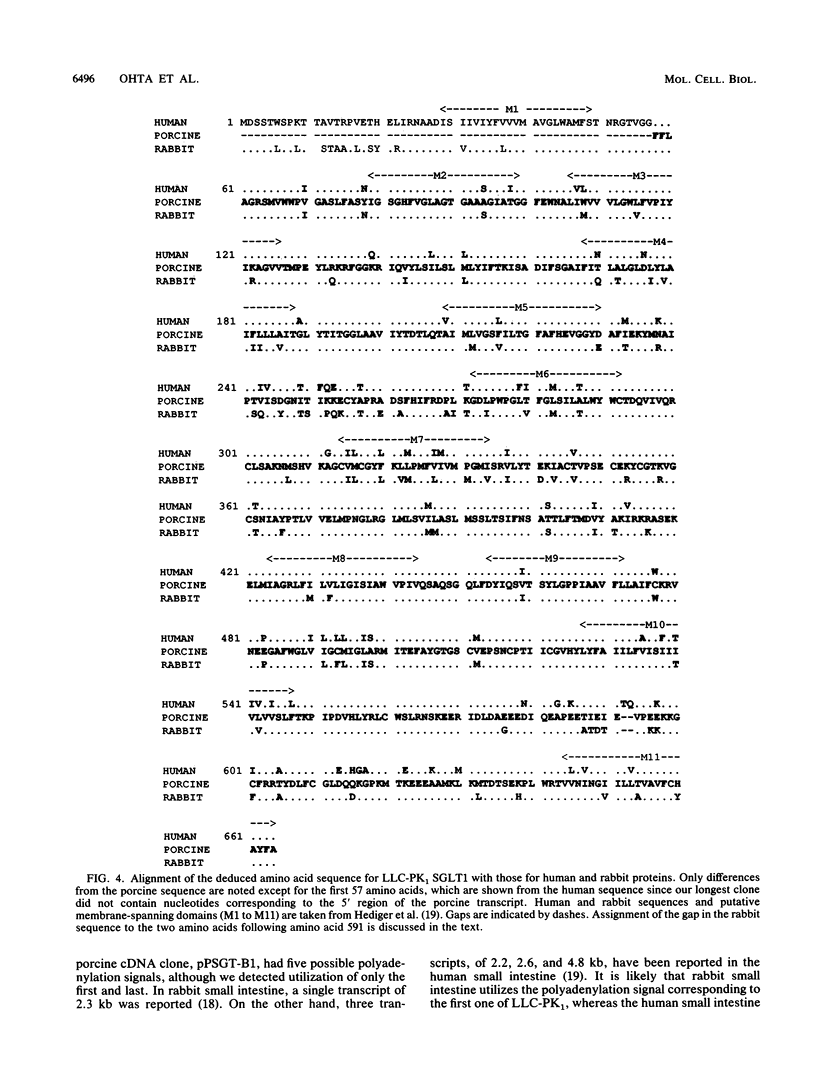
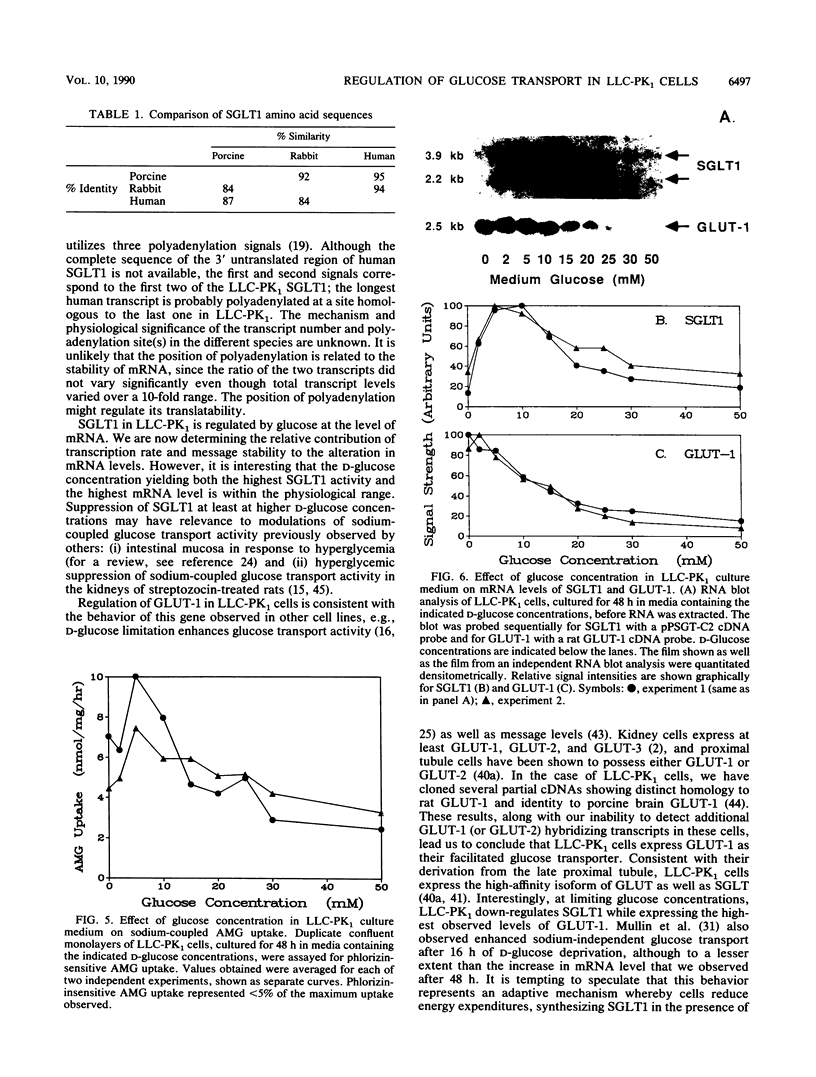
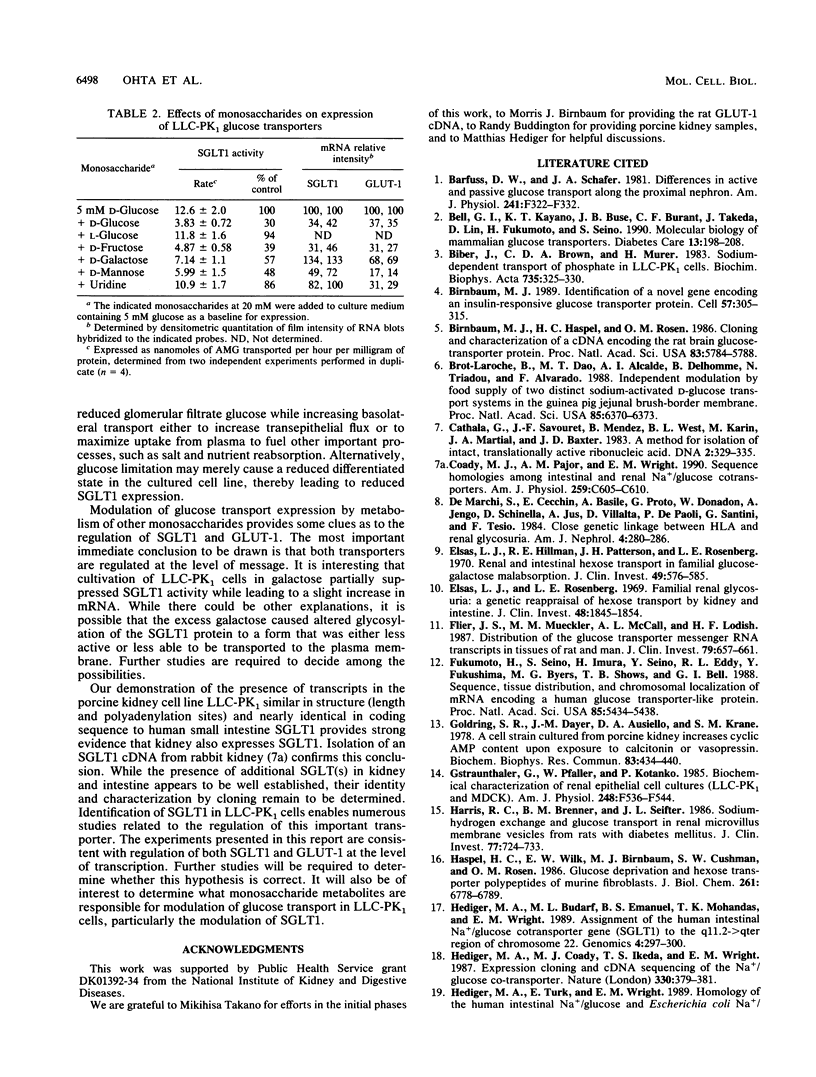
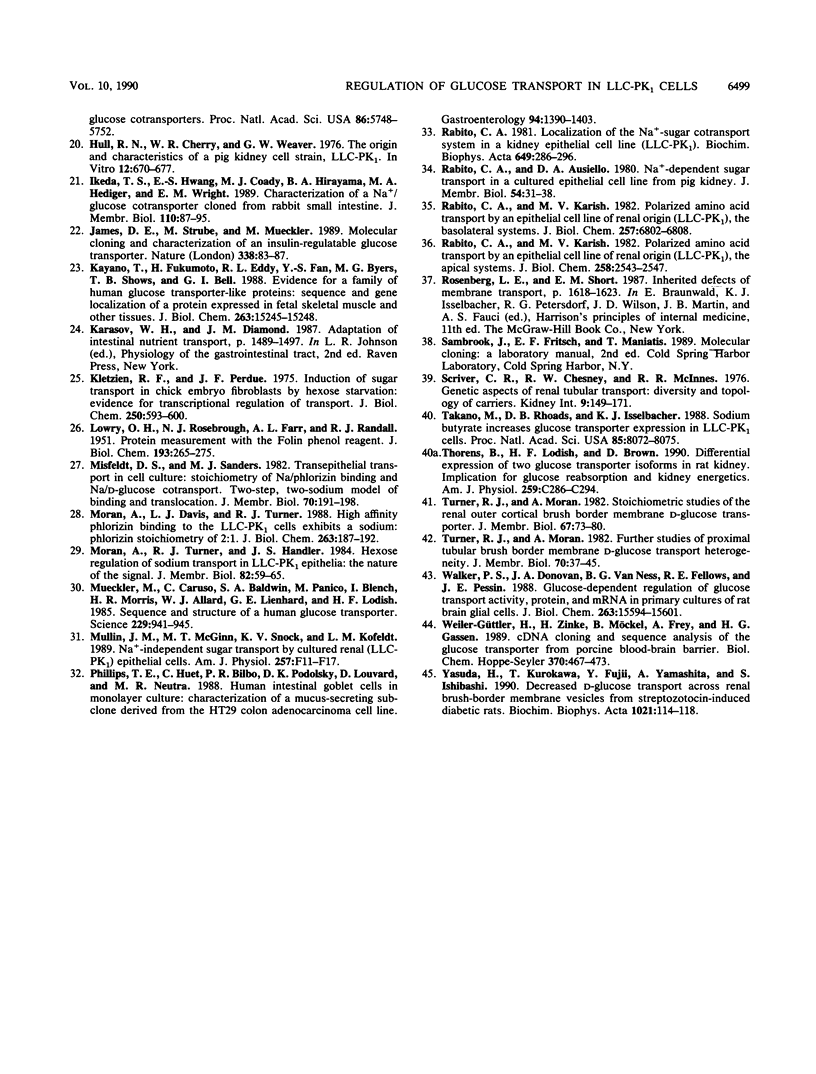
Images in this article
Selected References
These references are in PubMed. This may not be the complete list of references from this article.
- Barfuss D. W., Schafer J. A. Differences in active and passive glucose transport along the proximal nephron. Am J Physiol. 1981 Sep;241(3):F322–F332. doi: 10.1152/ajprenal.1981.241.3.F322. [DOI] [PubMed] [Google Scholar]
- Bell G. I., Kayano T., Buse J. B., Burant C. F., Takeda J., Lin D., Fukumoto H., Seino S. Molecular biology of mammalian glucose transporters. Diabetes Care. 1990 Mar;13(3):198–208. doi: 10.2337/diacare.13.3.198. [DOI] [PubMed] [Google Scholar]
- Biber J., Brown C. D., Murer H. Sodium-dependent transport of phosphate in LLC-PK1 cells. Biochim Biophys Acta. 1983 Nov 23;735(3):325–330. doi: 10.1016/0005-2736(83)90145-1. [DOI] [PubMed] [Google Scholar]
- Birnbaum M. J., Haspel H. C., Rosen O. M. Cloning and characterization of a cDNA encoding the rat brain glucose-transporter protein. Proc Natl Acad Sci U S A. 1986 Aug;83(16):5784–5788. doi: 10.1073/pnas.83.16.5784. [DOI] [PMC free article] [PubMed] [Google Scholar]
- Birnbaum M. J. Identification of a novel gene encoding an insulin-responsive glucose transporter protein. Cell. 1989 Apr 21;57(2):305–315. doi: 10.1016/0092-8674(89)90968-9. [DOI] [PubMed] [Google Scholar]
- Brot-Laroche E., Dao M. T., Alcalde A. I., Delhomme B., Triadou N., Alvarado F. Independent modulation by food supply of two distinct sodium-activated D-glucose transport systems in the guinea pig jejunal brush-border membrane. Proc Natl Acad Sci U S A. 1988 Sep;85(17):6370–6373. doi: 10.1073/pnas.85.17.6370. [DOI] [PMC free article] [PubMed] [Google Scholar]
- Cathala G., Savouret J. F., Mendez B., West B. L., Karin M., Martial J. A., Baxter J. D. A method for isolation of intact, translationally active ribonucleic acid. DNA. 1983;2(4):329–335. doi: 10.1089/dna.1983.2.329. [DOI] [PubMed] [Google Scholar]
- Coady M. J., Pajor A. M., Wright E. M. Sequence homologies among intestinal and renal Na+/glucose cotransporters. Am J Physiol. 1990 Oct;259(4 Pt 1):C605–C610. doi: 10.1152/ajpcell.1990.259.4.C605. [DOI] [PubMed] [Google Scholar]
- De Marchi S., Cecchin E., Basile A., Proto G., Donadon W., Jengo A., Schinella D., Jus A., Villalta D., De Paoli P. Close genetic linkage between HLA and renal glycosuria. Am J Nephrol. 1984;4(5):280–286. doi: 10.1159/000166826. [DOI] [PubMed] [Google Scholar]
- Elsas L. J., Hillman R. E., Patterson J. H., Rosenberg L. E. Renal and intestinal hexose transport in familial glucose-galactose malabsorption. J Clin Invest. 1970 Mar;49(3):576–585. doi: 10.1172/JCI106268. [DOI] [PMC free article] [PubMed] [Google Scholar]
- Elsas L. J., Rosenberg L. E. Familial renal glycosuria: a genetic reappraisal of hexose transport by kidney and intestine. J Clin Invest. 1969 Oct;48(10):1845–1854. doi: 10.1172/JCI106150. [DOI] [PMC free article] [PubMed] [Google Scholar]
- Flier J. S., Mueckler M., McCall A. L., Lodish H. F. Distribution of glucose transporter messenger RNA transcripts in tissues of rat and man. J Clin Invest. 1987 Feb;79(2):657–661. doi: 10.1172/JCI112864. [DOI] [PMC free article] [PubMed] [Google Scholar]
- Fukumoto H., Seino S., Imura H., Seino Y., Eddy R. L., Fukushima Y., Byers M. G., Shows T. B., Bell G. I. Sequence, tissue distribution, and chromosomal localization of mRNA encoding a human glucose transporter-like protein. Proc Natl Acad Sci U S A. 1988 Aug;85(15):5434–5438. doi: 10.1073/pnas.85.15.5434. [DOI] [PMC free article] [PubMed] [Google Scholar]
- Goldring S. R., Dayer J. M., Ausiello D. A., Krane S. M. A cell strain cultured from porcine kidney increases cyclic AMP content upon exposure to calcitonin or vasopressin. Biochem Biophys Res Commun. 1978 Jul 28;83(2):434–440. doi: 10.1016/0006-291x(78)91009-4. [DOI] [PubMed] [Google Scholar]
- Gstraunthaler G., Pfaller W., Kotanko P. Biochemical characterization of renal epithelial cell cultures (LLC-PK1 and MDCK). Am J Physiol. 1985 Apr;248(4 Pt 2):F536–F544. doi: 10.1152/ajprenal.1985.248.4.F536. [DOI] [PubMed] [Google Scholar]
- Harris R. C., Brenner B. M., Seifter J. L. Sodium-hydrogen exchange and glucose transport in renal microvillus membrane vesicles from rats with diabetes mellitus. J Clin Invest. 1986 Mar;77(3):724–733. doi: 10.1172/JCI112367. [DOI] [PMC free article] [PubMed] [Google Scholar]
- Haspel H. C., Wilk E. W., Birnbaum M. J., Cushman S. W., Rosen O. M. Glucose deprivation and hexose transporter polypeptides of murine fibroblasts. J Biol Chem. 1986 May 25;261(15):6778–6789. [PubMed] [Google Scholar]
- Hediger M. A., Budarf M. L., Emanuel B. S., Mohandas T. K., Wright E. M. Assignment of the human intestinal Na+/glucose cotransporter gene (SGLT1) to the q11.2----qter region of chromosome 22. Genomics. 1989 Apr;4(3):297–300. doi: 10.1016/0888-7543(89)90333-9. [DOI] [PubMed] [Google Scholar]
- Hediger M. A., Coady M. J., Ikeda T. S., Wright E. M. Expression cloning and cDNA sequencing of the Na+/glucose co-transporter. 1987 Nov 26-Dec 2Nature. 330(6146):379–381. doi: 10.1038/330379a0. [DOI] [PubMed] [Google Scholar]
- Hediger M. A., Turk E., Wright E. M. Homology of the human intestinal Na+/glucose and Escherichia coli Na+/proline cotransporters. Proc Natl Acad Sci U S A. 1989 Aug;86(15):5748–5752. doi: 10.1073/pnas.86.15.5748. [DOI] [PMC free article] [PubMed] [Google Scholar]
- Hull R. N., Cherry W. R., Weaver G. W. The origin and characteristics of a pig kidney cell strain, LLC-PK. In Vitro. 1976 Oct;12(10):670–677. doi: 10.1007/BF02797469. [DOI] [PubMed] [Google Scholar]
- Ikeda T. S., Hwang E. S., Coady M. J., Hirayama B. A., Hediger M. A., Wright E. M. Characterization of a Na+/glucose cotransporter cloned from rabbit small intestine. J Membr Biol. 1989 Aug;110(1):87–95. doi: 10.1007/BF01870995. [DOI] [PubMed] [Google Scholar]
- James D. E., Strube M., Mueckler M. Molecular cloning and characterization of an insulin-regulatable glucose transporter. Nature. 1989 Mar 2;338(6210):83–87. doi: 10.1038/338083a0. [DOI] [PubMed] [Google Scholar]
- Kayano T., Fukumoto H., Eddy R. L., Fan Y. S., Byers M. G., Shows T. B., Bell G. I. Evidence for a family of human glucose transporter-like proteins. Sequence and gene localization of a protein expressed in fetal skeletal muscle and other tissues. J Biol Chem. 1988 Oct 25;263(30):15245–15248. [PubMed] [Google Scholar]
- Kletzien R. F., Perdue J. F. Induction of sugar transport in chick embryo fibroblasts by hexose starvation. Evidence for transcriptional regulation of transport. J Biol Chem. 1975 Jan 25;250(2):593–600. [PubMed] [Google Scholar]
- LOWRY O. H., ROSEBROUGH N. J., FARR A. L., RANDALL R. J. Protein measurement with the Folin phenol reagent. J Biol Chem. 1951 Nov;193(1):265–275. [PubMed] [Google Scholar]
- Misfeldt D. S., Sanders M. J. Transepithelial transport in cell culture: stoichiometry of Na/phlorizin binding and Na/D-glucose cotransport. A two-step, two sodium model of binding and translocation. J Membr Biol. 1982;70(3):191–198. doi: 10.1007/BF01870562. [DOI] [PubMed] [Google Scholar]
- Moran A., Davis L. J., Turner R. J. High affinity phlorizin binding to the LLC-PK1 cells exhibits a sodium:phlorizin stoichiometry of 2:1. J Biol Chem. 1988 Jan 5;263(1):187–192. [PubMed] [Google Scholar]
- Moran A., Turner R. J., Handler J. S. Hexose regulation of sodium-hexose transport in LLC-PK1 epithelia: the nature of the signal. J Membr Biol. 1984;82(1):59–65. doi: 10.1007/BF01870732. [DOI] [PubMed] [Google Scholar]
- Mueckler M., Caruso C., Baldwin S. A., Panico M., Blench I., Morris H. R., Allard W. J., Lienhard G. E., Lodish H. F. Sequence and structure of a human glucose transporter. Science. 1985 Sep 6;229(4717):941–945. doi: 10.1126/science.3839598. [DOI] [PubMed] [Google Scholar]
- Mullin J. M., McGinn M. T., Snock K. V., Kofeldt L. M. Na+-independent sugar transport by cultured renal (LLC-PK1) epithelial cells. Am J Physiol. 1989 Jul;257(1 Pt 2):F11–F17. doi: 10.1152/ajprenal.1989.257.1.F11. [DOI] [PubMed] [Google Scholar]
- Phillips T. E., Huet C., Bilbo P. R., Podolsky D. K., Louvard D., Neutra M. R. Human intestinal goblet cells in monolayer culture: characterization of a mucus-secreting subclone derived from the HT29 colon adenocarcinoma cell line. Gastroenterology. 1988 Jun;94(6):1390–1403. doi: 10.1016/0016-5085(88)90678-6. [DOI] [PubMed] [Google Scholar]
- Rabito C. A., Ausiello D. A. Na+-dependent sugar transport in a cultured epithelial cell line from pig kidney. J Membr Biol. 1980;54(1):31–38. doi: 10.1007/BF01875374. [DOI] [PubMed] [Google Scholar]
- Rabito C. A., Karish M. V. Polarized amino acid transport by an epithelial cell line of renal origin (LLC-PK1). The apical systems. J Biol Chem. 1983 Feb 25;258(4):2543–2547. [PubMed] [Google Scholar]
- Rabito C. A., Karish M. V. Polarized amino acid transport by an epithelial cell line of renal origin (LLC-PK1). The basolateral systems. J Biol Chem. 1982 Jun 25;257(12):6802–6808. [PubMed] [Google Scholar]
- Rabito C. A. Localization of the Na+-sugar cotransport system in a kidney epithelial cell line (LLC PK1). Biochim Biophys Acta. 1981 Dec 7;649(2):286–296. doi: 10.1016/0005-2736(81)90417-x. [DOI] [PubMed] [Google Scholar]
- Scriver C. R., Chesney R. W., McInnes R. R. Genetic aspects of renal tubular transport: diversity and topology of carriers. Kidney Int. 1976 Feb;9(2):149–171. doi: 10.1038/ki.1976.18. [DOI] [PubMed] [Google Scholar]
- Takano M., Rhoads D. B., Isselbacher K. J. Sodium butyrate increases glucose transporter expression in LLC-PK1 cells. Proc Natl Acad Sci U S A. 1988 Nov;85(21):8072–8075. doi: 10.1073/pnas.85.21.8072. [DOI] [PMC free article] [PubMed] [Google Scholar]
- Thorens B., Lodish H. F., Brown D. Differential localization of two glucose transporter isoforms in rat kidney. Am J Physiol. 1990 Dec;259(6 Pt 1):C286–C294. doi: 10.1152/ajpcell.1990.259.2.C286. [DOI] [PubMed] [Google Scholar]
- Turner R. J., Moran A. Further studies of proximal tubular brush border membrane D-glucose transport heterogeneity. J Membr Biol. 1982;70(1):37–45. doi: 10.1007/BF01871587. [DOI] [PubMed] [Google Scholar]
- Turner R. J., Moran A. Stoichiometric studies of the renal outer cortical brush border membrane D-glucose transporter. J Membr Biol. 1982;67(1):73–80. doi: 10.1007/BF01868649. [DOI] [PubMed] [Google Scholar]
- Walker P. S., Donovan J. A., Van Ness B. G., Fellows R. E., Pessin J. E. Glucose-dependent regulation of glucose transport activity, protein, and mRNA in primary cultures of rat brain glial cells. J Biol Chem. 1988 Oct 25;263(30):15594–15601. [PubMed] [Google Scholar]
- Weiler-Güttler H., Zinke H., Möckel B., Frey A., Gassen H. G. cDNA cloning and sequence analysis of the glucose transporter from porcine blood-brain barrier. Biol Chem Hoppe Seyler. 1989 May;370(5):467–473. doi: 10.1515/bchm3.1989.370.1.467. [DOI] [PubMed] [Google Scholar]
- Yasuda H., Kurokawa T., Fujii Y., Yamashita A., Ishibashi S. Decreased D-glucose transport across renal brush-border membrane vesicles from streptozotocin-induced diabetic rats. Biochim Biophys Acta. 1990 Jan 29;1021(2):114–118. doi: 10.1016/0005-2736(90)90022-g. [DOI] [PubMed] [Google Scholar]