Abstract
There are two yeast enolase genes, designated ENO1 and ENO2, which are expressed differentially in vegetative cells grown on glucose and in cells grown on gluconeogenic carbon sources. ENO2 is induced more than 20-fold in cells grown on glucose, whereas ENO1 expression is similar in cells grown on glucose and in cells grown on gluconeogenic carbon sources. Sequences within the 5' flanking region of ENO2 which are required for glucose-dependent induction were identified by deletion mapping analysis. These studies were carried out by using a fused gene containing the ENO2 5' flanking sequences and the ENO1 coding sequences. This fused gene undergoes glucose-dependent induction and is expressed at the same level as the resident ENO2 gene in cells grown on glucose or gluconeogenic carbon sources. Expression of fused genes containing deletion mutations within the ENO2 5' flanking region was monitored after integration at the ENO1 locus of a strain carrying a deletion of the resident ENO1 coding sequences. This analysis showed that there are two upstream activation sites located immediately upstream and downstream from a position 461 base pairs upstream from the transcriptional initiation site. Either one of these upstream activation sites is sufficient for glucose-dependent induction and normal gene expression in the presence of gluconeogenic carbon sources. Deletion of both regulatory regions results in a complete loss of gene expression. The regulatory regions function normally in both orientations relative to the coding sequences. Mutant fused genes containing small deletions within the regulatory regions were constructed; these genes were expressed normally in gluconeogenic carbon sources but were not induced in the presence of glucose. Based on this analysis, ENO2 contains a cis-acting regulatory region which is required for gene expression and mediates glucose-dependent induction of gene expression.
Full text
PDF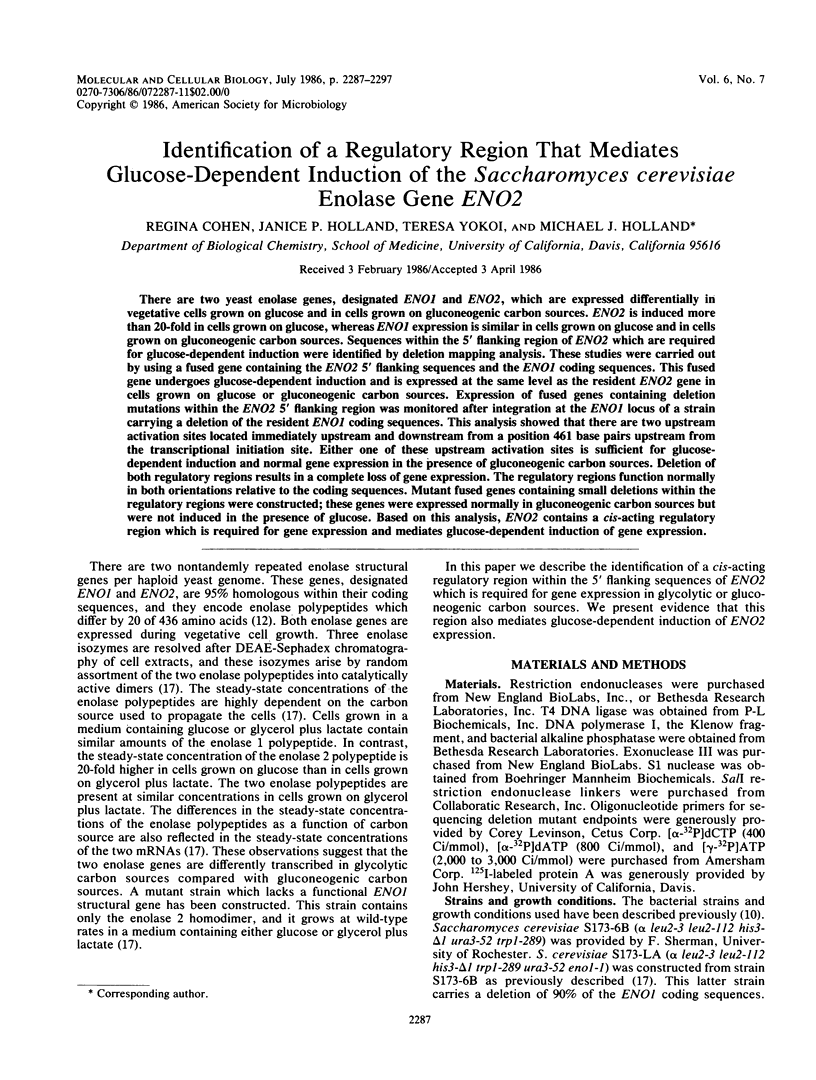
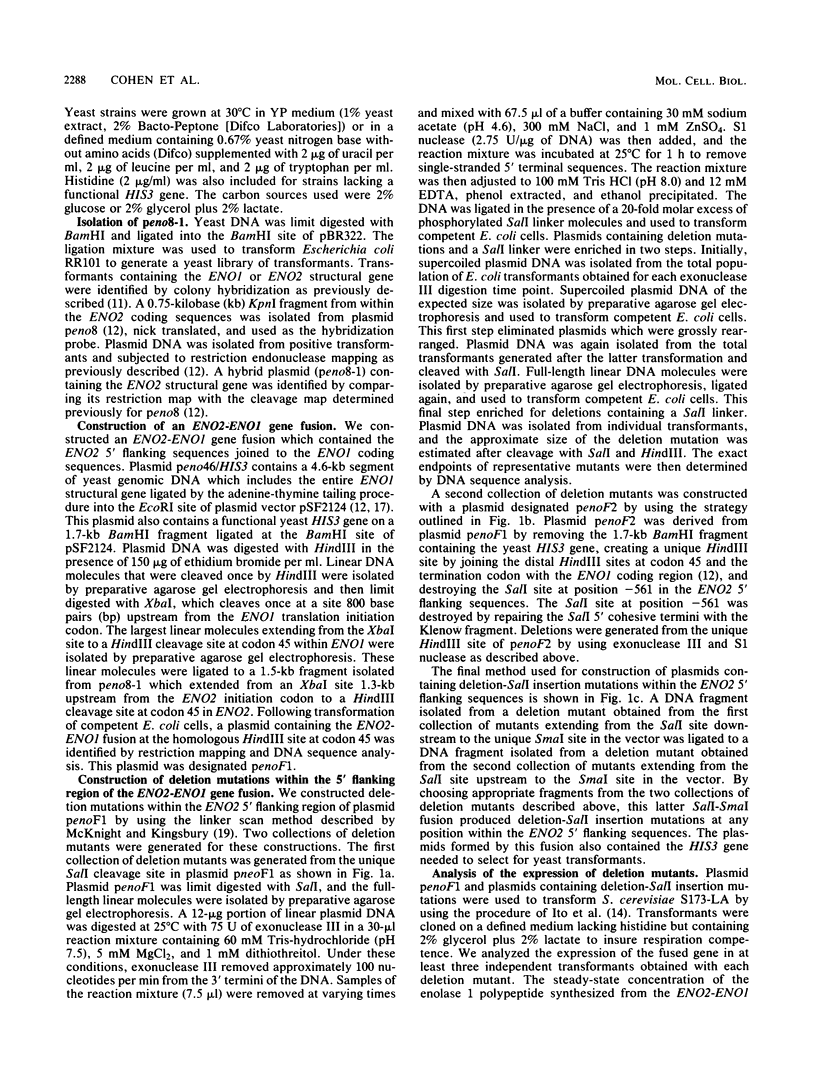
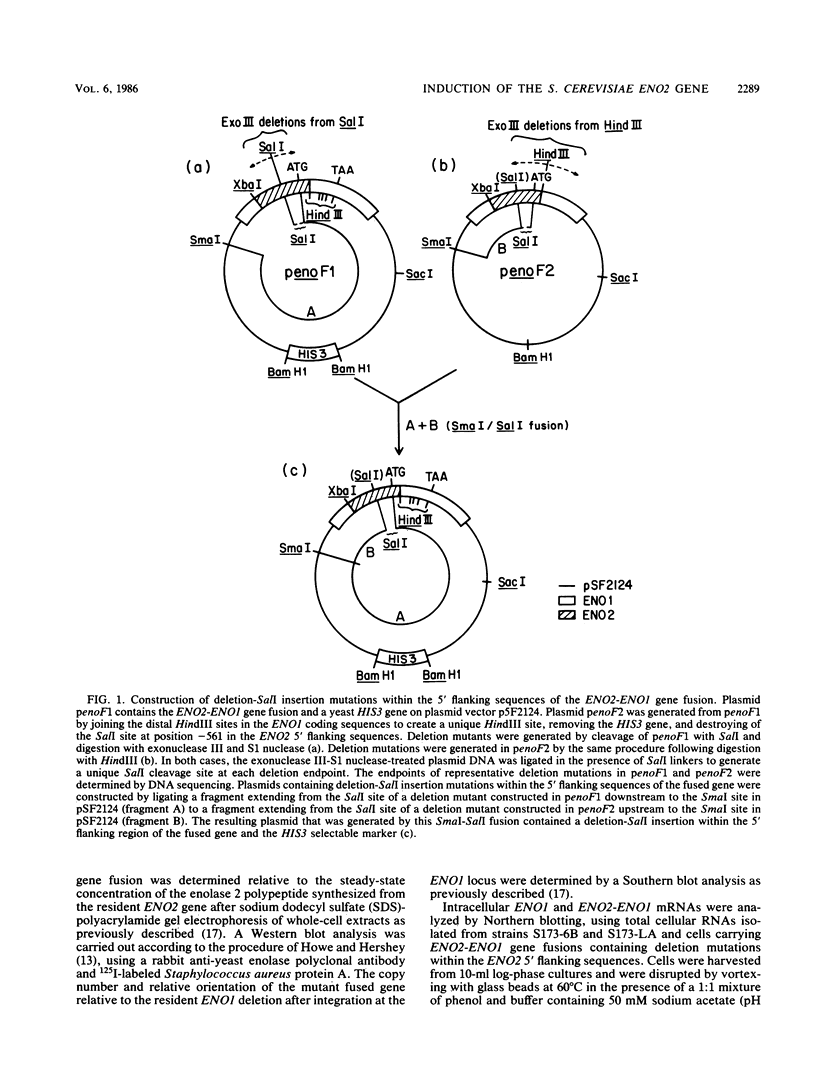
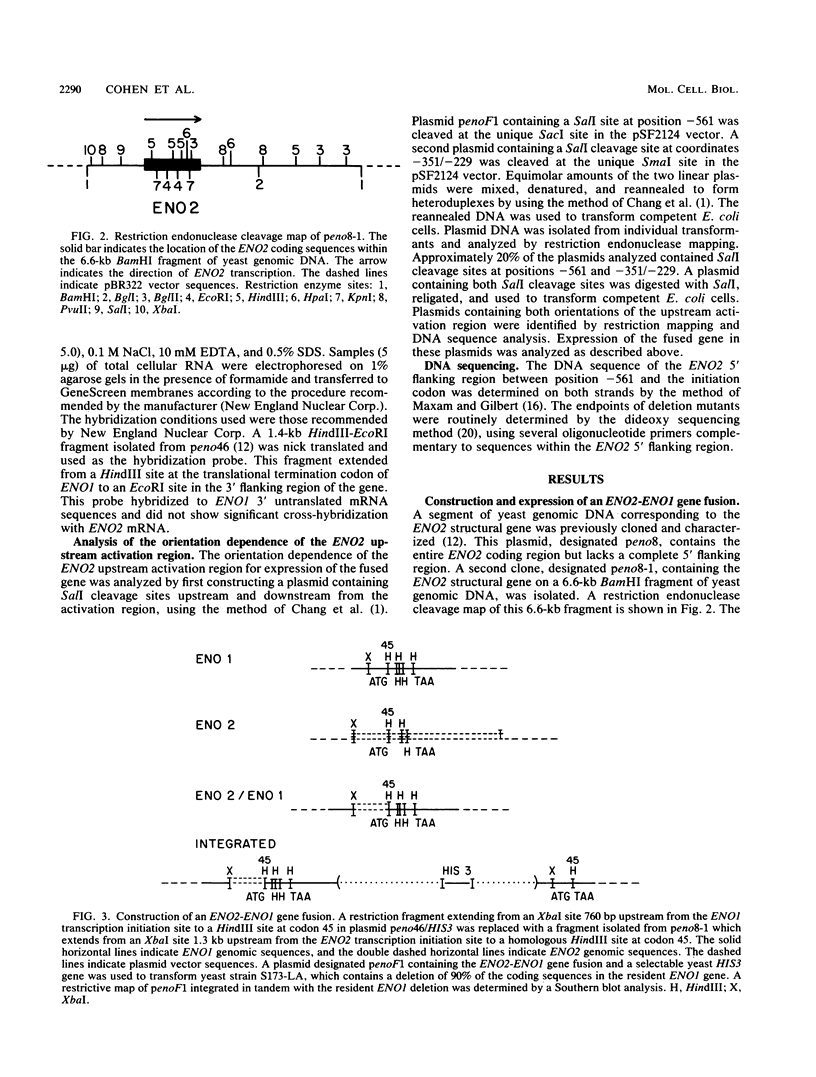
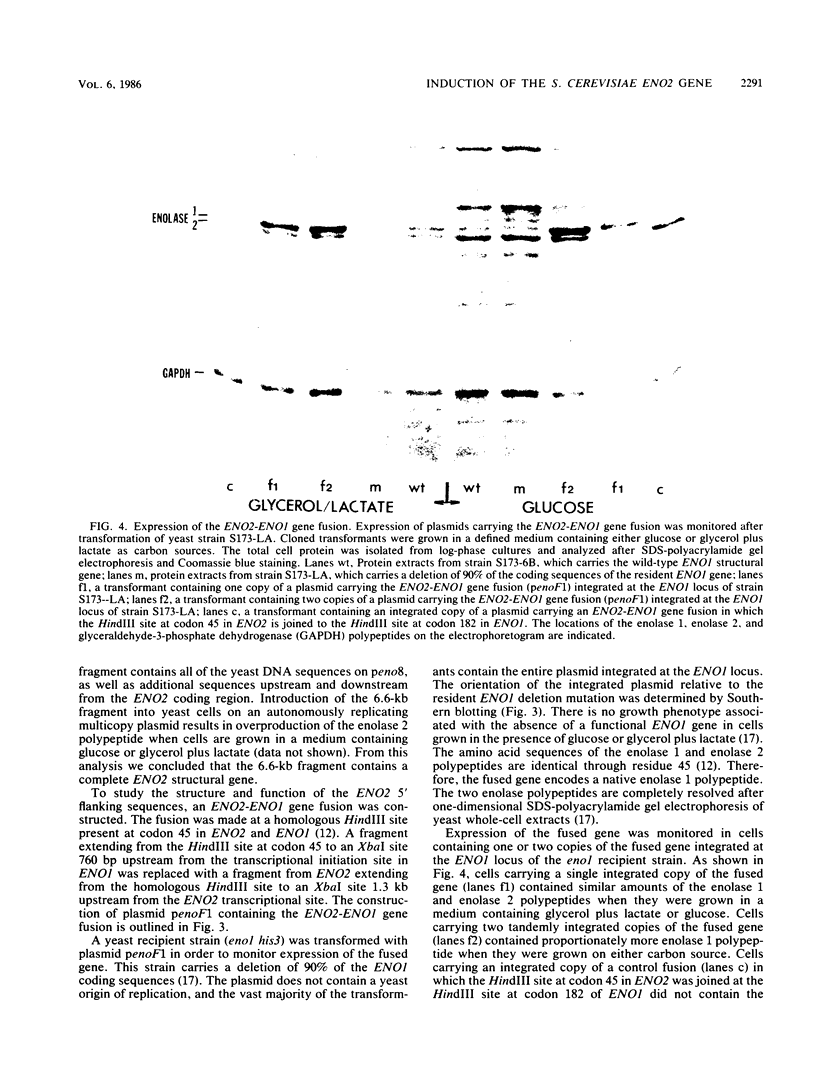
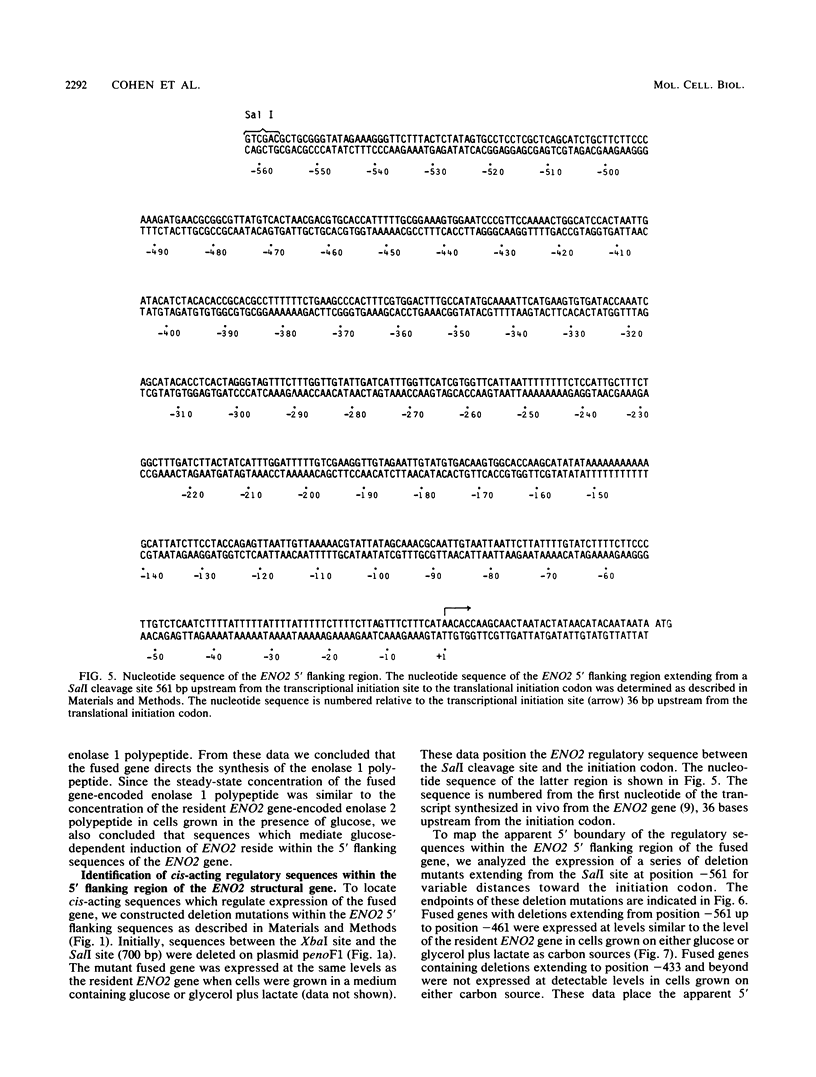
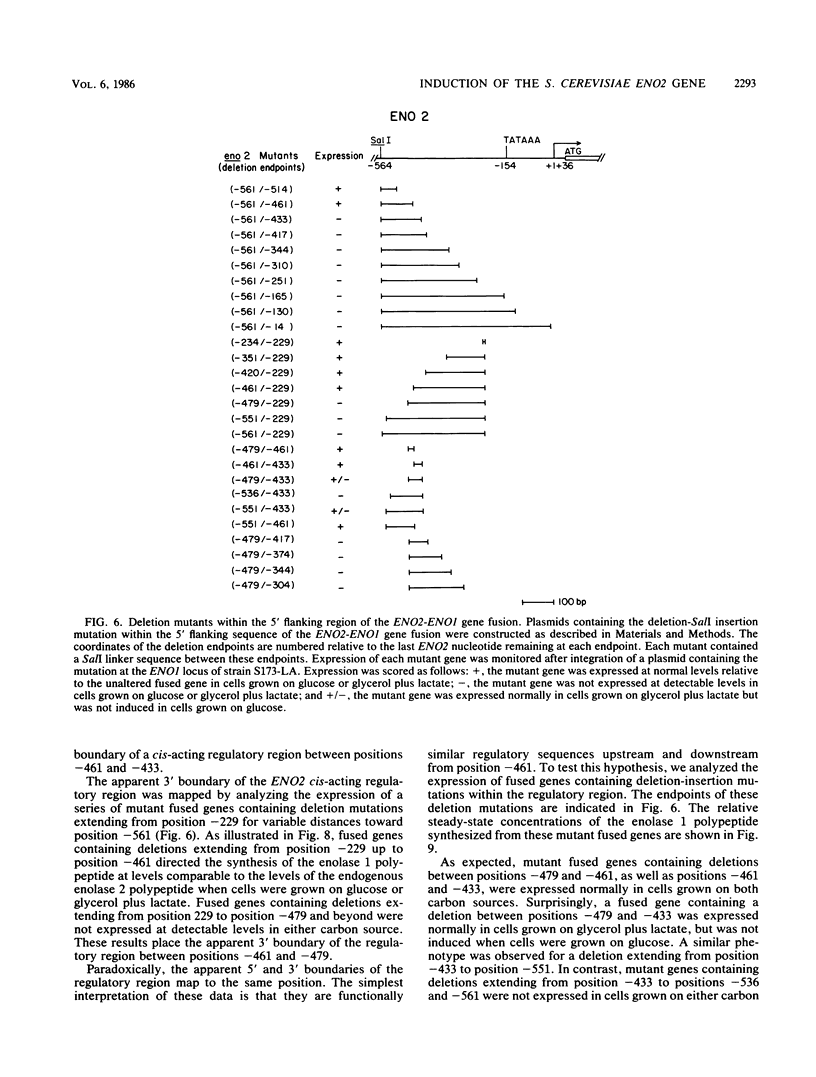
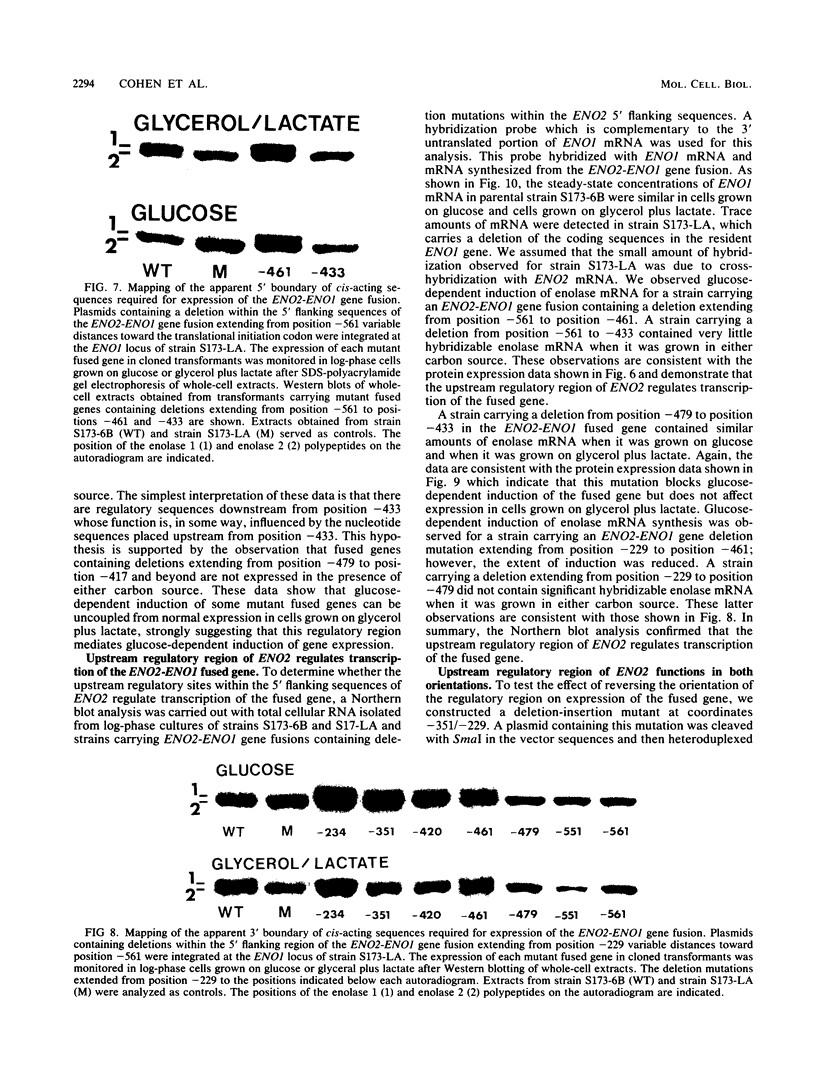
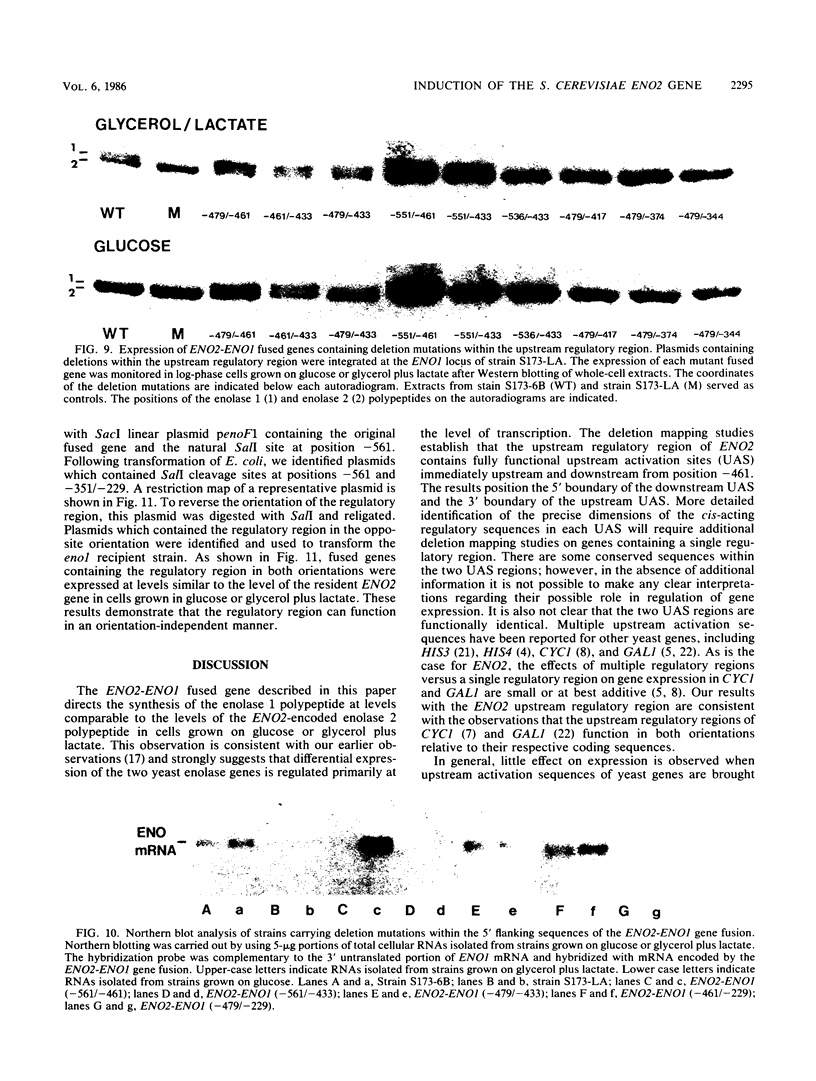
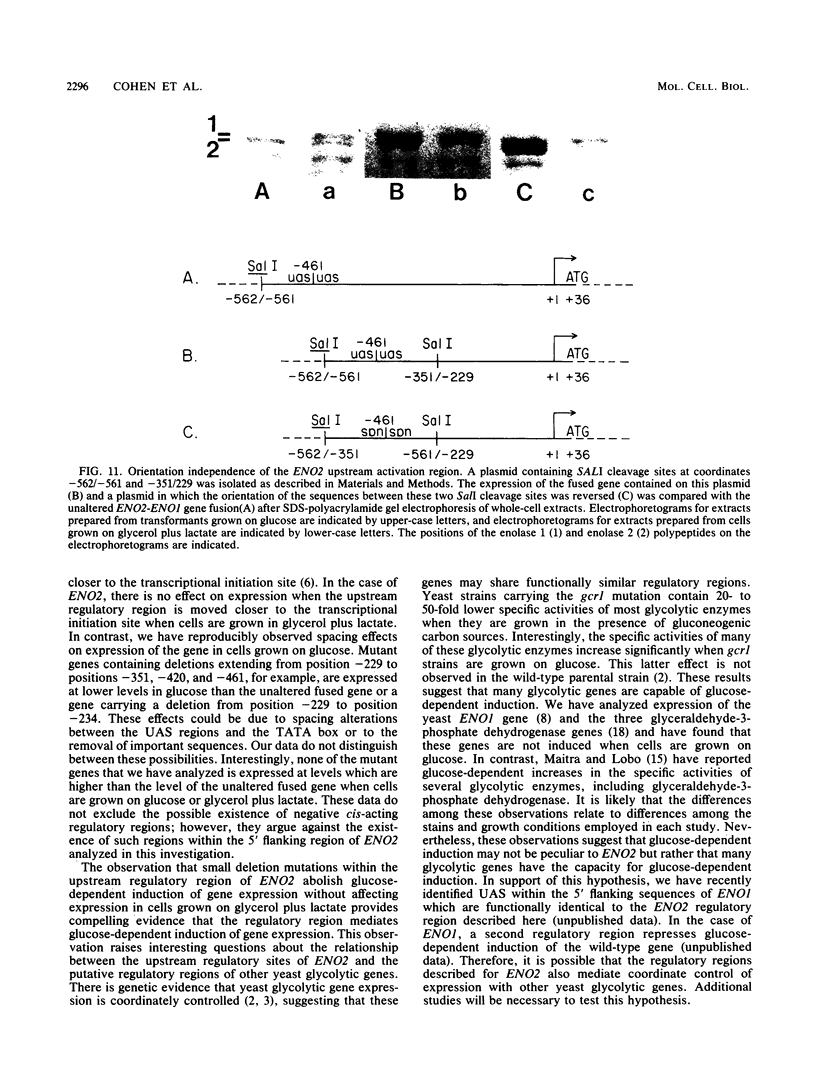
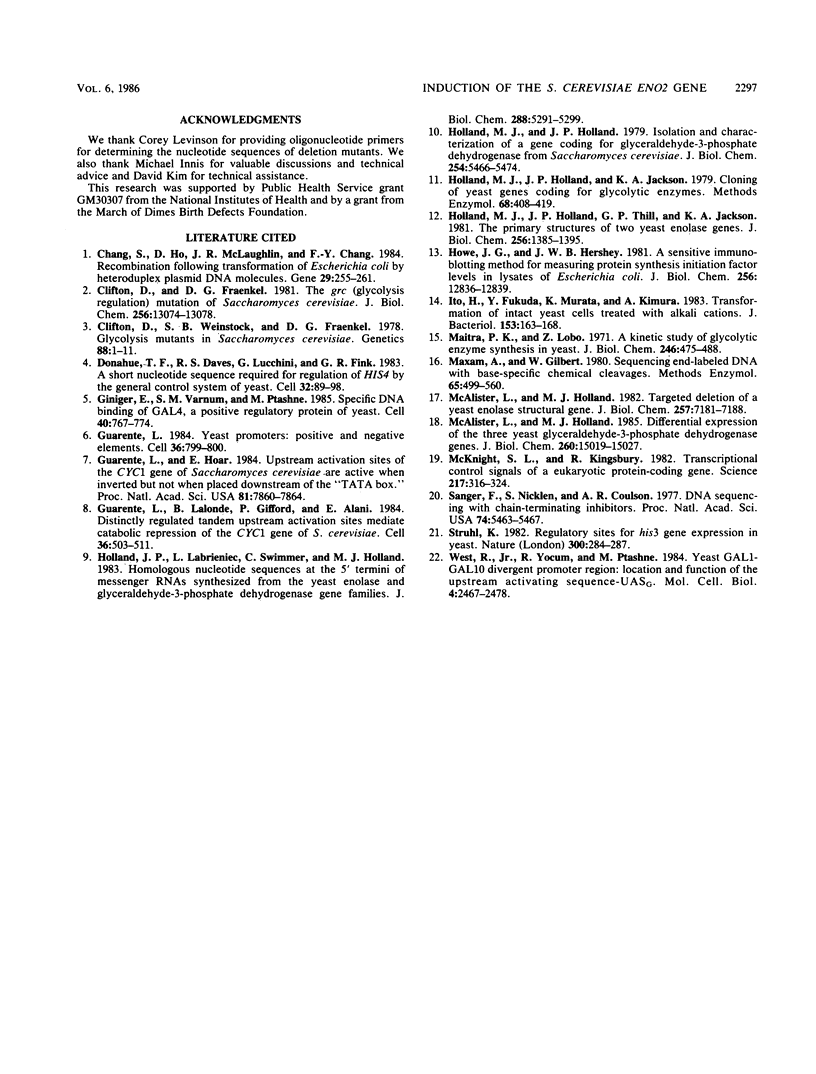
Images in this article
Selected References
These references are in PubMed. This may not be the complete list of references from this article.
- Chang S., Ho D., McLaughlin J. R., Chang S. Y. Recombination following transformation of Escherichia coli by heteroduplex plasmid DNA molecules. Gene. 1984 Sep;29(3):255–261. doi: 10.1016/0378-1119(84)90054-4. [DOI] [PubMed] [Google Scholar]
- Clifton D., Fraenkel D. G. The gcr (glycolysis regulation) mutation of Saccharomyces cerevisiae. J Biol Chem. 1981 Dec 25;256(24):13074–13078. [PubMed] [Google Scholar]
- Clifton D., Weinstock S. B., Fraenkel D. G. Glycolysis mutants in Saccharomyces cerevisiae. Genetics. 1978 Jan;88(1):1–11. doi: 10.1093/genetics/88.1.1. [DOI] [PMC free article] [PubMed] [Google Scholar]
- Donahue T. F., Daves R. S., Lucchini G., Fink G. R. A short nucleotide sequence required for regulation of HIS4 by the general control system of yeast. Cell. 1983 Jan;32(1):89–98. doi: 10.1016/0092-8674(83)90499-3. [DOI] [PubMed] [Google Scholar]
- Giniger E., Varnum S. M., Ptashne M. Specific DNA binding of GAL4, a positive regulatory protein of yeast. Cell. 1985 Apr;40(4):767–774. doi: 10.1016/0092-8674(85)90336-8. [DOI] [PubMed] [Google Scholar]
- Guarente L., Hoar E. Upstream activation sites of the CYC1 gene of Saccharomyces cerevisiae are active when inverted but not when placed downstream of the "TATA box". Proc Natl Acad Sci U S A. 1984 Dec;81(24):7860–7864. doi: 10.1073/pnas.81.24.7860. [DOI] [PMC free article] [PubMed] [Google Scholar]
- Guarente L., Lalonde B., Gifford P., Alani E. Distinctly regulated tandem upstream activation sites mediate catabolite repression of the CYC1 gene of S. cerevisiae. Cell. 1984 Feb;36(2):503–511. doi: 10.1016/0092-8674(84)90243-5. [DOI] [PubMed] [Google Scholar]
- Guarente L. Yeast promoters: positive and negative elements. Cell. 1984 Apr;36(4):799–800. doi: 10.1016/0092-8674(84)90028-x. [DOI] [PubMed] [Google Scholar]
- Holland J. P., Labieniec L., Swimmer C., Holland M. J. Homologous nucleotide sequences at the 5' termini of messenger RNAs synthesized from the yeast enolase and glyceraldehyde-3-phosphate dehydrogenase gene families. The primary structure of a third yeast glyceraldehyde-3-phosphate dehydrogenase gene. J Biol Chem. 1983 Apr 25;258(8):5291–5299. [PubMed] [Google Scholar]
- Holland M. J., Holland J. P. Isolation and characterization of a gene coding for glyceraldehyde-3-phosphate dehydrogenase from Saccharomyces cerevisiae. J Biol Chem. 1979 Jun 25;254(12):5466–5474. [PubMed] [Google Scholar]
- Holland M. J., Holland J. P., Jackson K. A. Cloning of yeast genes coding for glycolytic enzymes. Methods Enzymol. 1979;68:408–419. doi: 10.1016/0076-6879(79)68030-8. [DOI] [PubMed] [Google Scholar]
- Holland M. J., Holland J. P., Thill G. P., Jackson K. A. The primary structures of two yeast enolase genes. Homology between the 5' noncoding flanking regions of yeast enolase and glyceraldehyde-3-phosphate dehydrogenase genes. J Biol Chem. 1981 Feb 10;256(3):1385–1395. [PubMed] [Google Scholar]
- Howe J. G., Hershey J. W. A sensitive immunoblotting method for measuring protein synthesis initiation factor levels in lysates of Escherichia coli. J Biol Chem. 1981 Dec 25;256(24):12836–12839. [PubMed] [Google Scholar]
- Ito H., Fukuda Y., Murata K., Kimura A. Transformation of intact yeast cells treated with alkali cations. J Bacteriol. 1983 Jan;153(1):163–168. doi: 10.1128/jb.153.1.163-168.1983. [DOI] [PMC free article] [PubMed] [Google Scholar]
- Maitra P. K., Lobo Z. A kinetic study of glycolytic enzyme synthesis in yeast. J Biol Chem. 1971 Jan 25;246(2):475–488. [PubMed] [Google Scholar]
- Maxam A. M., Gilbert W. Sequencing end-labeled DNA with base-specific chemical cleavages. Methods Enzymol. 1980;65(1):499–560. doi: 10.1016/s0076-6879(80)65059-9. [DOI] [PubMed] [Google Scholar]
- McAlister L., Holland M. J. Differential expression of the three yeast glyceraldehyde-3-phosphate dehydrogenase genes. J Biol Chem. 1985 Dec 5;260(28):15019–15027. [PubMed] [Google Scholar]
- McAlister L., Holland M. J. Targeted deletion of a yeast enolase structural gene. Identification and isolation of yeast enolase isozymes. J Biol Chem. 1982 Jun 25;257(12):7181–7188. [PubMed] [Google Scholar]
- McKnight S. L., Kingsbury R. Transcriptional control signals of a eukaryotic protein-coding gene. Science. 1982 Jul 23;217(4557):316–324. doi: 10.1126/science.6283634. [DOI] [PubMed] [Google Scholar]
- Sanger F., Nicklen S., Coulson A. R. DNA sequencing with chain-terminating inhibitors. Proc Natl Acad Sci U S A. 1977 Dec;74(12):5463–5467. doi: 10.1073/pnas.74.12.5463. [DOI] [PMC free article] [PubMed] [Google Scholar]
- West R. W., Jr, Yocum R. R., Ptashne M. Saccharomyces cerevisiae GAL1-GAL10 divergent promoter region: location and function of the upstream activating sequence UASG. Mol Cell Biol. 1984 Nov;4(11):2467–2478. doi: 10.1128/mcb.4.11.2467. [DOI] [PMC free article] [PubMed] [Google Scholar]