Abstract
The distribution of cytoplasmic messenger ribonucleic acids (RNAs) in translationally active polysomes and inactive ribonucleoprotein particles changes during early development. Cellular levels and subcellular distributions have been determined for most messenger RNAs, but little is known about how individual sequences change. In this study, we used hybridization techniques with cloned sequences to measure the titers of 23 mitochondrial and non-mitochondrial polyadenylate-containing [poly(A)+]RNA species during early development in the frog Xenopus laevis. These RNA species were some of the most abundant cellular poly(A)+ RNA species in early embryos. The concentrations of most of the non-mitochondrial (cytoplasmic) RNAs remained constant in embryos during the first 10 h of development, although the concentrations of a few species increased. During neurulation, we detected several new poly(A)+ RNA sequences in polysomes, and with one possible exception the accumulation of these sequences was largely the result of new synthesis or de novo polyadenylation and not due to the recruitment of nonpolysomal (free ribonucleoprotein) poly(A)+ RNA. We measured the subcellular distributions of these RNA species in polysomes and free ribonucleoproteins during early development. In gastrulae, non-mitochondrial RNAs were distributed differentially between the two cell fractions; some RNA species were represented more in free ribonucleoproteins, and others were represented less. By the neurula stage this differential distribution in polysomes and free ribonucleoproteins was less pronounced, and we found species almost entirely in polysomes. Some poly(A)+ RNA species transcribed from the mitochondrial genome were localized within the mitochondria and were mapped to discrete fragments of the mitochondrial genome. Much of this poly(A)+ RNA was transcribed from the ribosomal locus. Nonribosomal mitochondrial poly(A)+ RNA species became enriched in polysome-like structures after fertilization, with time courses similar to the time course of mobilization of cytoplasmic poly(A)+ RNA.
Full text
PDF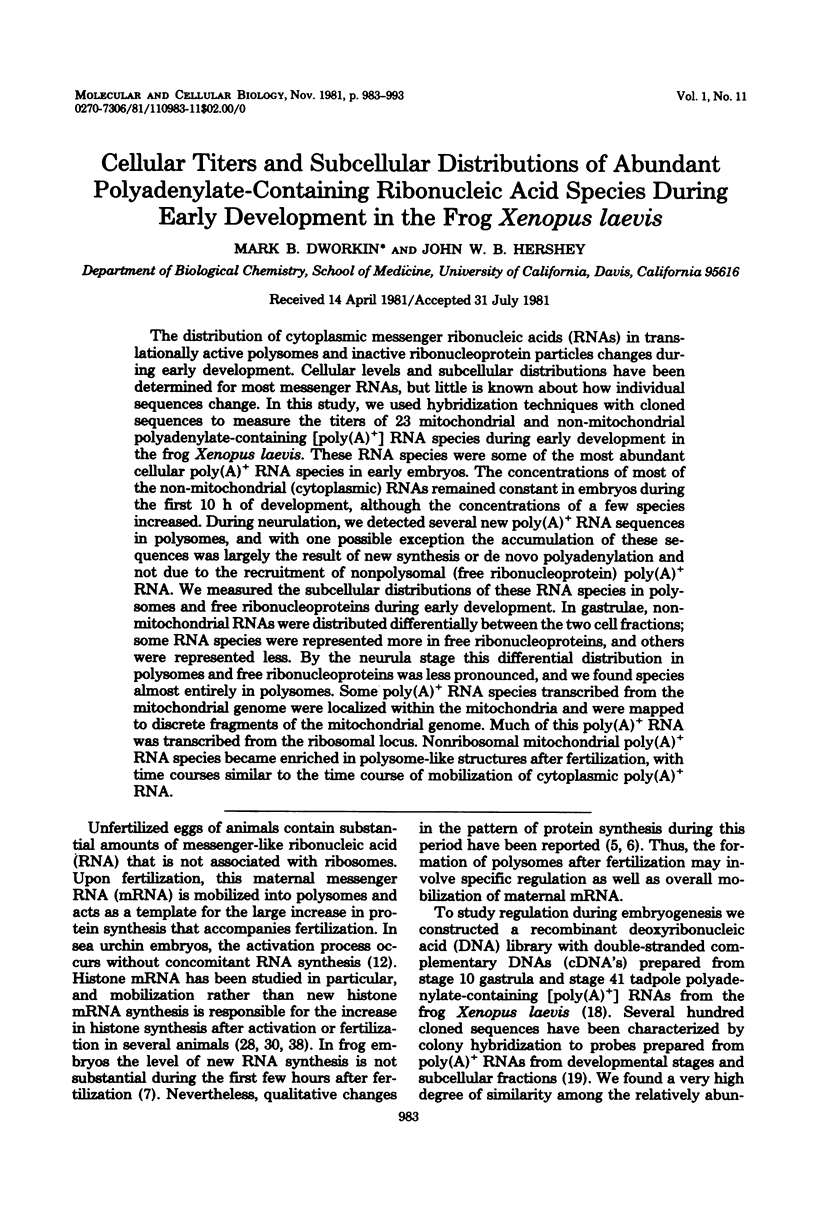
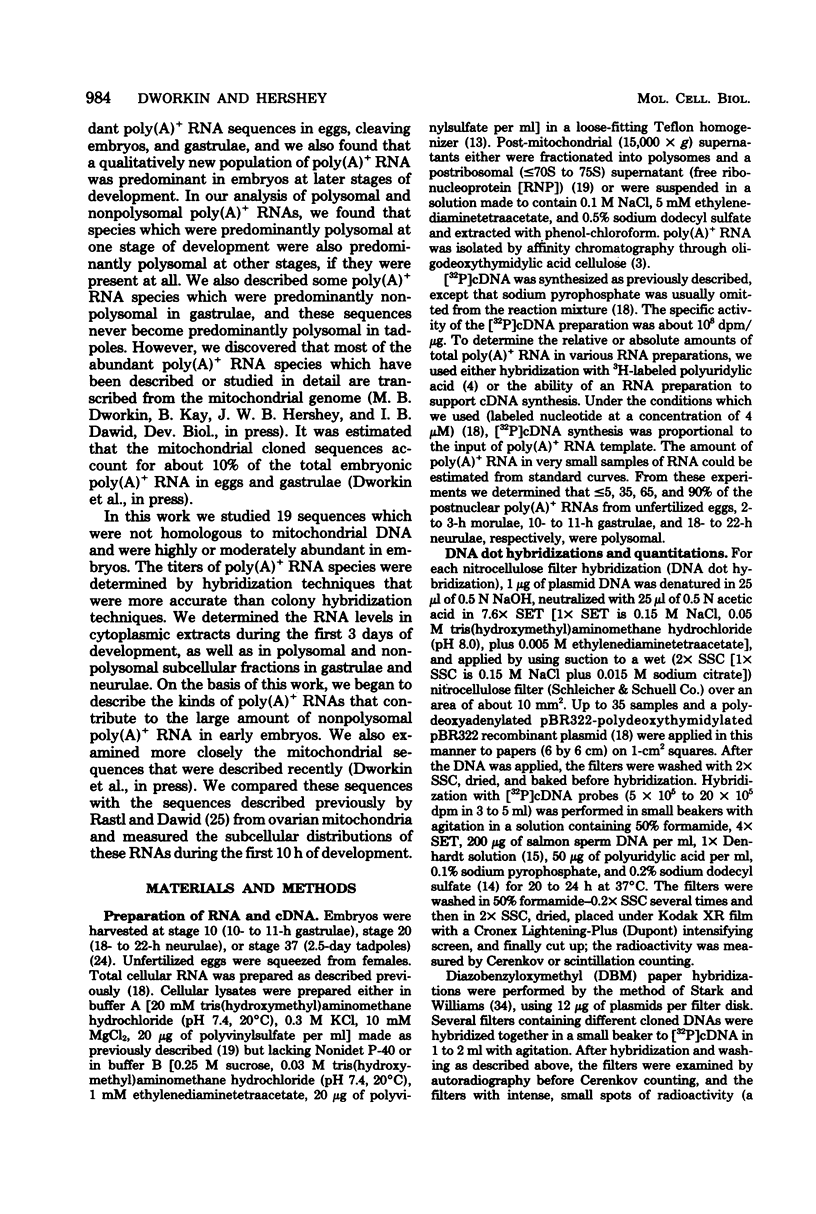
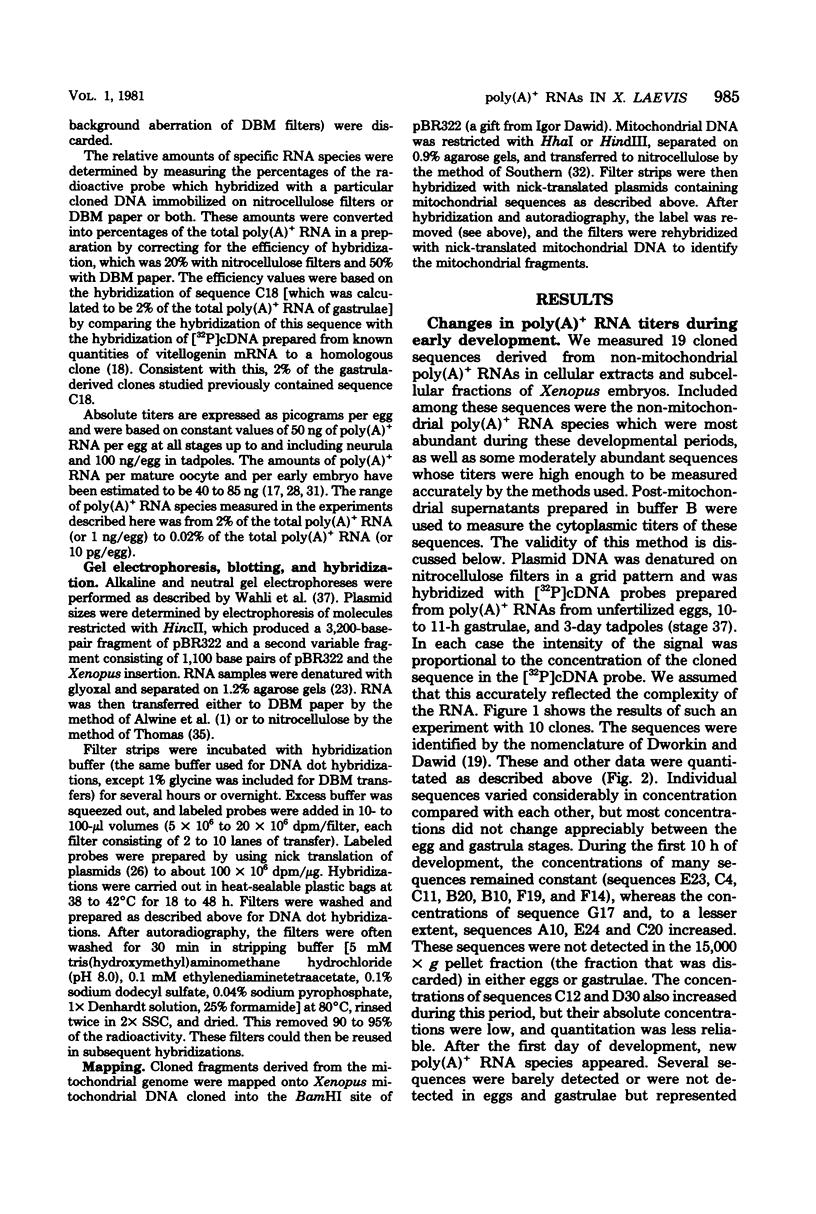
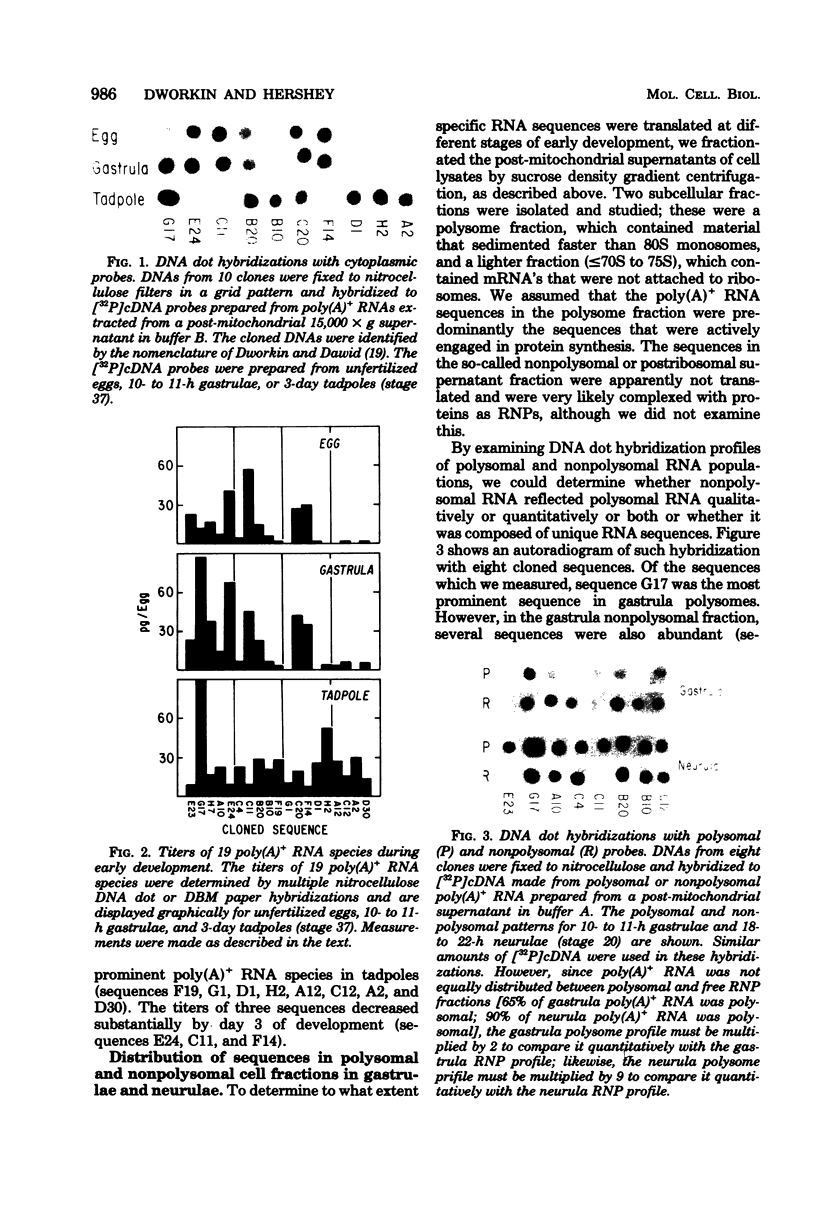
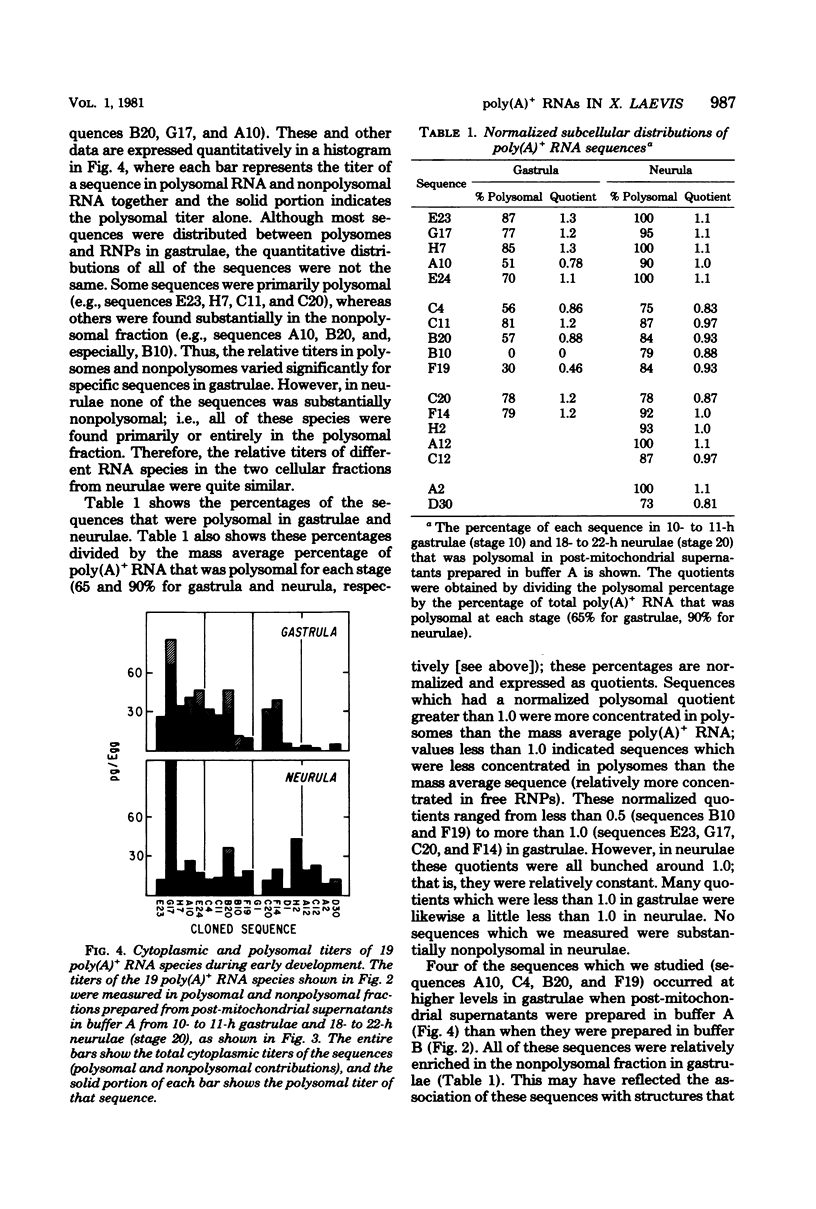
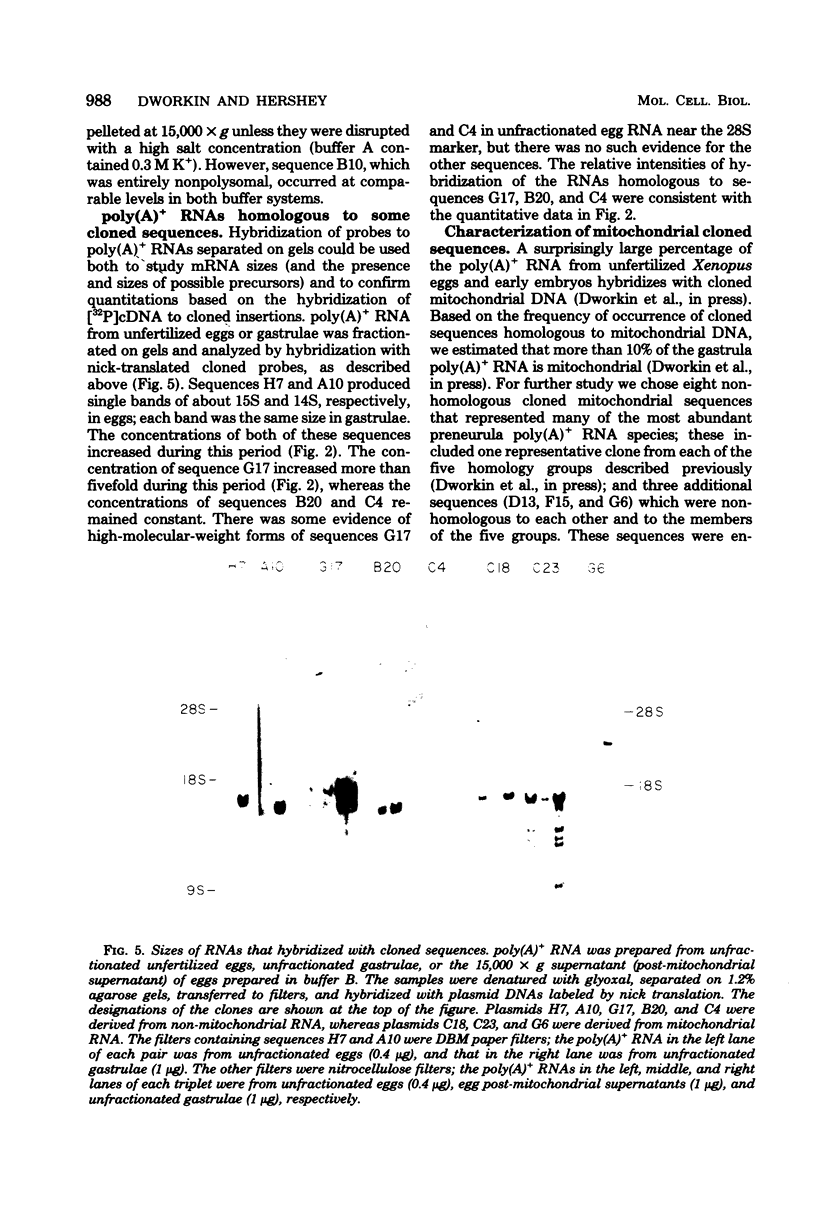
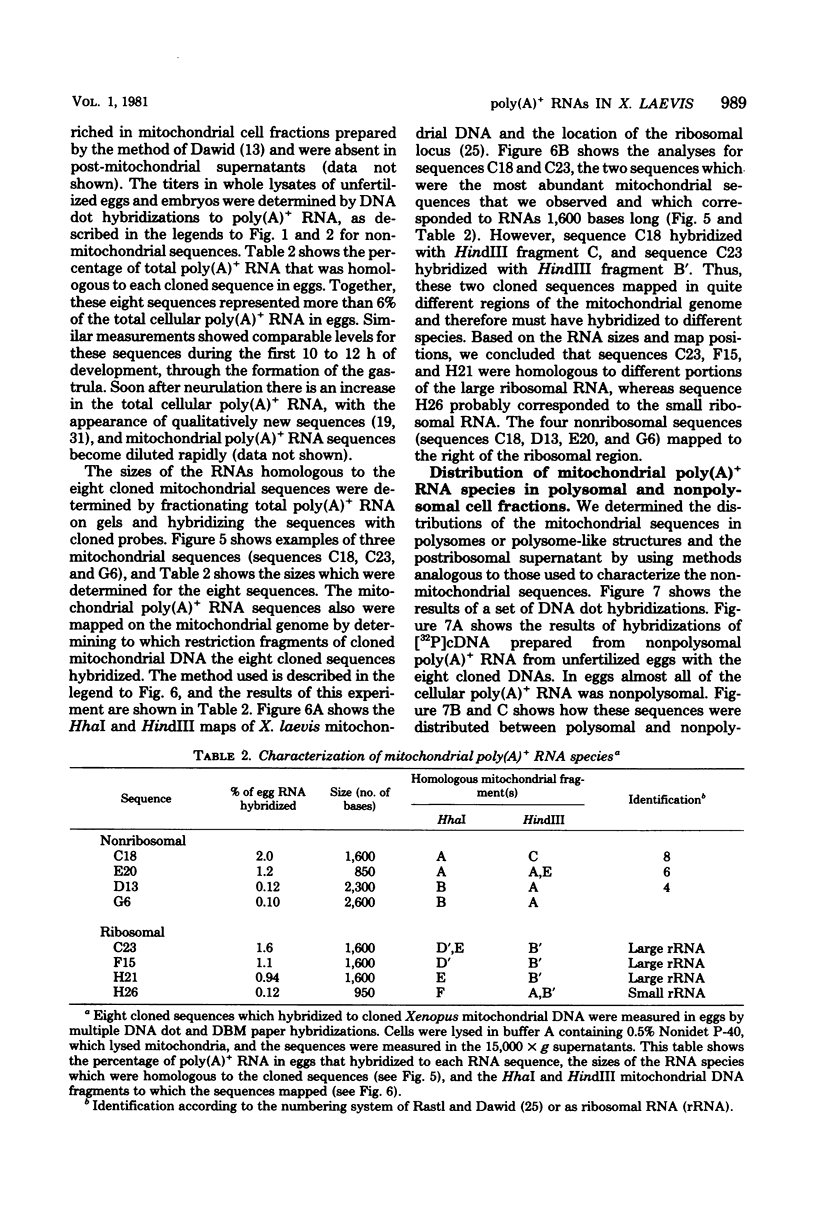
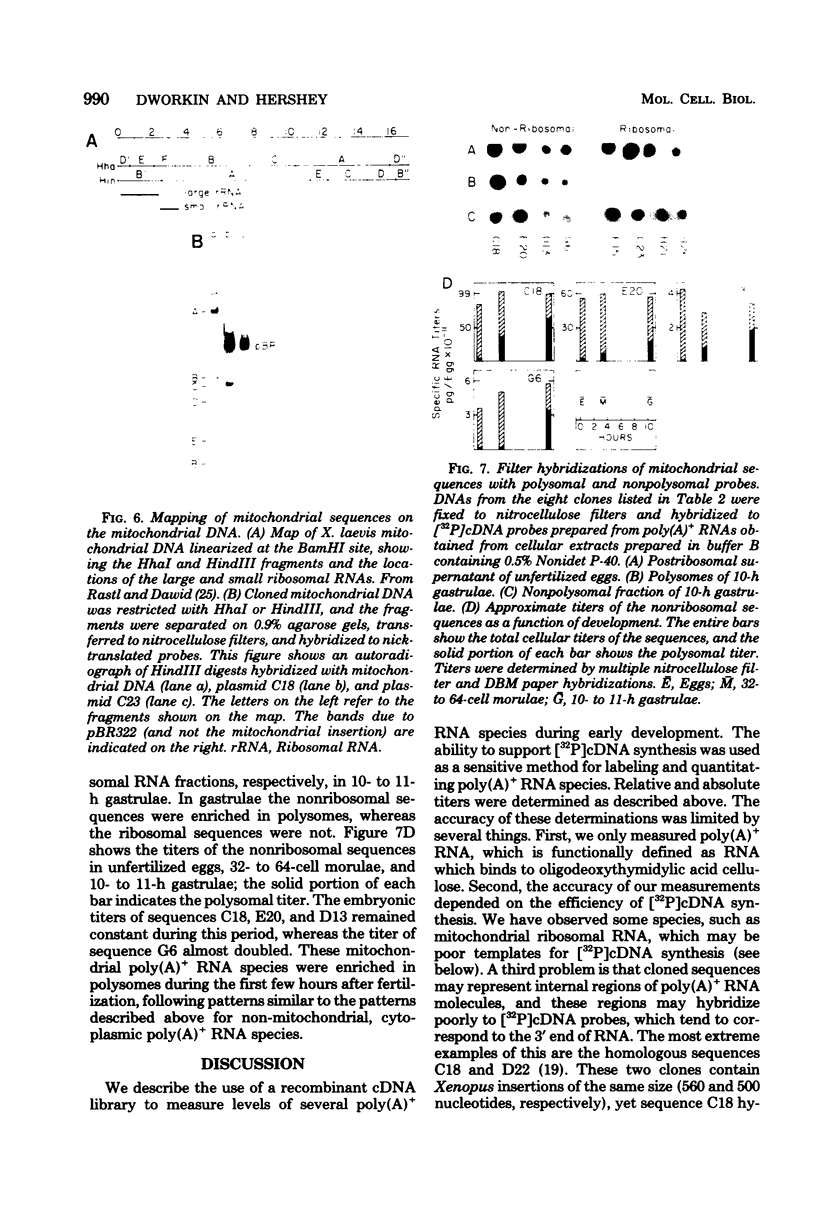
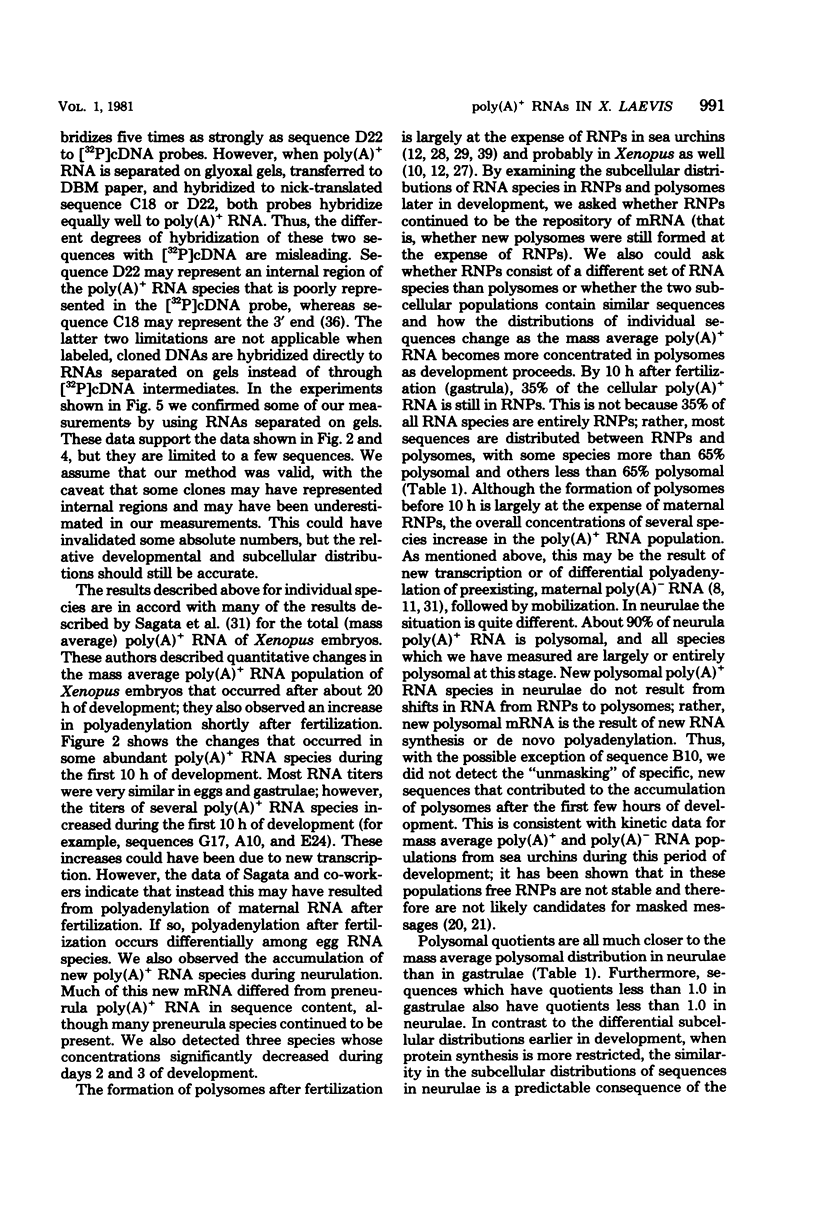
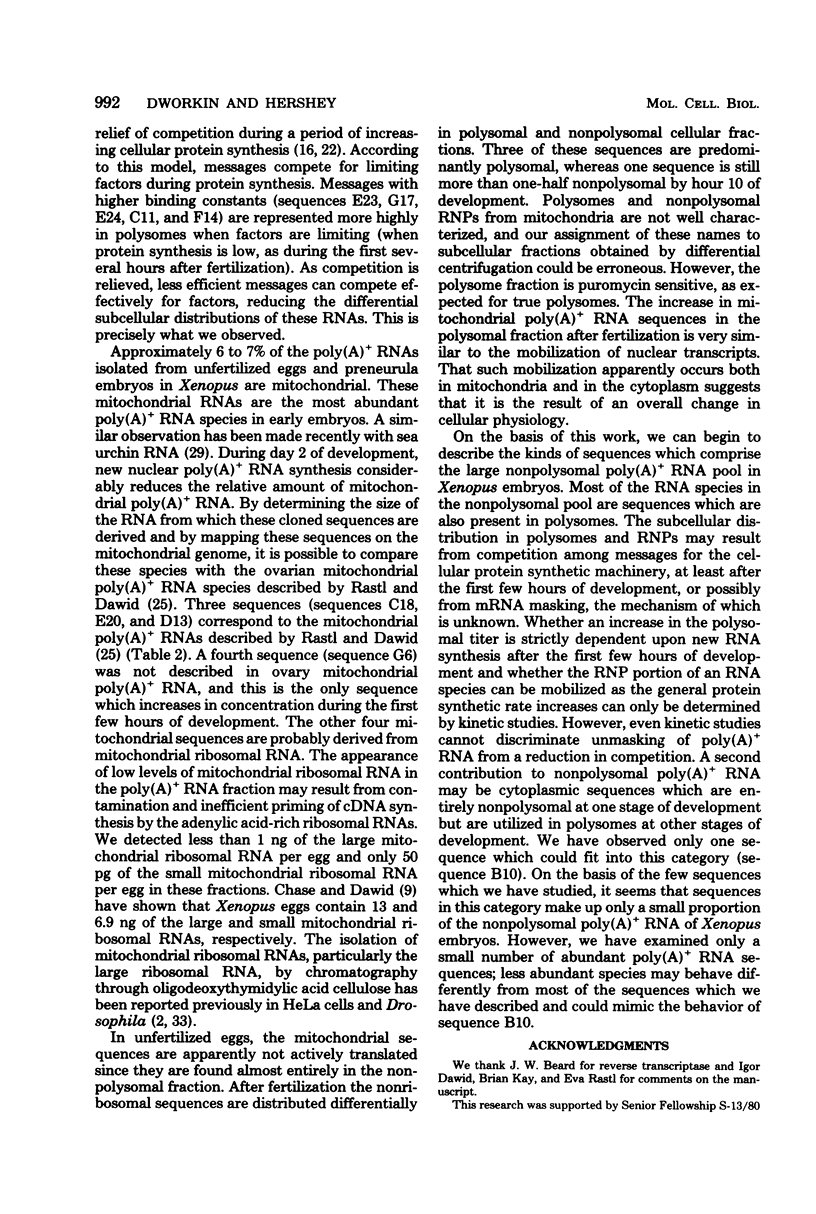
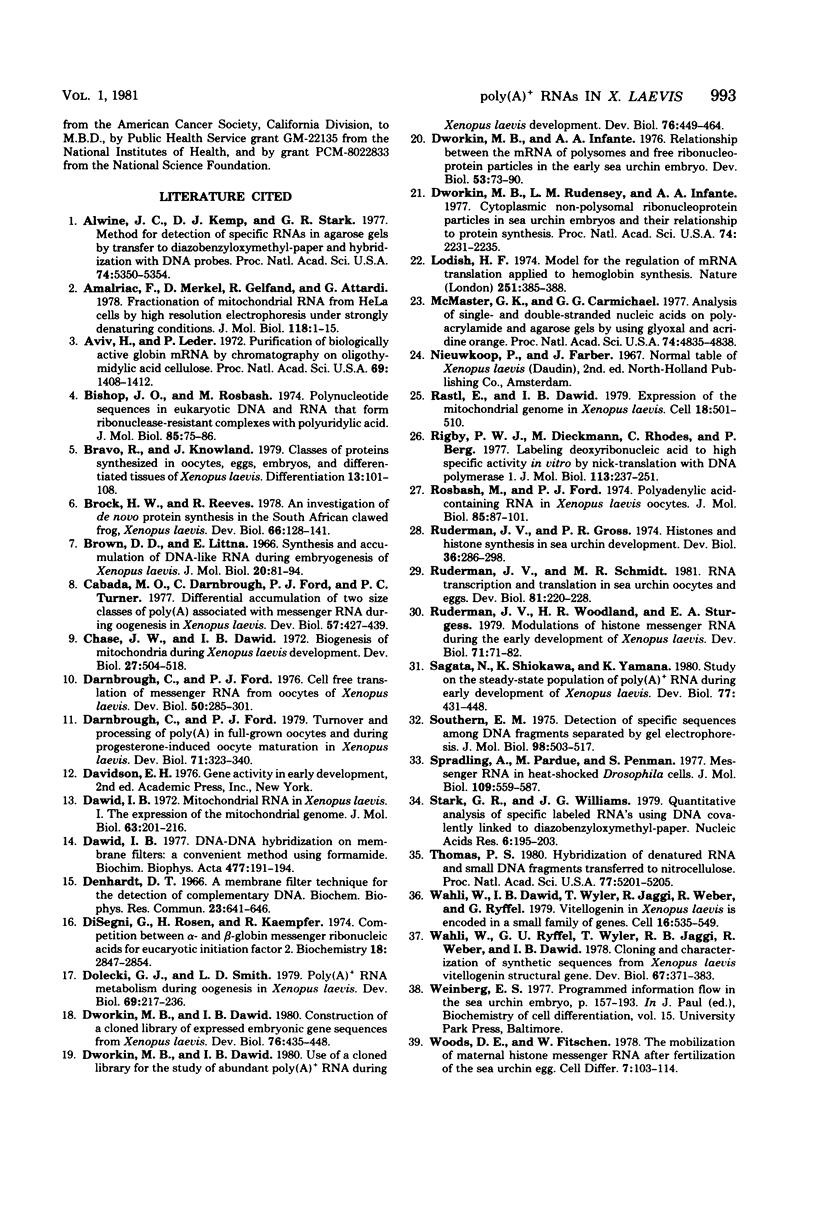
Images in this article
Selected References
These references are in PubMed. This may not be the complete list of references from this article.
- Alwine J. C., Kemp D. J., Stark G. R. Method for detection of specific RNAs in agarose gels by transfer to diazobenzyloxymethyl-paper and hybridization with DNA probes. Proc Natl Acad Sci U S A. 1977 Dec;74(12):5350–5354. doi: 10.1073/pnas.74.12.5350. [DOI] [PMC free article] [PubMed] [Google Scholar]
- Amalric F., Merkel C., Gelfand R., Attardi G. Fractionation of mitochondrial RNA from HeLa cells by high-resolution electrophoresis under strongly denaturing conditions. J Mol Biol. 1978 Jan 5;118(1):1–25. doi: 10.1016/0022-2836(78)90241-3. [DOI] [PubMed] [Google Scholar]
- Aviv H., Leder P. Purification of biologically active globin messenger RNA by chromatography on oligothymidylic acid-cellulose. Proc Natl Acad Sci U S A. 1972 Jun;69(6):1408–1412. doi: 10.1073/pnas.69.6.1408. [DOI] [PMC free article] [PubMed] [Google Scholar]
- Bishop J. O., Rosbash M. Polynucleotide sequences in eukaryotic DNA and RNA that form ribonuclease-resistant complexes with polyuridylic acid. J Mol Biol. 1974 May 5;85(1):75–86. doi: 10.1016/0022-2836(74)90130-2. [DOI] [PubMed] [Google Scholar]
- Bravo R., Knowland J. Classes of proteins synthesized in oocytes, eggs, embryos, and differentiated tissues of Xenopus laevis. Differentiation. 1979;13(2):101–108. doi: 10.1111/j.1432-0436.1979.tb01572.x. [DOI] [PubMed] [Google Scholar]
- Brock H. W., Reeves R. An investigation of de novo protein synthesis in the South African clawed frog, Xenopus laevis. Dev Biol. 1978 Sep;66(1):128–141. doi: 10.1016/0012-1606(78)90279-8. [DOI] [PubMed] [Google Scholar]
- Brown D. D., Littna E. Synthesis and accumulation of DNA-like RNA during embryogenesis of Xenopus laevis. J Mol Biol. 1966 Sep;20(1):81–94. doi: 10.1016/0022-2836(66)90119-7. [DOI] [PubMed] [Google Scholar]
- Cabada M. O., Darnbrough C., Ford P. J., Turner P. C. Differential accumulation of two size classes of poly(A) associated with messenger RNA during oogenesis in Xenopus laevis. Dev Biol. 1977 Jun;57(2):427–439. doi: 10.1016/0012-1606(77)90227-5. [DOI] [PubMed] [Google Scholar]
- Chase J. W., Dawid I. B. Biogenesis of mitochondria during Xenopus laevis development. Dev Biol. 1972 Apr;27(4):504–518. doi: 10.1016/0012-1606(72)90189-3. [DOI] [PubMed] [Google Scholar]
- Darnbrough C., Ford P. J. Cell-free translation of messenger RNA from oocytes of Xenopus laevis. Dev Biol. 1976 Jun;50(2):285–301. doi: 10.1016/0012-1606(76)90152-4. [DOI] [PubMed] [Google Scholar]
- Darnbrough C., Ford P. J. Turnover and processing of poly(A) in full-grown oocytes and during progesterone-induced oocyte maturation in Xenopus laevis. Dev Biol. 1979 Aug;71(2):323–340. doi: 10.1016/0012-1606(79)90173-8. [DOI] [PubMed] [Google Scholar]
- Dawid I. B. DNA-DNA hybridization on membrane filters: a convenient method using formamide. Biochim Biophys Acta. 1977 Jul 15;477(2):191–194. doi: 10.1016/0005-2787(77)90235-0. [DOI] [PubMed] [Google Scholar]
- Dawid I. B. Mitochondrial RNA In Xenopus laevis. I. The expression of the mitochondrial genome. J Mol Biol. 1972 Jan 28;63(2):201–216. doi: 10.1016/0022-2836(72)90370-1. [DOI] [PubMed] [Google Scholar]
- Denhardt D. T. A membrane-filter technique for the detection of complementary DNA. Biochem Biophys Res Commun. 1966 Jun 13;23(5):641–646. doi: 10.1016/0006-291x(66)90447-5. [DOI] [PubMed] [Google Scholar]
- Di Segni G., Rosen H., Kaempfer R. Competition between alpha- and beta-globin messenger ribonucleic acids for eucaryotic initiation factor 2. Biochemistry. 1979 Jun 26;18(13):2847–2854. doi: 10.1021/bi00580a027. [DOI] [PubMed] [Google Scholar]
- Dolecki G. J., Smith L. D. Poly(A)+ RNA metabolism during oogenesis in Xenopus laevis. Dev Biol. 1979 Mar;69(1):217–236. doi: 10.1016/0012-1606(79)90287-2. [DOI] [PubMed] [Google Scholar]
- Dworkin M. B., Dawid I. B. Construction of a cloned library of expressed embryonic gene sequences from Xenopus laevis. Dev Biol. 1980 May;76(2):435–448. doi: 10.1016/0012-1606(80)90392-9. [DOI] [PubMed] [Google Scholar]
- Dworkin M. B., Dawid I. B. Use of a cloned library for the study of abundant poly(A)+RNA during Xenopus laevis development. Dev Biol. 1980 May;76(2):449–464. doi: 10.1016/0012-1606(80)90393-0. [DOI] [PubMed] [Google Scholar]
- Dworkin M. B., Infante A. A. Relationship between the mRNA of polysomes and free ribonucleoprotein particles in the early sea urchin embryo. Dev Biol. 1976 Oct 1;53(1):73–90. doi: 10.1016/0012-1606(76)90210-4. [DOI] [PubMed] [Google Scholar]
- Dworkin M. B., Rudensey L. M., Infante A. A. Cytoplasmic nonpolysomal ribonucleoprotein particles in sea urchin embryos and their relationship to protein synthesis. Proc Natl Acad Sci U S A. 1977 Jun;74(6):2231–2235. doi: 10.1073/pnas.74.6.2231. [DOI] [PMC free article] [PubMed] [Google Scholar]
- Lodish H. F. Model for the regulation of mRNA translation applied to haemoglobin synthesis. Nature. 1974 Oct 4;251(5474):385–388. doi: 10.1038/251385a0. [DOI] [PubMed] [Google Scholar]
- McMaster G. K., Carmichael G. G. Analysis of single- and double-stranded nucleic acids on polyacrylamide and agarose gels by using glyoxal and acridine orange. Proc Natl Acad Sci U S A. 1977 Nov;74(11):4835–4838. doi: 10.1073/pnas.74.11.4835. [DOI] [PMC free article] [PubMed] [Google Scholar]
- Rastl E., Dawid I. B. Expression of the mitochondrial genome in Xenopus laevis: a map of transcripts. Cell. 1979 Oct;18(2):501–510. doi: 10.1016/0092-8674(79)90067-9. [DOI] [PubMed] [Google Scholar]
- Rigby P. W., Dieckmann M., Rhodes C., Berg P. Labeling deoxyribonucleic acid to high specific activity in vitro by nick translation with DNA polymerase I. J Mol Biol. 1977 Jun 15;113(1):237–251. doi: 10.1016/0022-2836(77)90052-3. [DOI] [PubMed] [Google Scholar]
- Rosbash M. Polyadenylic acid-containing RNA in Xenopus laevis oocytes. J Mol Biol. 1974 May 5;85(1):87–101. doi: 10.1016/0022-2836(74)90131-4. [DOI] [PubMed] [Google Scholar]
- Ruderman J. V., Gross P. R. Histones and histone synthesis in sea urchin development. Dev Biol. 1974 Feb;36(2):286–298. doi: 10.1016/0012-1606(74)90052-9. [DOI] [PubMed] [Google Scholar]
- Ruderman J. V., Schmidt M. R. RNA transcription and translation in sea urchin oocytes and eggs. Dev Biol. 1981 Jan 30;81(2):220–228. doi: 10.1016/0012-1606(81)90285-2. [DOI] [PubMed] [Google Scholar]
- Ruderman J. V., Woodland H. R., Sturgess E. A. Modulations of histone messenger RNA during the early development of Xenopus laevis. Dev Biol. 1979 Jul;71(1):71–82. doi: 10.1016/0012-1606(79)90083-6. [DOI] [PubMed] [Google Scholar]
- Sagata N., Shiokawa K., Yamana K. A study on the steady-state population of poly(A)+RNA during early development of Xenopus laevis. Dev Biol. 1980 Jun 15;77(2):431–448. doi: 10.1016/0012-1606(80)90486-8. [DOI] [PubMed] [Google Scholar]
- Southern E. M. Detection of specific sequences among DNA fragments separated by gel electrophoresis. J Mol Biol. 1975 Nov 5;98(3):503–517. doi: 10.1016/s0022-2836(75)80083-0. [DOI] [PubMed] [Google Scholar]
- Spradling A., Pardue M. L., Penman S. Messenger RNA in heat-shocked Drosophila cells. J Mol Biol. 1977 Feb 5;109(4):559–587. doi: 10.1016/s0022-2836(77)80091-0. [DOI] [PubMed] [Google Scholar]
- Stark G. R., Williams J. G. Quantitative analysis of specific labelled RNA'S using DNA covalently linked to diazobenzyloxymethyl-paper. Nucleic Acids Res. 1979 Jan;6(1):195–203. doi: 10.1093/nar/6.1.195. [DOI] [PMC free article] [PubMed] [Google Scholar]
- Thomas P. S. Hybridization of denatured RNA and small DNA fragments transferred to nitrocellulose. Proc Natl Acad Sci U S A. 1980 Sep;77(9):5201–5205. doi: 10.1073/pnas.77.9.5201. [DOI] [PMC free article] [PubMed] [Google Scholar]
- Wahli W., Dawid I. B., Wyler T., Jaggi R. B., Weber R., Ryffel G. U. Vitellogenin in Xenopus laevis is encoded in a small family of genes. Cell. 1979 Mar;16(3):535–549. doi: 10.1016/0092-8674(79)90028-x. [DOI] [PubMed] [Google Scholar]
- Wahli W., Ryffel G. U., Wyler T., Jaggi F. B., Weber R., Dawid I. B. Cloning and characterization of synthetic sequences from the Xenopus iaevis vitellogenin structural gene. Dev Biol. 1978 Dec;67(2):371–383. doi: 10.1016/0012-1606(78)90207-5. [DOI] [PubMed] [Google Scholar]
- Woods D. E., Fitschen W. The mobilization of maternal histone messenger RNA after fertilization of the sea urchin egg. Cell Differ. 1978 Apr;7(1-2):103–114. doi: 10.1016/0045-6039(78)90011-8. [DOI] [PubMed] [Google Scholar]