Abstract
To investigate the contribution of branched-chain amino acids as a nitrogen source for alanine in vivo, dogs were infused with l-[15N]leucine, l-[U-14C]leucine, l-[2,3,3,3-2H4]alanine, and d-[6,6-2H2]-glucose. 14C and 15N isotopic equilibrium in plasma leucine, and deuterium enrichment in arterial and femoral plasma glucose and alanine were achieved within 3 h of initiation of the respective isotope infusion in all animals. The average flux of leucine determined by [15N]leucine was 5.4 μmol·kg−1·min−1, whereas using [14C]leucine it was 3.7 μmol·kg−1·min−1. Turnover rates for alanine and glucose were 11.0 and 17.2 μmol·kg−1·min−1, respectively.
[15N]alanine was detected as early as 30 min, but nitrogen isotopic equilibrium in alanine was not achieved until 6 h. The absolute rate of leucine nitrogen transfer to alanine was 1.92 μmol·kg−1·min−1, which represented 41-73% (mean 53%) of leucine's nitrogen and 15-20% (mean 18%) of alanine's nitrogen.
Fractional extraction of alanine and leucine by the dog hindlimb was 35 and 24%, respectively. Average net alanine balance was −6.7 μmol·leg−1·min−1, reflecting a release rate (17.4 μmol·kg−1·min−1) that exceeded the rate of uptake (10.8 μmol·leg−1·min−1). Of the leucine taken up by the hindlimb, 34% transferred its nitrogen to alanine and 8% was oxidized to CO2. Since the latter value reflects transamination as well as irreversible catabolism, the nitrogen derived from the oxidation of leucine by the hindlimb could account for only 25% of the observed 15N incorporation into alanine.
The significantly faster flux of leucine nitrogen when compared with leucine carbon suggests significant recycling of the leucine α-ketoacid. These studies demonstrate that leucine is a major donor of nitrogen to circulating alanine in vivo.
Full text
PDF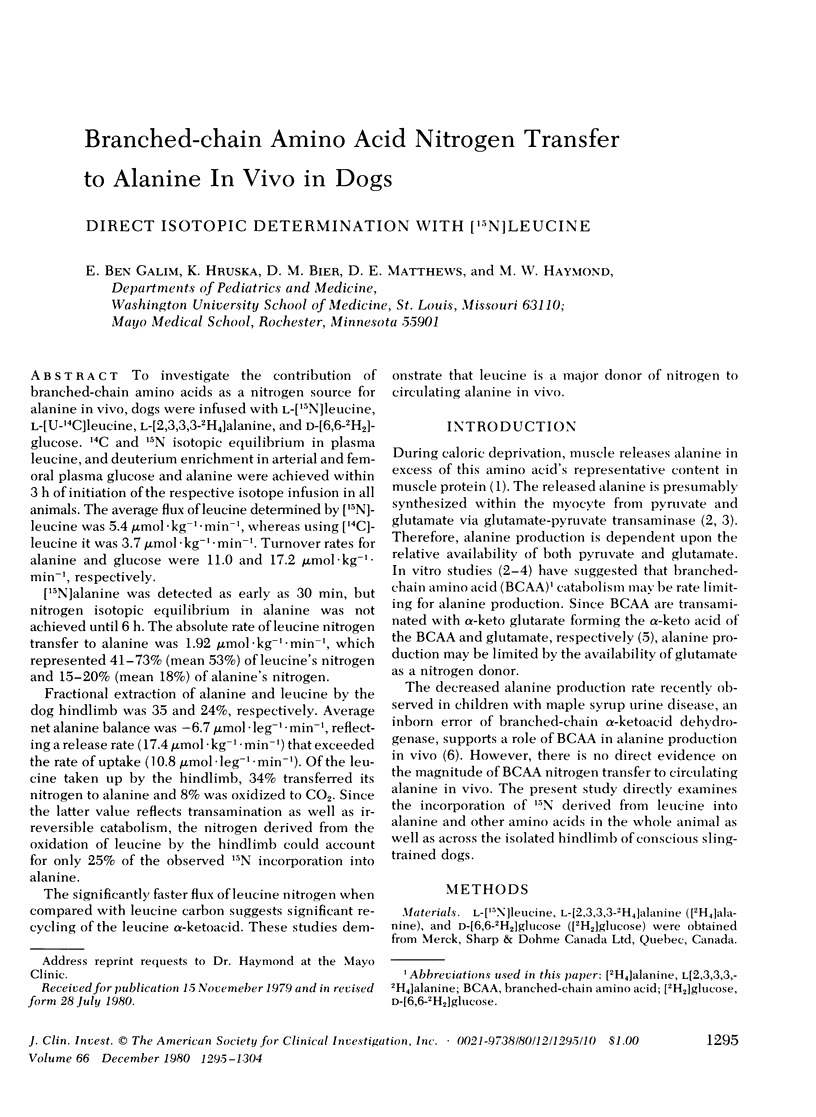
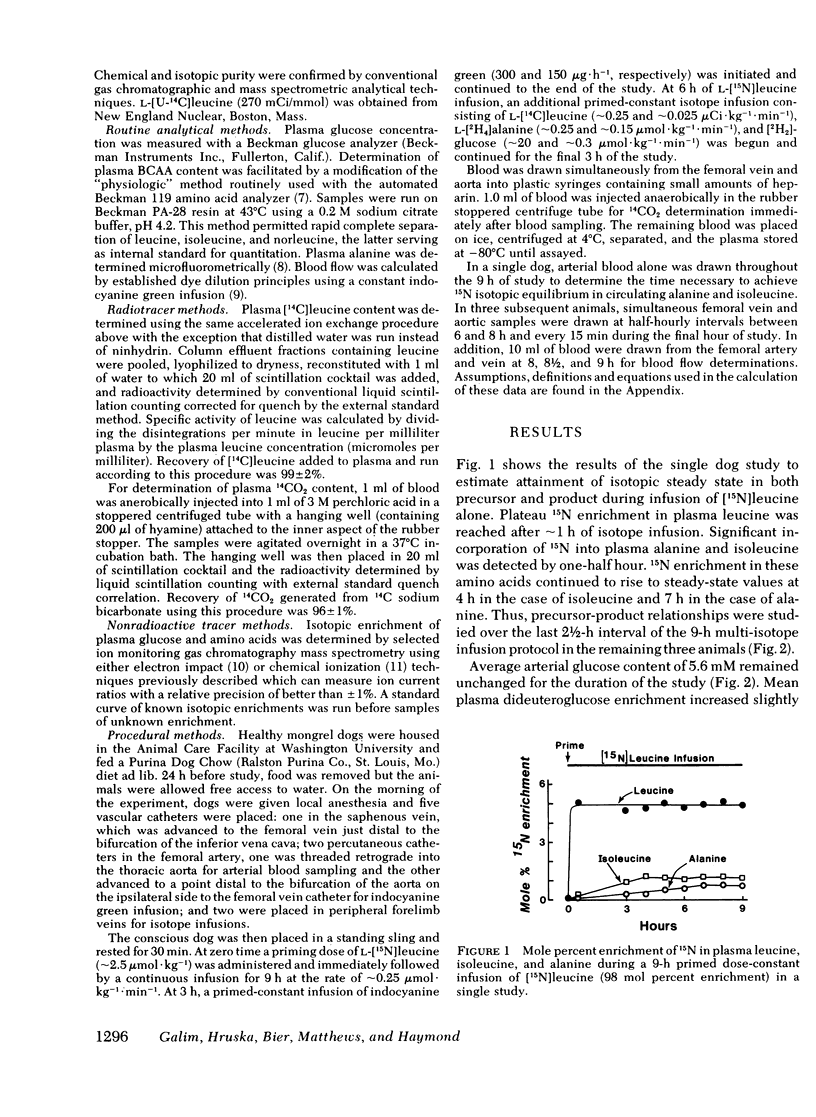
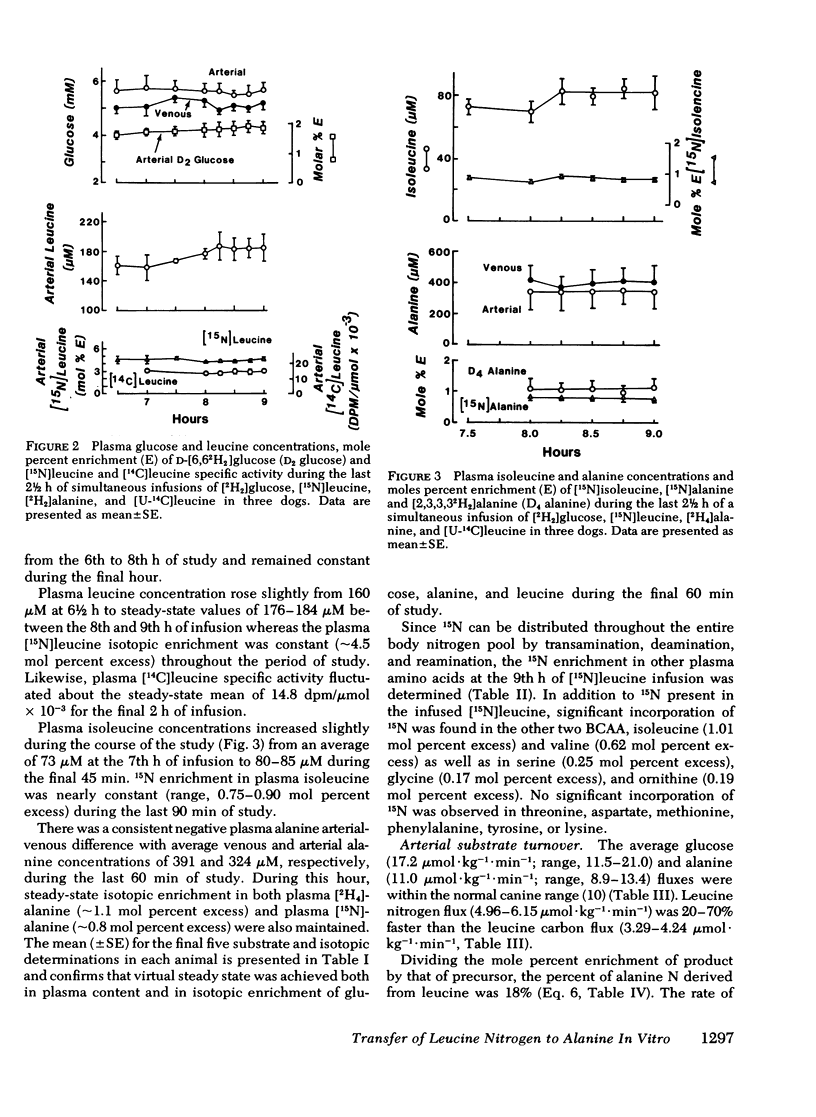
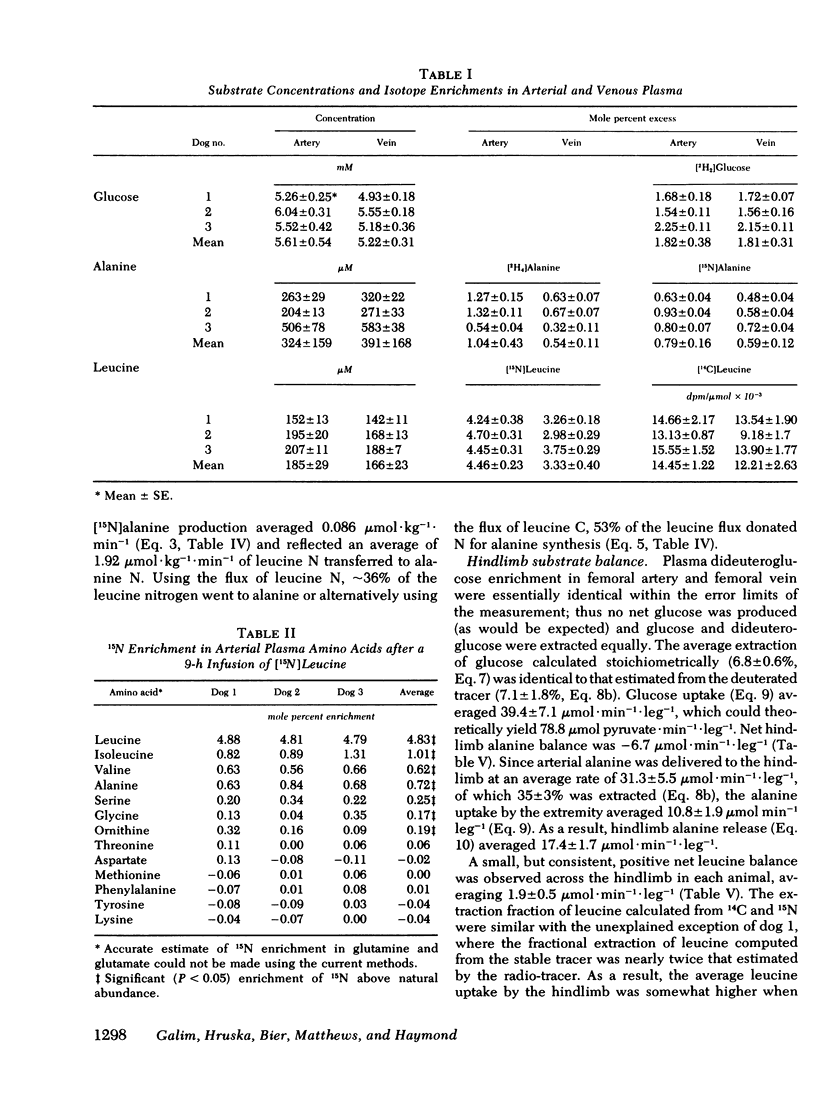
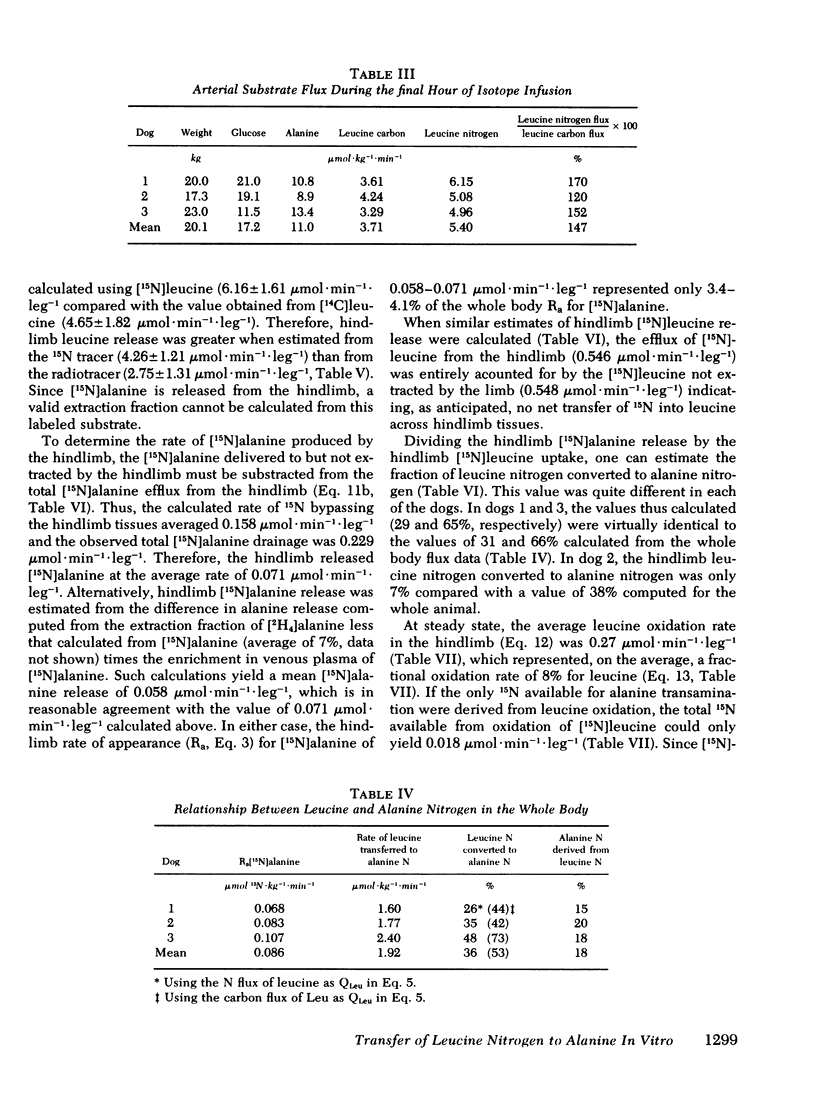
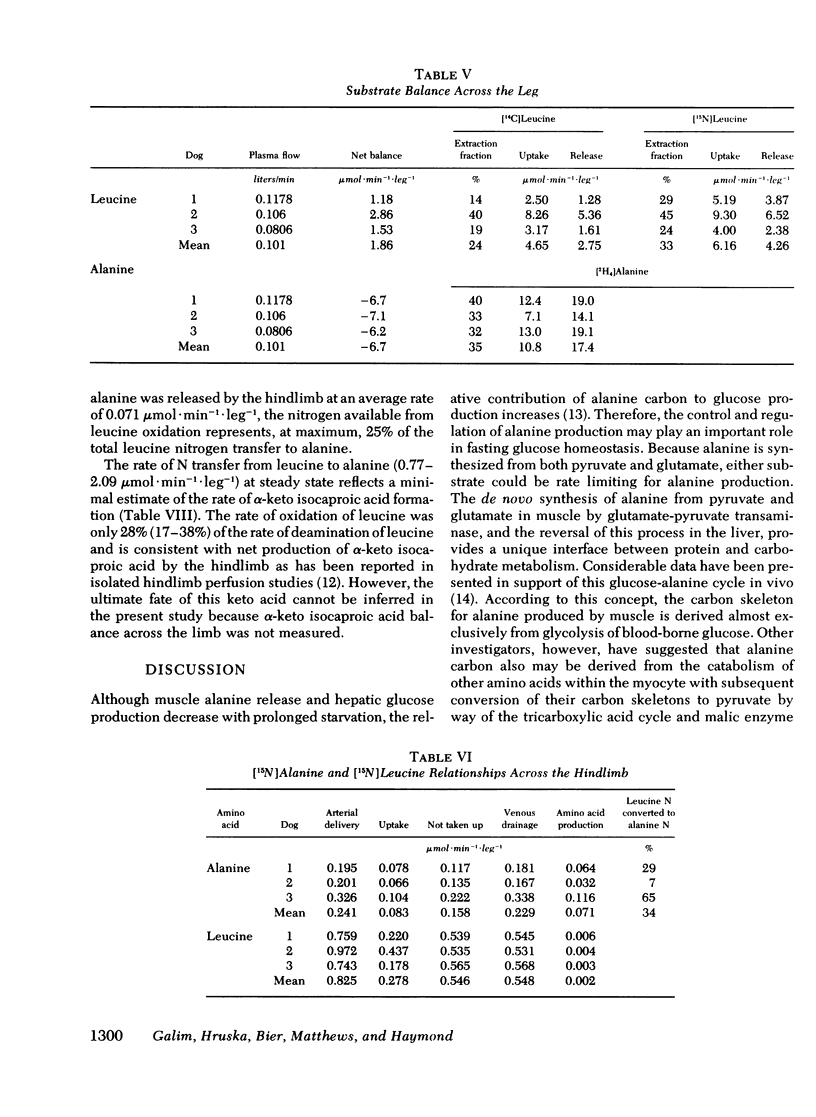
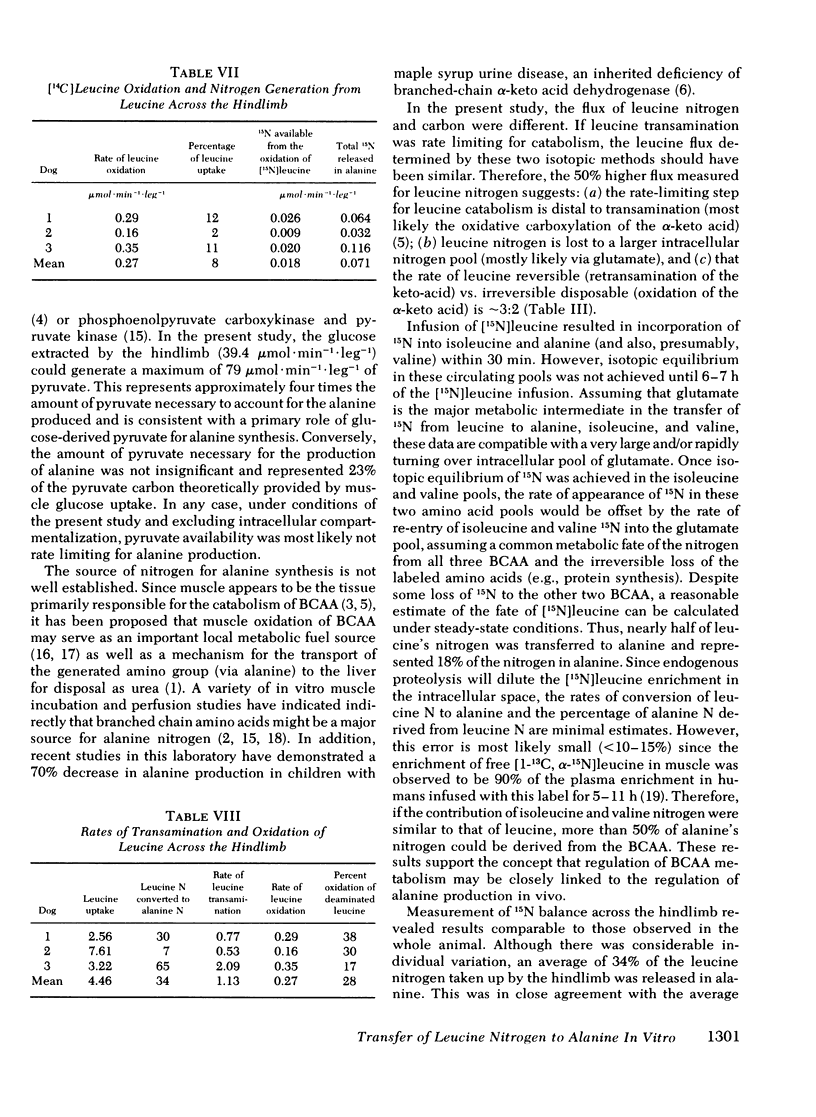
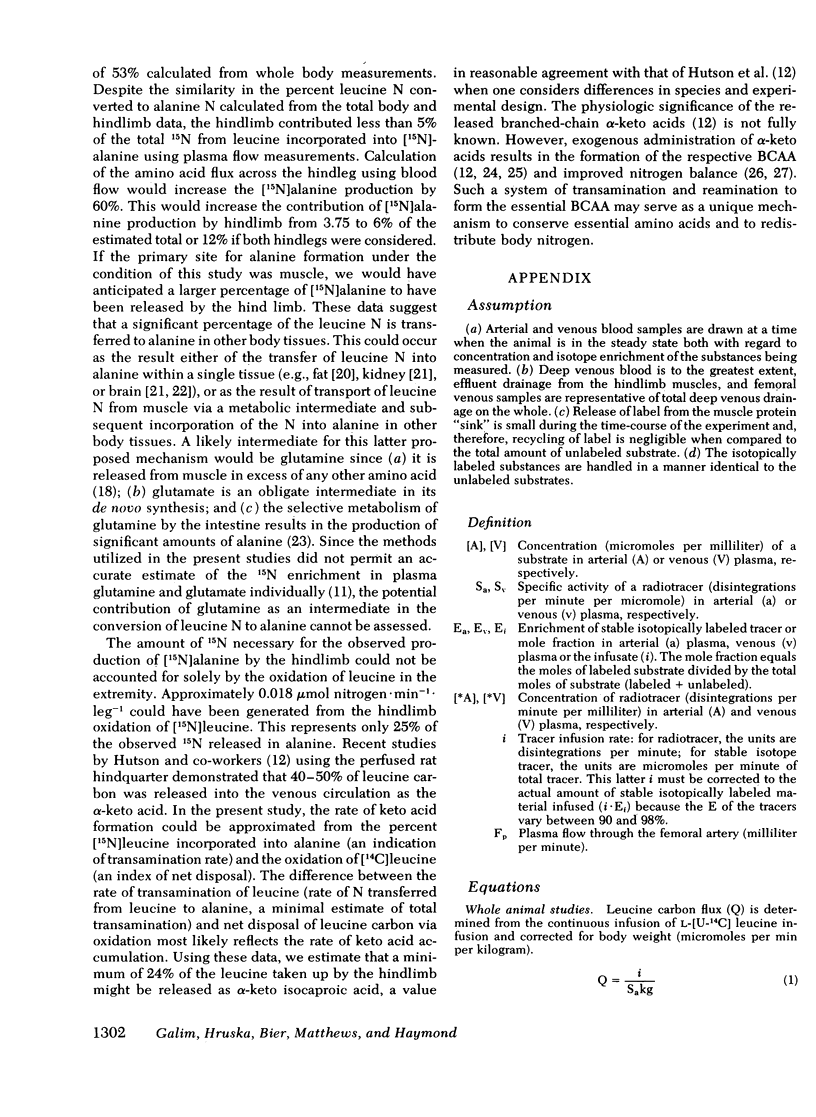
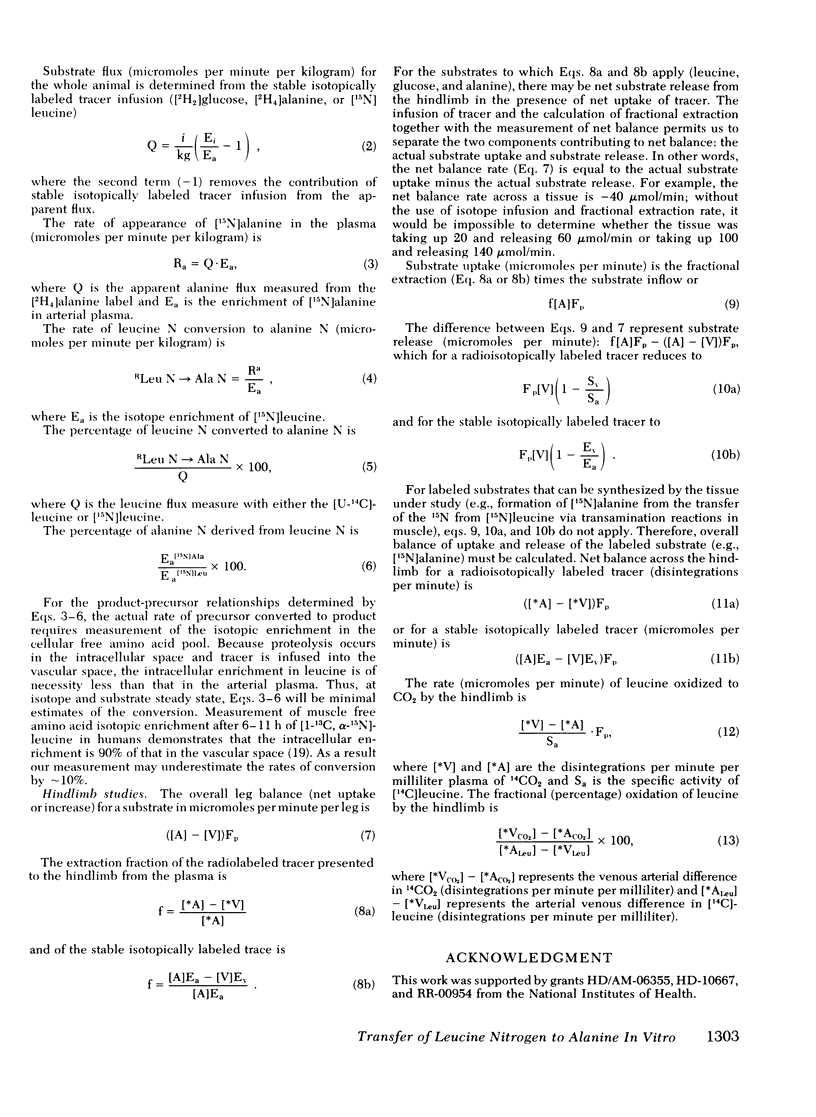
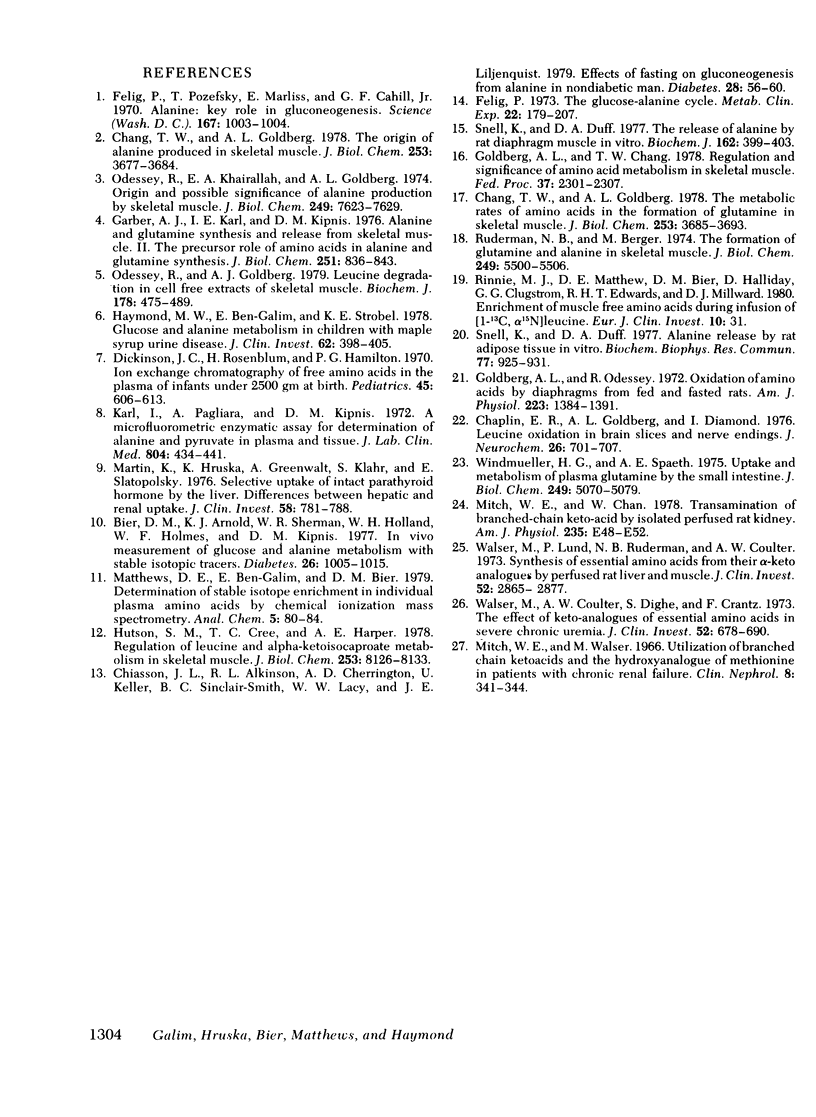
Selected References
These references are in PubMed. This may not be the complete list of references from this article.
- Bier D. M., Arnold K. J., Sherman W. R., Holland W. H., Holmes W. F., Kipnis D. M. In-vivo measurement of glucose and alanine metabolism with stable isotopic tracers. Diabetes. 1977 Nov;26(11):1005–1015. doi: 10.2337/diab.26.11.1005. [DOI] [PubMed] [Google Scholar]
- Chang T. W., Goldberg A. L. The metabolic fates of amino acids and the formation of glutamine in skeletal muscle. J Biol Chem. 1978 May 25;253(10):3685–3693. [PubMed] [Google Scholar]
- Chang T. W., Goldberg A. L. The origin of alanine produced in skeletal muscle. J Biol Chem. 1978 May 25;253(10):3677–3684. [PubMed] [Google Scholar]
- Chaplin E. R., Goldberg A. L., Diamond I. Leucine oxidation in brain slices and nerve endings. J Neurochem. 1976 Apr;26(4):701–707. doi: 10.1111/j.1471-4159.1976.tb04440.x. [DOI] [PubMed] [Google Scholar]
- Chiasson J. L., Atkinson R. L., Cherrington A. D., Keller U., Sinclair-Smith B. C., Lacy W. W., Liljenquist J. E. Effects of fasting on gluconeogenesis from alanine in nondiabetic man. Diabetes. 1979 Jan;28(1):56–60. doi: 10.2337/diab.28.1.56. [DOI] [PubMed] [Google Scholar]
- Dickinson J. C., Rosenblum H., Hamilton P. B. Ion exchange chromatography of the free amino acids in the plasma of infants under 2,500 gm at birth. Pediatrics. 1970 Apr;45(4):606–613. [PubMed] [Google Scholar]
- Felig P., Pozefsky T., Marliss E., Cahill G. F., Jr Alanine: key role in gluconeogenesis. Science. 1970 Feb 13;167(3920):1003–1004. doi: 10.1126/science.167.3920.1003. [DOI] [PubMed] [Google Scholar]
- Felig P. The glucose-alanine cycle. Metabolism. 1973 Feb;22(2):179–207. doi: 10.1016/0026-0495(73)90269-2. [DOI] [PubMed] [Google Scholar]
- Garber A. J., Karl I. E., Kipnis D. M. Alanine and glutamine synthesis and release from skeletal muscle. II. The precursor role of amino acids in alanine and glutamine synthesis. J Biol Chem. 1976 Feb 10;251(3):836–843. [PubMed] [Google Scholar]
- Goldberg A. L., Chang T. W. Regulation and significance of amino acid metabolism in skeletal muscle. Fed Proc. 1978 Jul;37(9):2301–2307. [PubMed] [Google Scholar]
- Goldberg A. L., Odessey R. Oxidation of amino acids by diaphragms from fed and fasted rats. Am J Physiol. 1972 Dec;223(6):1384–1391. doi: 10.1152/ajplegacy.1972.223.6.1384. [DOI] [PubMed] [Google Scholar]
- Haymond M. W., Ben-Galim E., Strobel K. E. Glucose and alanine metabolism in children with maple syrup urine disease. J Clin Invest. 1978 Aug;62(2):398–405. doi: 10.1172/JCI109141. [DOI] [PMC free article] [PubMed] [Google Scholar]
- Hutson S. M., Cree T. C., Harper A. E. Regulation of leucine and alpha-ketoisocaproate metabolism in skeletal muscle. J Biol Chem. 1978 Nov 25;253(22):8126–8133. [PubMed] [Google Scholar]
- Karl I. E., Pagliara A. S., Kipnis D. M. A microfluorometric enzymatic assay for the determination of alanine and pyruvate in plasma and tissues. J Lab Clin Med. 1972 Sep;80(3):434–441. [PubMed] [Google Scholar]
- Martin K., Hruska K., Greenwalt A., Klahr S., Slatopolsky E. Selective uptake of intact parathyroid hormone by the liver: differences between hepatic and renal uptake. J Clin Invest. 1976 Oct;58(4):781–788. doi: 10.1172/JCI108529. [DOI] [PMC free article] [PubMed] [Google Scholar]
- Matthews D. E., Ben-Galim E., Bier D. M. Determination of stable isotopic enrichment in individual plasma amino acids by chemical ionization mass spectrometry. Anal Chem. 1979 Jan;51(1):80–84. doi: 10.1021/ac50037a028. [DOI] [PubMed] [Google Scholar]
- Mitch W. E., Chan W. Transamination of branched-chain keto acids by isolated perfused rat kidney. Am J Physiol. 1978 Jul;235(1):E47–E52. doi: 10.1152/ajpendo.1978.235.1.E47. [DOI] [PubMed] [Google Scholar]
- Mitch W., Walser M. Nitrogen balance of uremic patients receiving branched-chain ketoacids and the hydroxy-analogue of methionine as substitutes for the respective amino acids. Clin Nephrol. 1977 Aug;8(2):341–344. [PubMed] [Google Scholar]
- Odessey R., Goldberg A. L. Leucine degradation in cell-free extracts of skeletal muscle. Biochem J. 1979 Feb 15;178(2):475–489. doi: 10.1042/bj1780475. [DOI] [PMC free article] [PubMed] [Google Scholar]
- Odessey R., Khairallah E. A., Goldberg A. L. Origin and possible significance of alanine production by skeletal muscle. J Biol Chem. 1974 Dec 10;249(23):7623–7629. [PubMed] [Google Scholar]
- Ruderman N. B., Berger M. The formation of glutamine and alanine in skeletal muscle. J Biol Chem. 1974 Sep 10;249(17):5500–5506. [PubMed] [Google Scholar]
- Snell K., Duff D. A. Alanine release by rat adipose tissue in vitro. Biochem Biophys Res Commun. 1977 Aug 8;77(3):925–931. doi: 10.1016/s0006-291x(77)80066-1. [DOI] [PubMed] [Google Scholar]
- Snell K., Duff D. A. The release of alanine by rat diaphragm muscle in vitro. Biochem J. 1977 Feb 15;162(2):399–403. doi: 10.1042/bj1620399. [DOI] [PMC free article] [PubMed] [Google Scholar]
- Walser M., Coulter A. W., Dighe S., Crantz F. R. The effect of keto-analogues of essential amino acids in severe chronic uremia. J Clin Invest. 1973 Mar;52(3):678–690. doi: 10.1172/JCI107229. [DOI] [PMC free article] [PubMed] [Google Scholar]
- Walser M., Lund P., Ruderman N. B., Coulter A. W. Synthesis of essential amino acids from their alpha-keto analogues by perfused rat liver and muscle. J Clin Invest. 1973 Nov;52(11):2865–2877. doi: 10.1172/JCI107483. [DOI] [PMC free article] [PubMed] [Google Scholar]
- Windmueller H. G., Spaeth A. E. Uptake and metabolism of plasma glutamine by the small intestine. J Biol Chem. 1974 Aug 25;249(16):5070–5079. [PubMed] [Google Scholar]