Abstract
Propionic and methylmalonic acidemia are both known to be associated with hyperammonemia. Rats injected with 10 or 20 mmol/kg of propionate or 20 mmol/kg of methylmalonate, along with 1.5 g/kg of a mixture of amino acids, developed severe hyperammonemia, whereas rats administered the same dosages of acetate did not. In vitro, neither propionyl nor methylmalonyl CoA affected the activity of carbamyl phosphate synthetase I, ornithine transcarbamylase, nor the activation constant (KA) of carbamyl phosphate synthetase I for N-acetyl glutamate. Furthermore, rats injected with propionate showed no alteration of liver amino acid concentrations, which could explain impaired ureagenesis. Animals injected with methylmalonate showed an increase in both citrulline and aspartate, suggesting that argininosuccinic acid synthetase may also have been inhibited. Liver ATP levels were unchanged. Citrullinogenesis, measured in intact mitochondria from livers of injected animals, was reduced 20-25% by 20 mmol/kg of propionate or methylmalonate (compared with acetate). This effect was attributable to an impairment in the normal rise of liver N-acetyl glutamate content after amino acid injection. Thus, carbamyl phosphate synthetase I activation was reduced. Liver levels of acetyl CoA and free CoA were reduced. Levels of unidentified acyl CoA derivatives rose, presumably reflecting the accumulation of propionyl and methylmalonyl CoA. Thus, the principal mechanism for hyperammonemia induced by these acids is depletion of liver N-acetyl glutamate, which is in turn attributable to depletion of acetyl CoA and/or competitive inhibition by propionyl and methylmalonyl CoA of N-acetyl glutamate synthetase. Injection of methylmalonate may also have an additional inhibitory effect on argininosuccinic acid synthetase.
Full text
PDF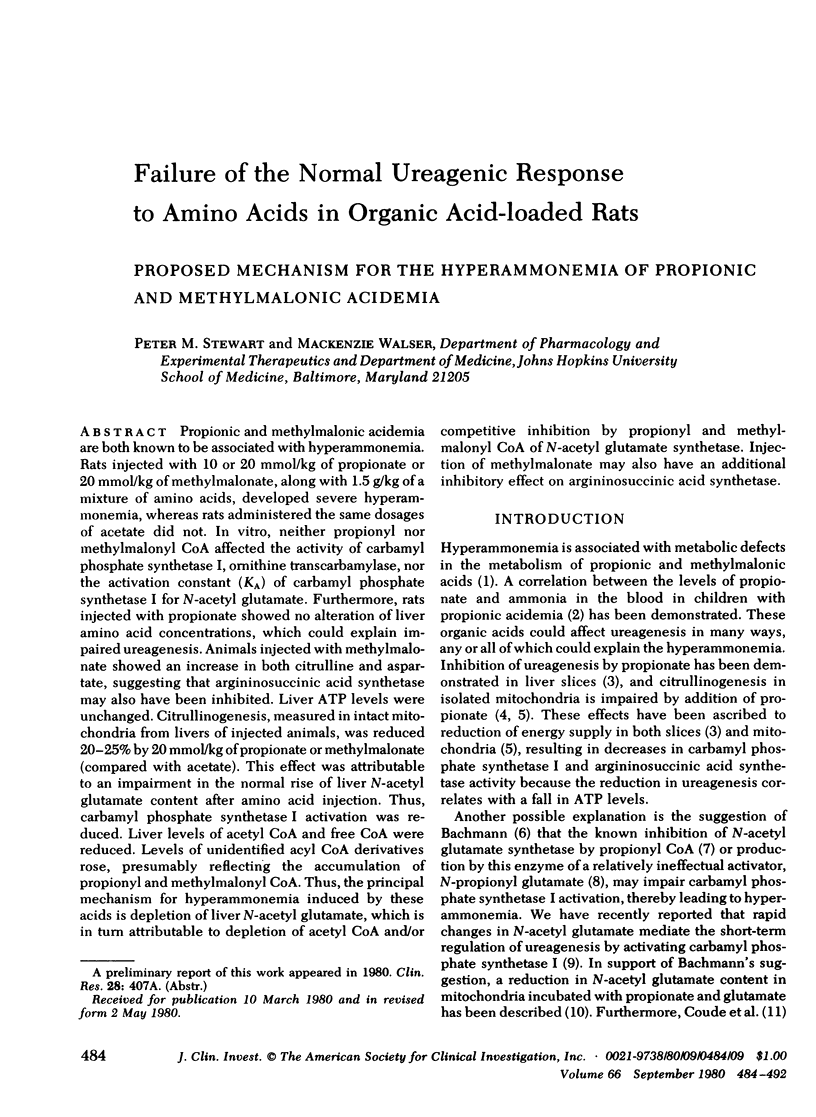
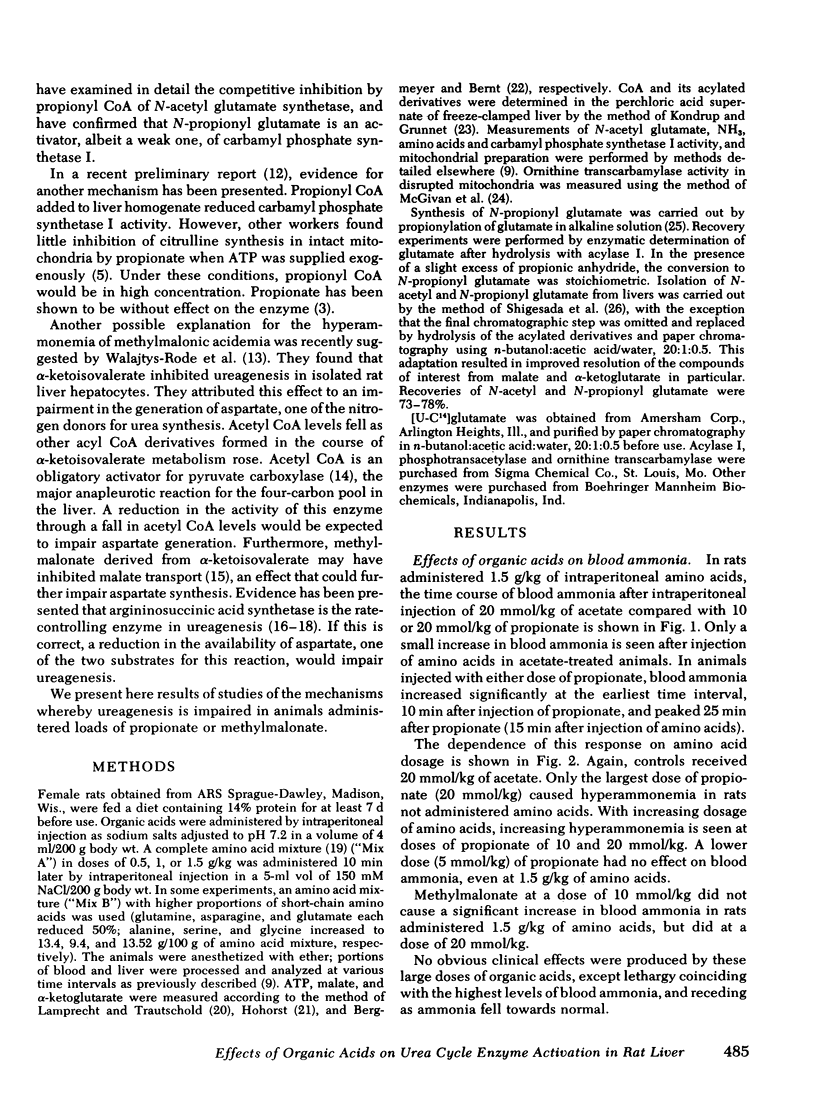
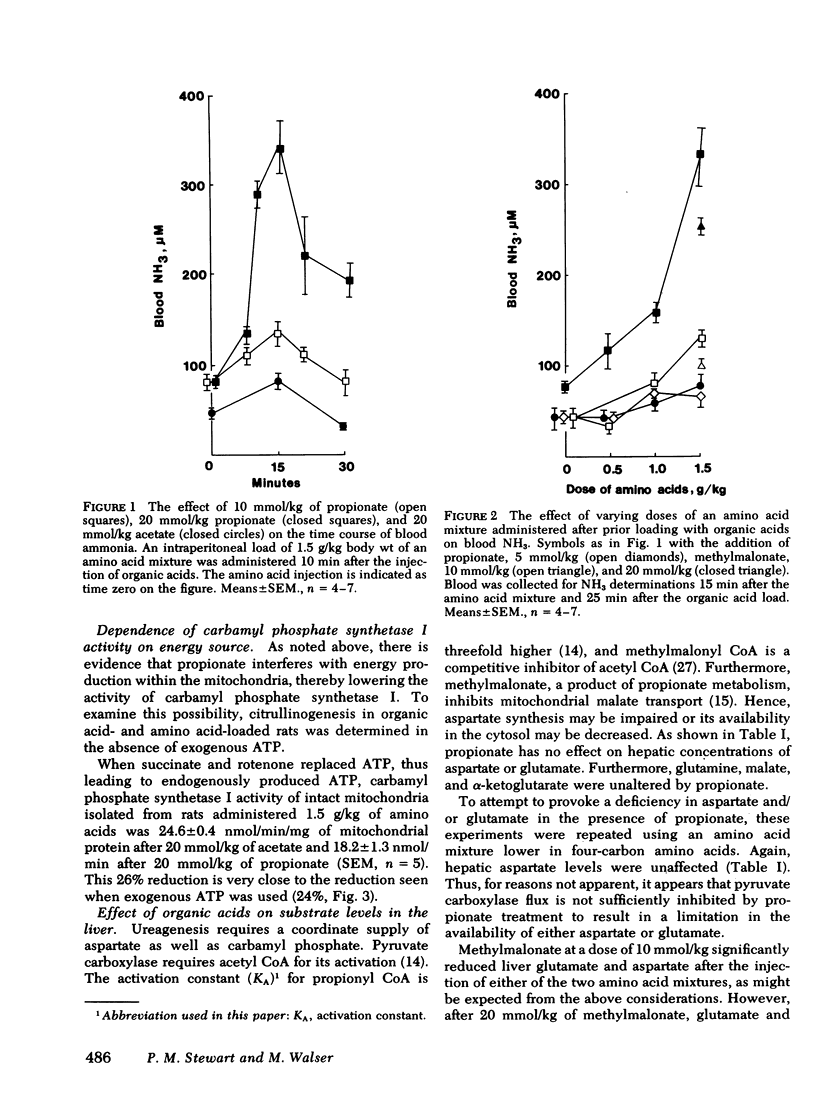
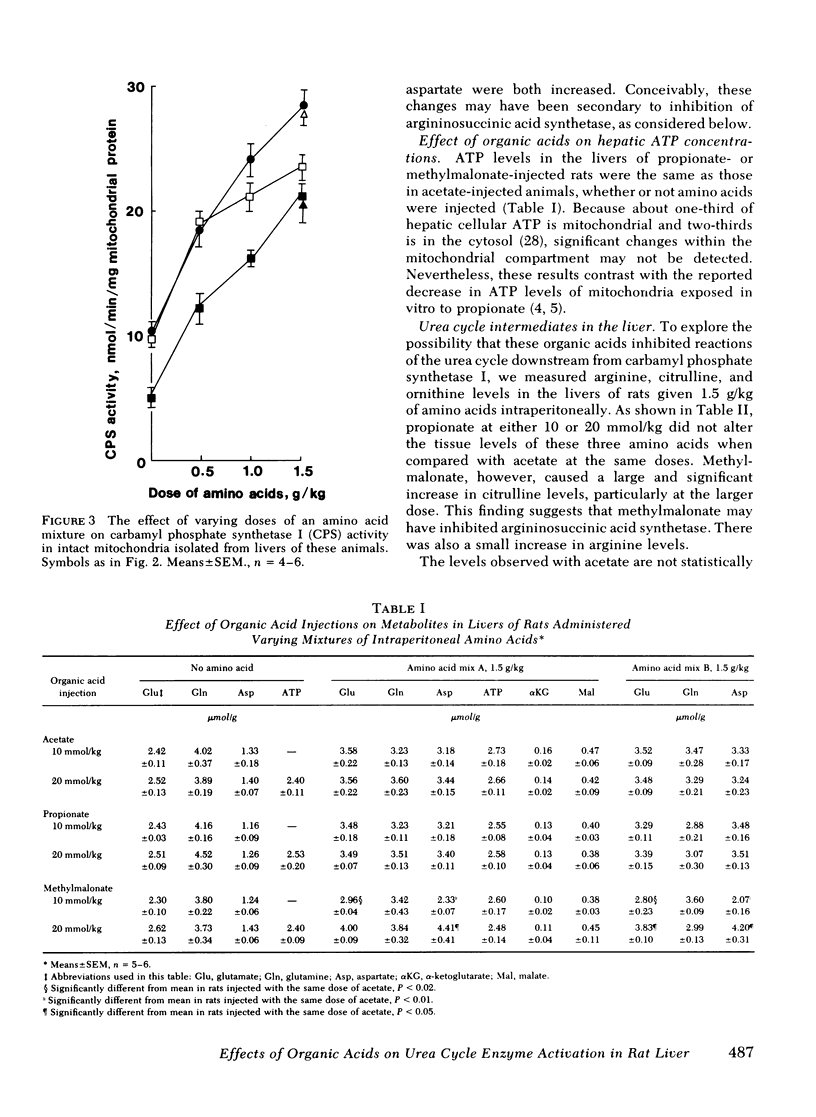
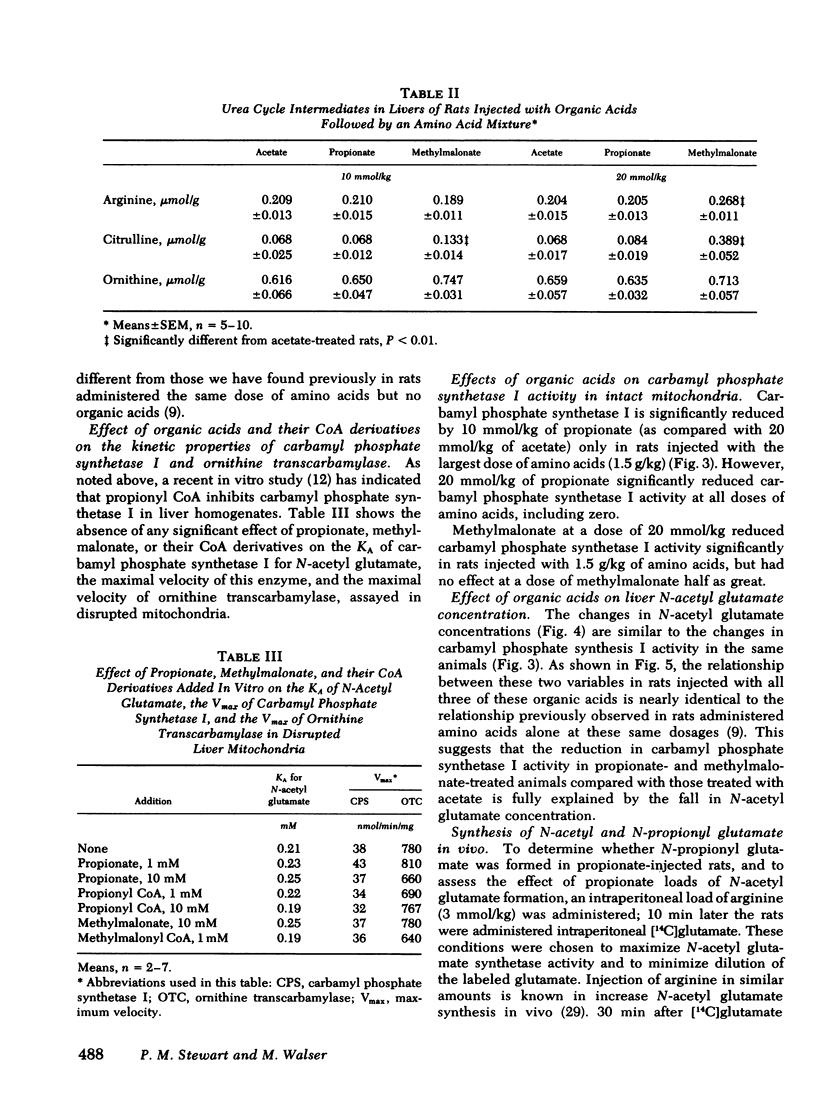
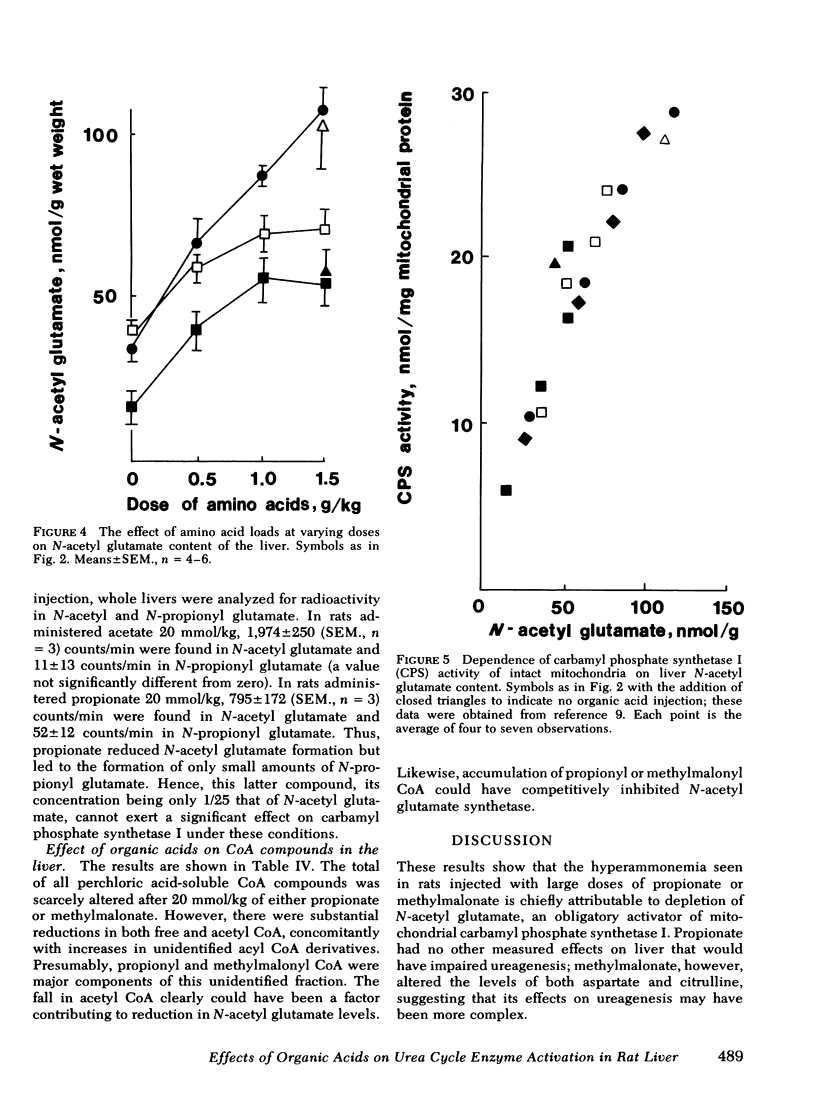
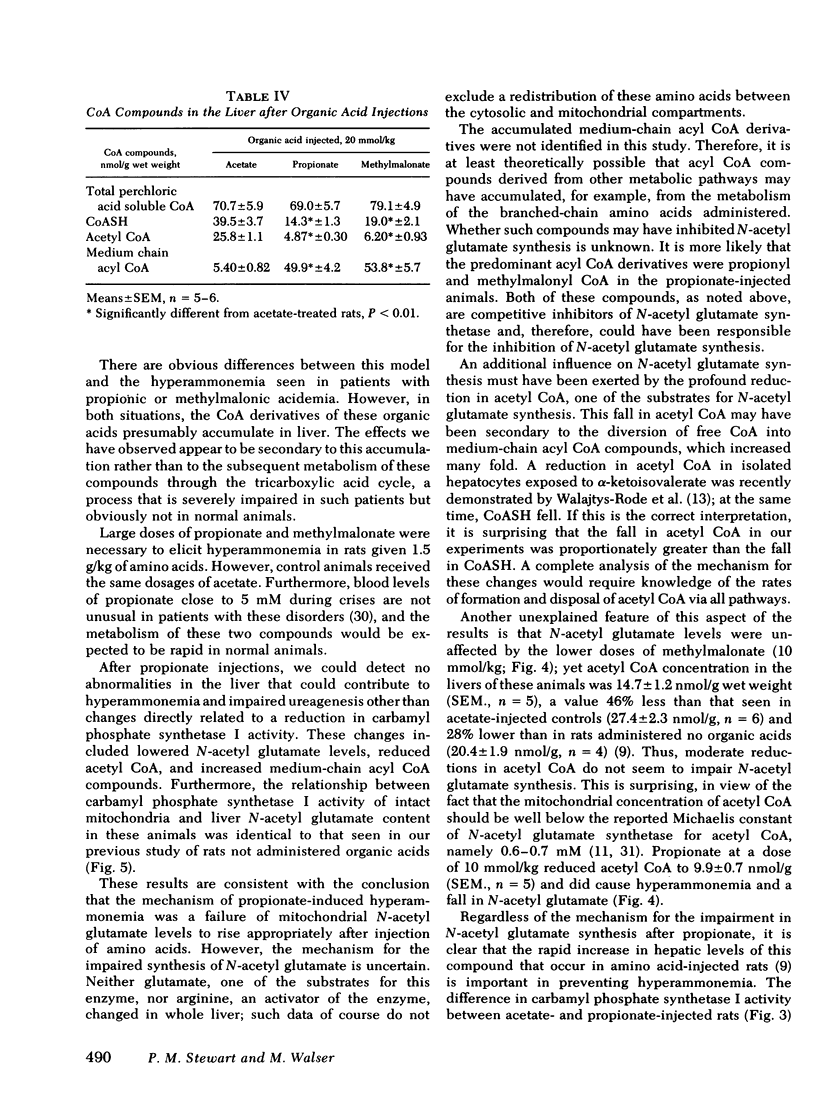
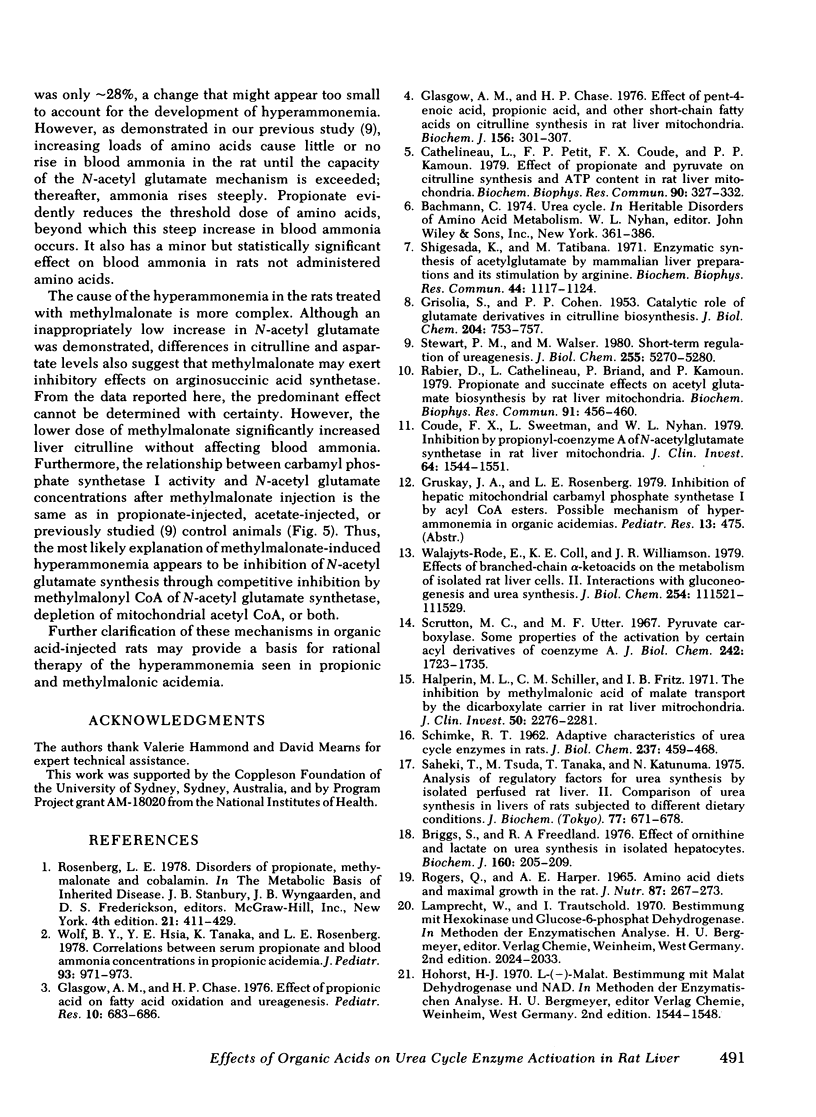
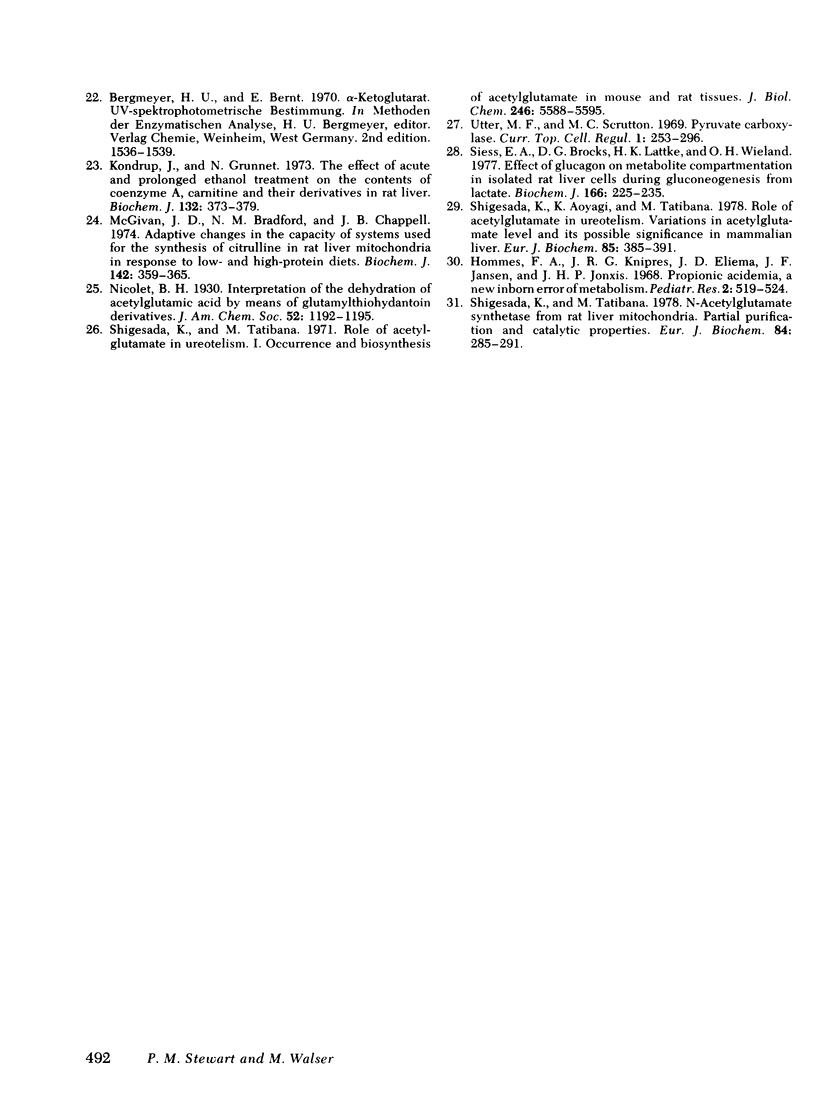
Selected References
These references are in PubMed. This may not be the complete list of references from this article.
- Briggs S., Freedland R. A. Effect of ornithine and lactate on urea synthesis in isolated hepatocytes. Biochem J. 1976 Nov 15;160(2):205–209. doi: 10.1042/bj1600205. [DOI] [PMC free article] [PubMed] [Google Scholar]
- Cathelineau L., Petit F. P., Coudé F. X., Kamoun P. P. Effect of propionate and pyruvate on citrulline synthesis and ATP content in rat liver mitochondria. Biochem Biophys Res Commun. 1979 Sep 12;90(1):327–332. doi: 10.1016/0006-291x(79)91628-0. [DOI] [PubMed] [Google Scholar]
- Coude F. X., Sweetman L., Nyhan W. L. Inhibition by propionyl-coenzyme A of N-acetylglutamate synthetase in rat liver mitochondria. A possible explanation for hyperammonemia in propionic and methylmalonic acidemia. J Clin Invest. 1979 Dec;64(6):1544–1551. doi: 10.1172/JCI109614. [DOI] [PMC free article] [PubMed] [Google Scholar]
- GRISOLIA S., COHEN P. P. Catalytic rôle of of glutamate derivatives in citrulline biosynthesis. J Biol Chem. 1953 Oct;204(2):753–757. [PubMed] [Google Scholar]
- Glasgow A. M., Chase H. P. Effect of pent-4-enoic acid, propionic acid and other short-chain fatty acids on citrulline synthesis in rat liver mitochondria. Biochem J. 1976 May 15;156(2):301–307. doi: 10.1042/bj1560301. [DOI] [PMC free article] [PubMed] [Google Scholar]
- Glasgow A. M., Chase H. P. Effect of propionic acid on fatty acid oxidation and ureagenesis. Pediatr Res. 1976 Jul;10(7):683–686. doi: 10.1203/00006450-197607000-00010. [DOI] [PubMed] [Google Scholar]
- Halperin M. L., Schiller C. M., Fritz I. B. The inhibition by methylmalonic acid of malate transport by the dicarboxylate carrier in rat liver mitochondria. A possible explantation for hypoglycemia in methylmalonic aciduria. J Clin Invest. 1971 Nov;50(11):2276–2282. doi: 10.1172/JCI106725. [DOI] [PMC free article] [PubMed] [Google Scholar]
- Hommes F. A., Kuipers J. R., Elema J. D., Jansen J. F., Jonxis J. H. Propionicacidemia, a new inborn error of metabolism. Pediatr Res. 1968 Nov;2(6):519–524. doi: 10.1203/00006450-196811000-00010. [DOI] [PubMed] [Google Scholar]
- Kondrup J., Grunnet N. The effect of acute and prolonged ethanol treatment on the contents of coenzyme A, carnitine and their derivatives in rat liver. Biochem J. 1973 Mar;132(3):373–379. doi: 10.1042/bj1320373. [DOI] [PMC free article] [PubMed] [Google Scholar]
- McGivan J. D., Bradford N. M., Chappell J. B. Adaptive changes in the capacity of systems used for the synthesis of citrulline in rat liver mitochondria in response to high- and-low-protein diets. Biochem J. 1974 Aug;142(2):359–364. doi: 10.1042/bj1420359. [DOI] [PMC free article] [PubMed] [Google Scholar]
- Rabier D., Cathelineau L., Briand P., Kamoun P. Propionate and succinate effects on acetyl glutamate biosynthesis by rat liver mitochondria. Biochem Biophys Res Commun. 1979 Nov 28;91(2):456–460. doi: 10.1016/0006-291x(79)91543-2. [DOI] [PubMed] [Google Scholar]
- Rogers Q. R., Harper A. E. Amino acid diets and maximal growth in the rat. J Nutr. 1965 Nov;87(3):267–273. doi: 10.1093/jn/87.3.267. [DOI] [PubMed] [Google Scholar]
- SCHIMKE R. T. Adaptive characteristics of urea cycle enzymes in the rat. J Biol Chem. 1962 Feb;237:459–468. [PubMed] [Google Scholar]
- Saheki T., Tsuda M., Tanaka T., katunuma N. Analysis of regulatory factors for urea synthesis by isolated perfused rat liver. II. Comparison of urea synthesis in livers of rats subjected to different dietary conditions. J Biochem. 1975 Mar;77(3):671–678. doi: 10.1093/oxfordjournals.jbchem.a130769. [DOI] [PubMed] [Google Scholar]
- Scrutton M. C., Utter M. F. Pyruvate carboxylase. IX. Some properties of the activation by certain acyl derivatives of coenzyme A. J Biol Chem. 1967 Apr 25;242(8):1723–1735. [PubMed] [Google Scholar]
- Shigesada K., Aoyagi K., Tatibana M. Role of acetylglutamate in ureotelism. Variations in acetylglutamate level and its possible significance in control of urea synthesis in mammalian liver. Eur J Biochem. 1978 Apr 17;85(2):385–391. doi: 10.1111/j.1432-1033.1978.tb12250.x. [DOI] [PubMed] [Google Scholar]
- Shigesada K., Tatibana M. Enzymatic synthesis of acetylglutamate by mammalian liver preparations and its stimulation by arginine. Biochem Biophys Res Commun. 1971 Sep;44(5):1117–1124. doi: 10.1016/s0006-291x(71)80201-2. [DOI] [PubMed] [Google Scholar]
- Shigesada K., Tatibana M. N-Acetylglutamate synthetase from rat-liver mitochondria. Partial purification and catalytic properties. Eur J Biochem. 1978 Mar;84(1):285–291. doi: 10.1111/j.1432-1033.1978.tb12167.x. [DOI] [PubMed] [Google Scholar]
- Shigesada K., Tatibana M. Role of acetylglutamate in ureotelism. I. Occurrence and biosynthesis of acetylglutamate in mouse and rat tissues. J Biol Chem. 1971 Sep 25;246(18):5588–5595. [PubMed] [Google Scholar]
- Siess E. A., Brocks D. G., Lattke H. K., Wieland O. H. Effect of glucagon on metabolite compartmentation in isolated rat liver cells during gluconeogenesis from lactate. Biochem J. 1977 Aug 15;166(2):225–235. doi: 10.1042/bj1660225. [DOI] [PMC free article] [PubMed] [Google Scholar]
- Stewart P. M., Walser M. Short term regulation of ureagenesis. J Biol Chem. 1980 Jun 10;255(11):5270–5280. [PubMed] [Google Scholar]