Abstract
The state of intracellular water has been a matter of controversy for a long time for two reasons. First, experiments have often given conflicting results. Second, hitherto, there have been no plausible grounds for assuming that intracellular water should be significantly different from bulk water. A collective behavior of water molecules is suggested here as a thermodynamically inevitable mechanism for generation of appreciable zones of abnormal water. At a highly charged surface, water molecules move together, generating a zone of water perhaps 6 nm thick, which is weakly hydrogen bonded, fluid, and reactive and selectively accumulates small cations, multivalent anions, and hydrophobic solutes. At a hydrophobic surface, molecules move apart and local water becomes strongly bonded, inert, and viscous and accumulates large cations, univalent anions, and compatible solutes. Proteins and many other biopolymers have patchy surfaces which therefore induce, by the two mechanisms described, patchy interfacial water structures, which extended appreciable distances from the surface. The reason for many conflicting experimental results now becomes apparent. Average values of properties of water measured in gels, cells, or solutions of proteins are often not very different from the same properties of normal water, giving no indication that they are averages of extreme values. To detect the operation of this phenomenon, it is necessary to probe selectively a single abnormal population. Examples of such experiments are given. It is shown that this collective behavior of water molecules amounts to a considerable biological force, which can be equivalent to a pressure of 1,000 atm (1.013 x 10(5) kPa). It is suggested that cells selectively accumulate K+ ions and compatible solutes to avoid extremes of water structure in their aqueous compartments, but that cation pumps and other enzymes exploit the different solvent properties and reactivities of water to perform work of transport or synthesis.
Full text
PDF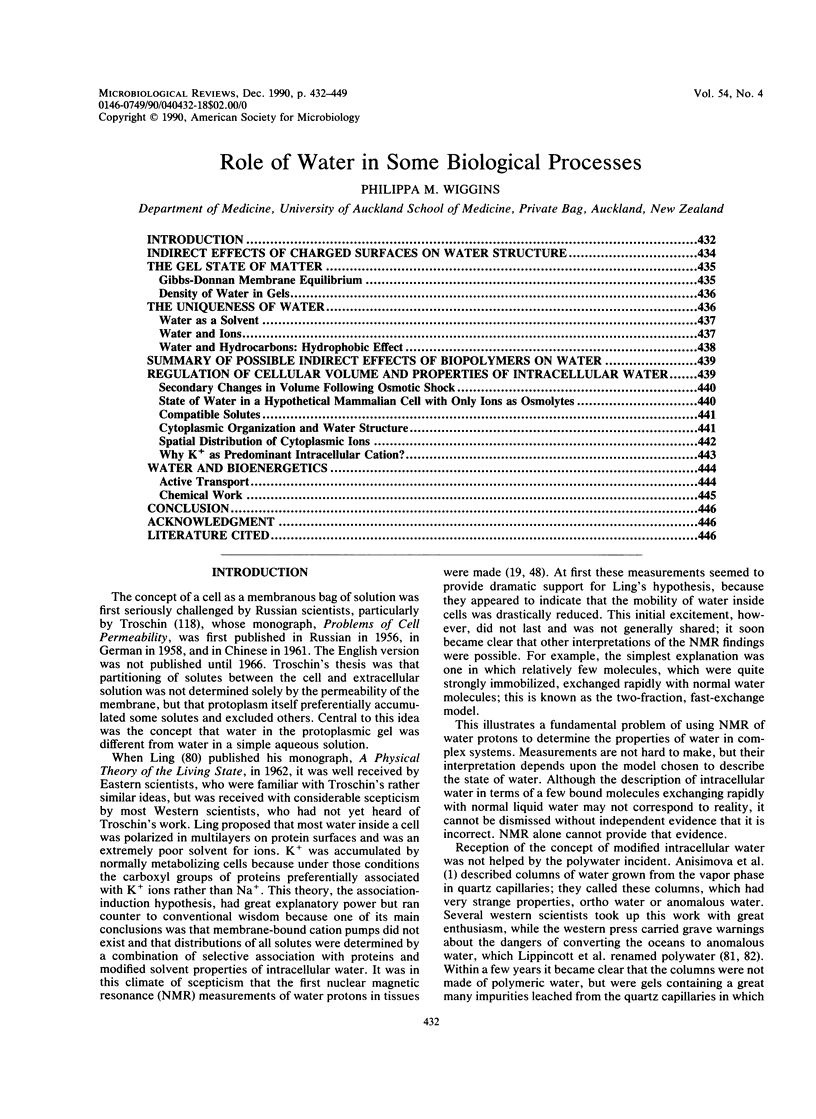
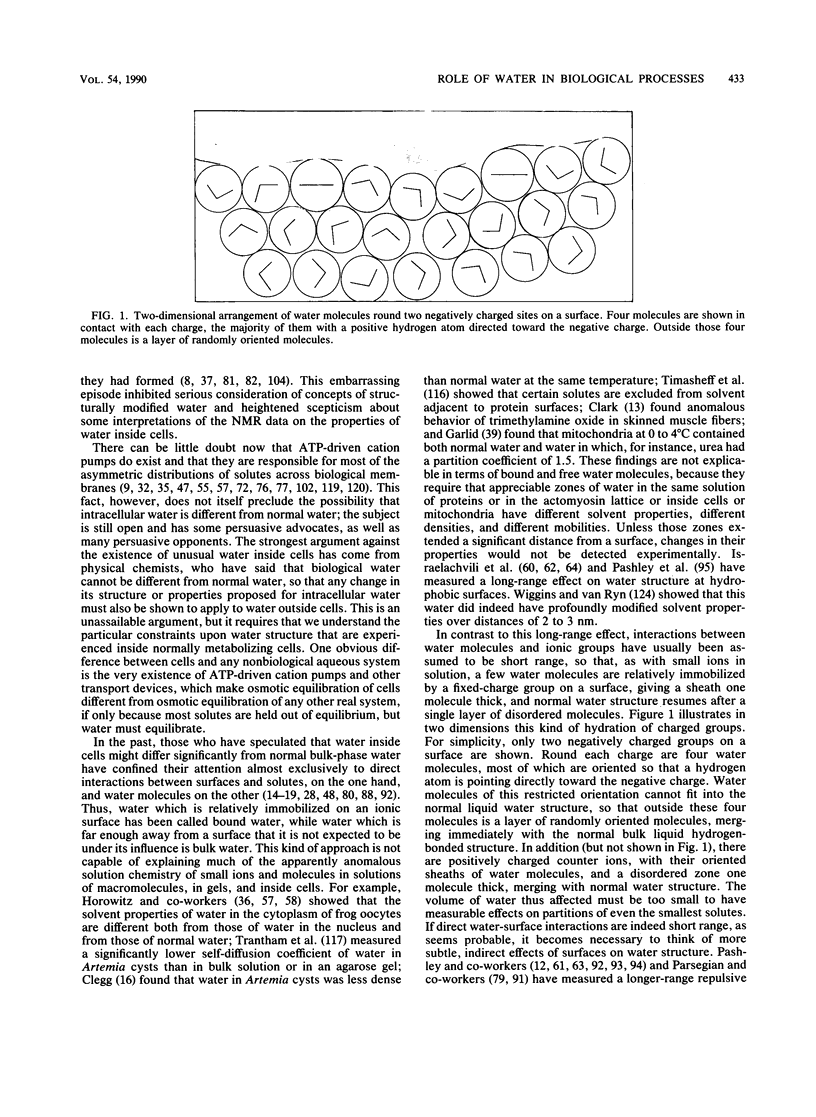
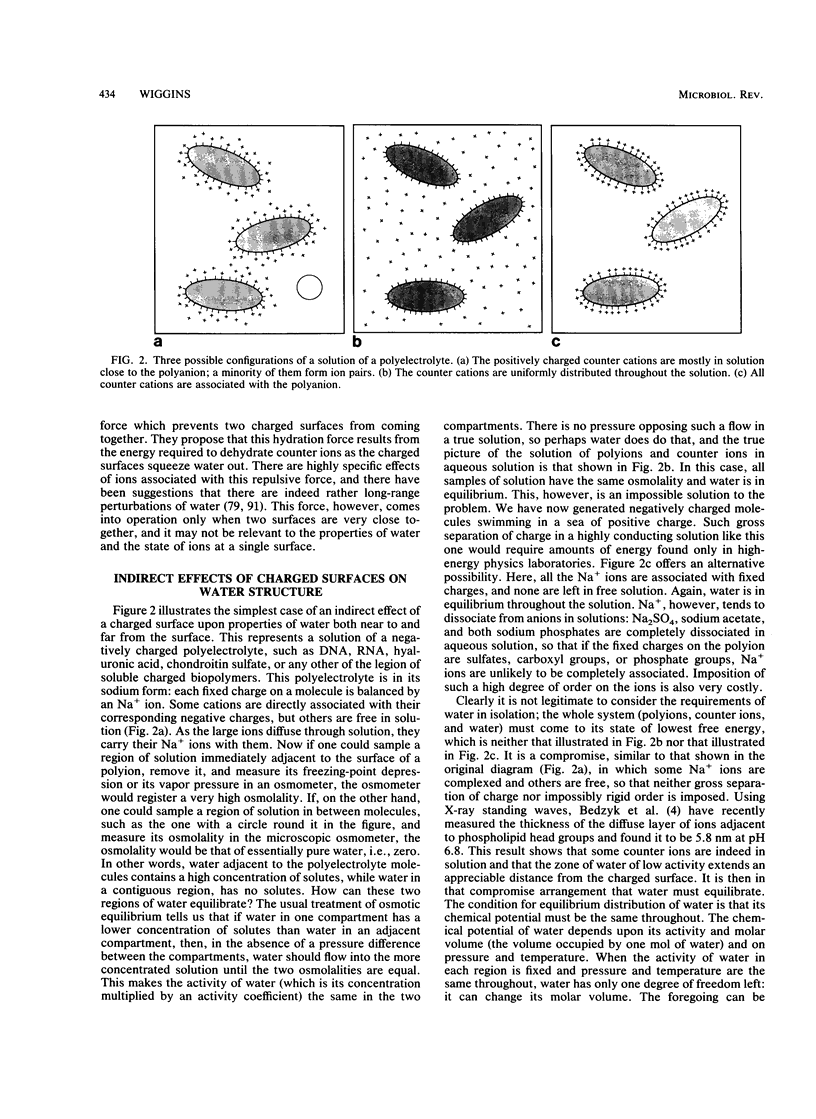
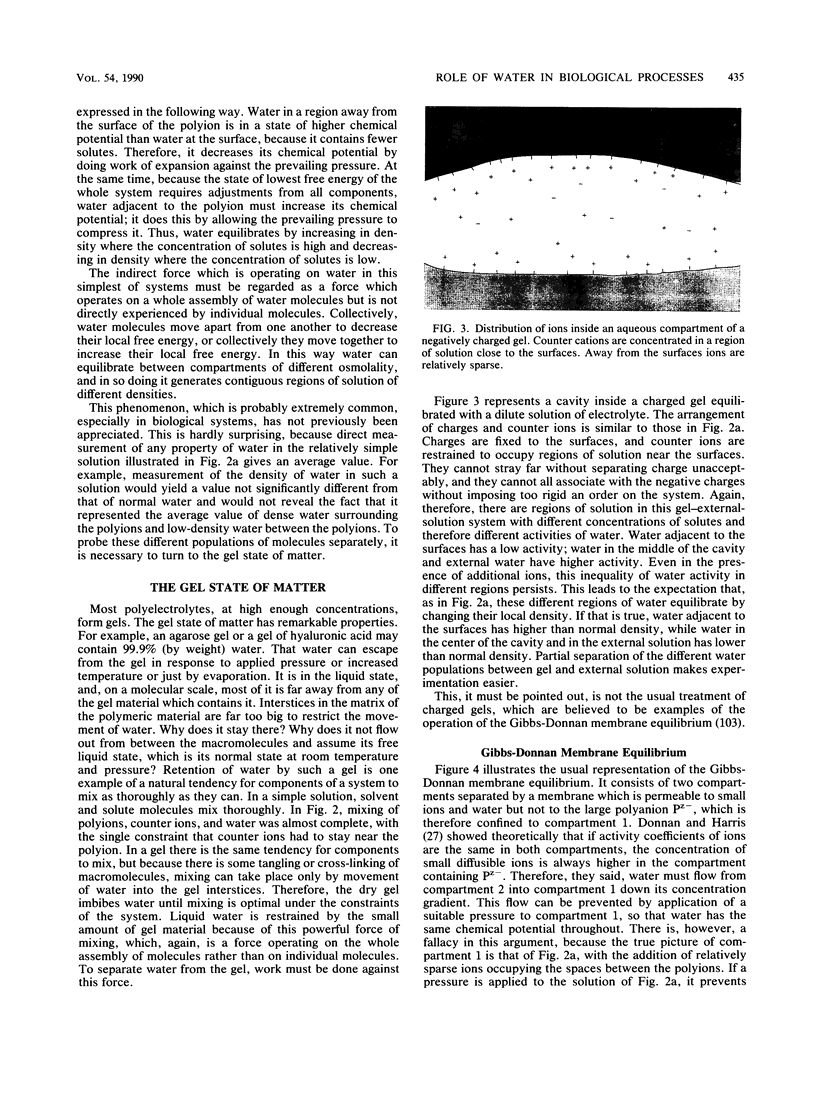
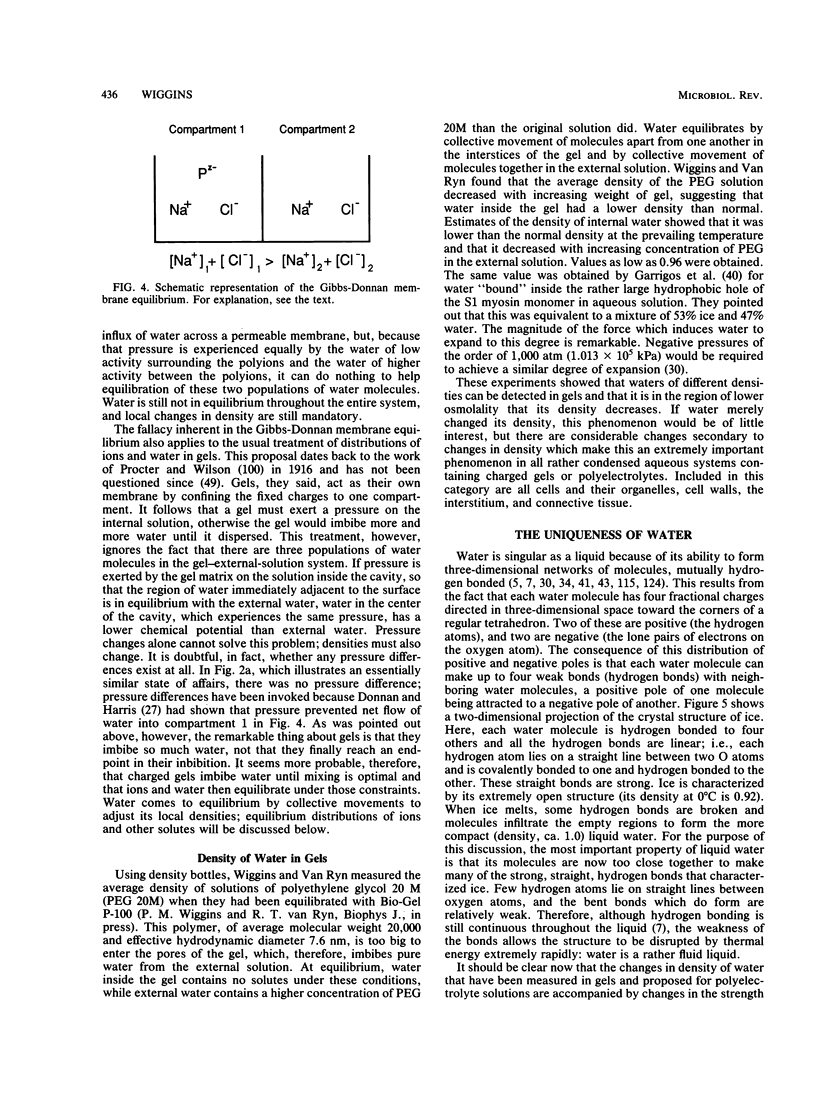
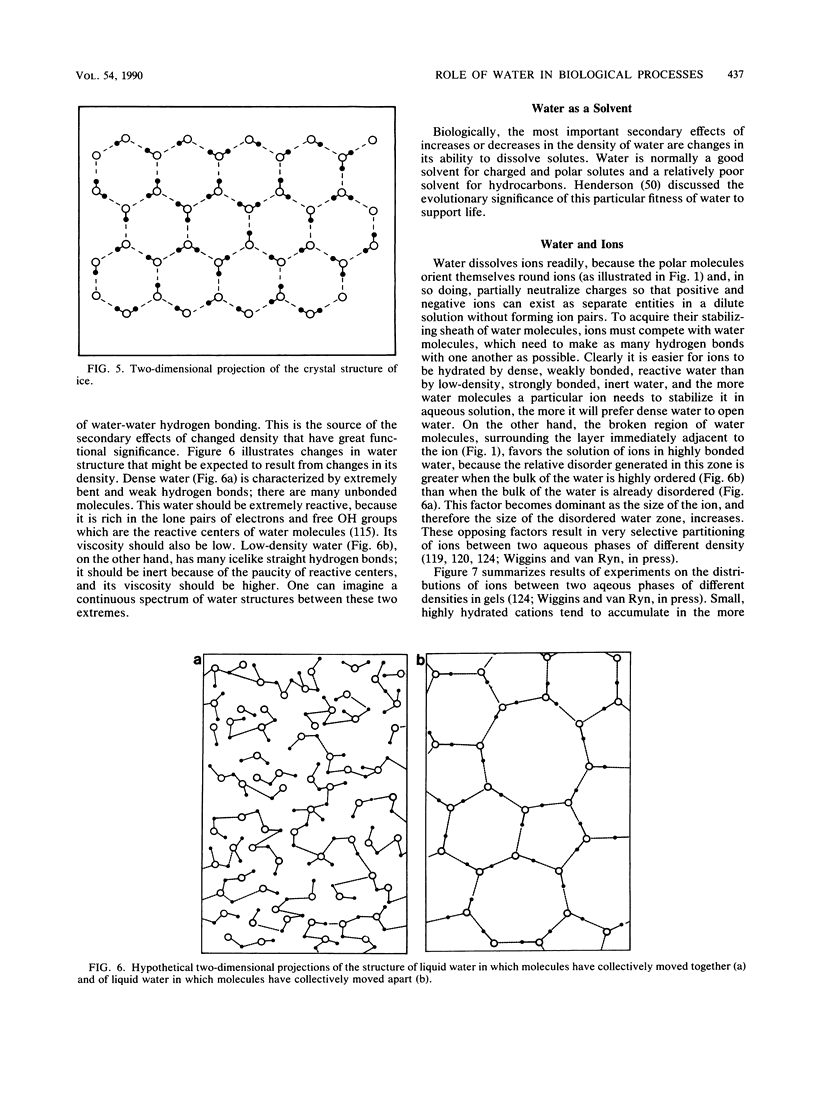
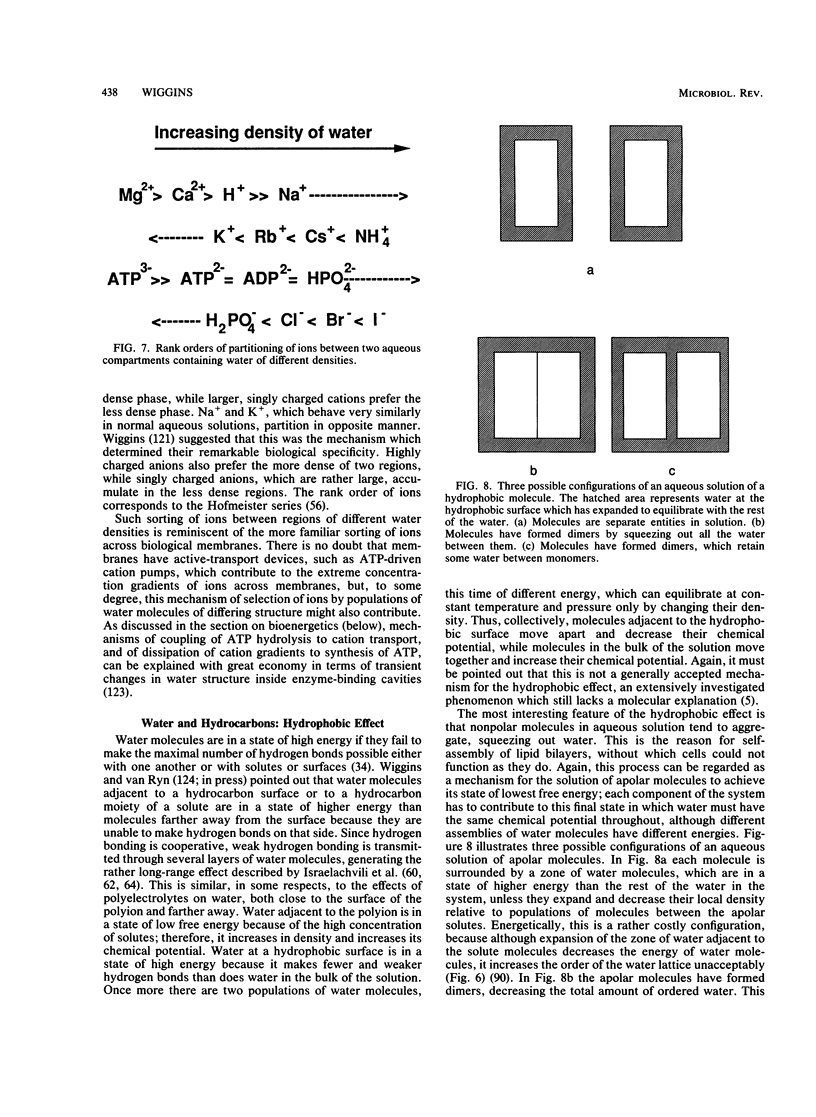
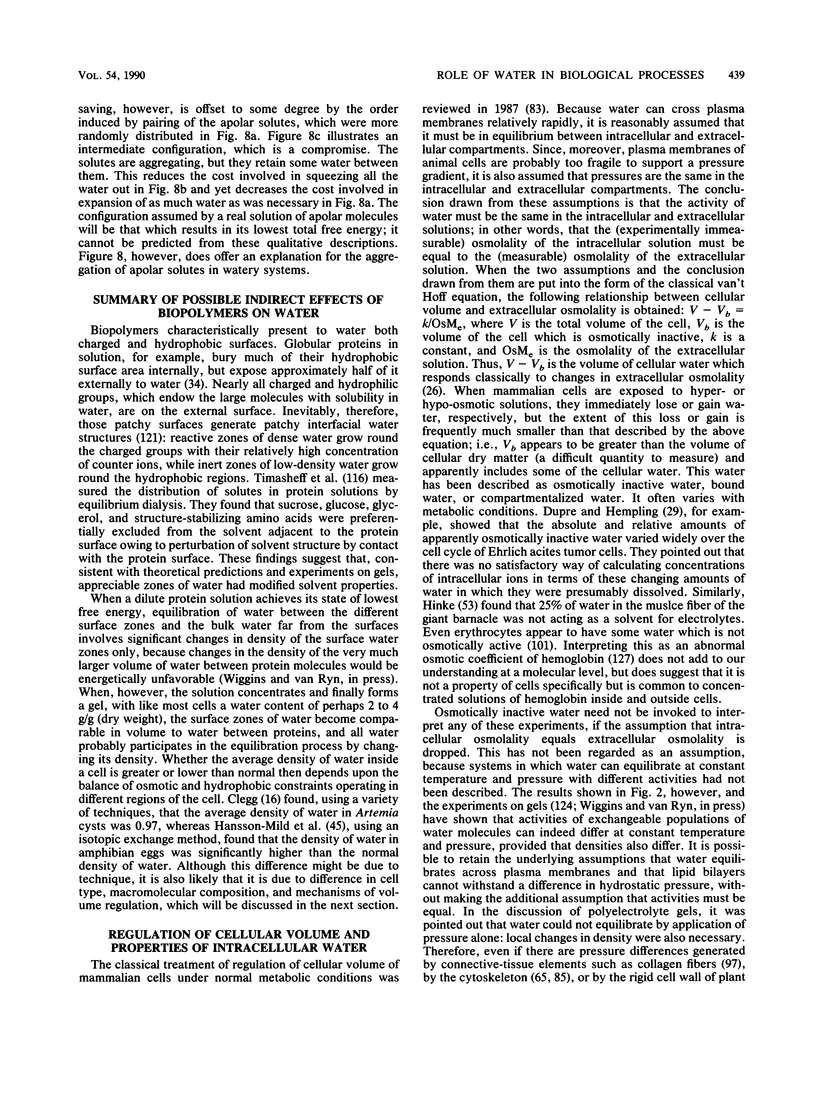
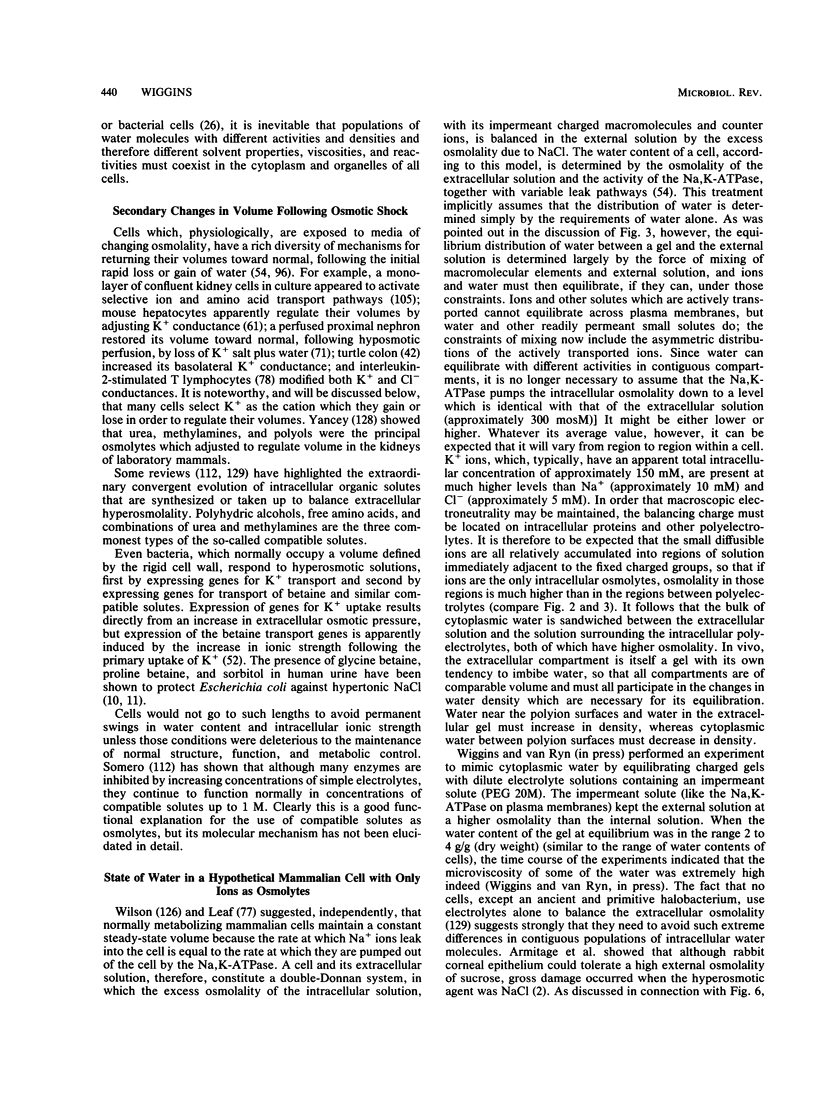
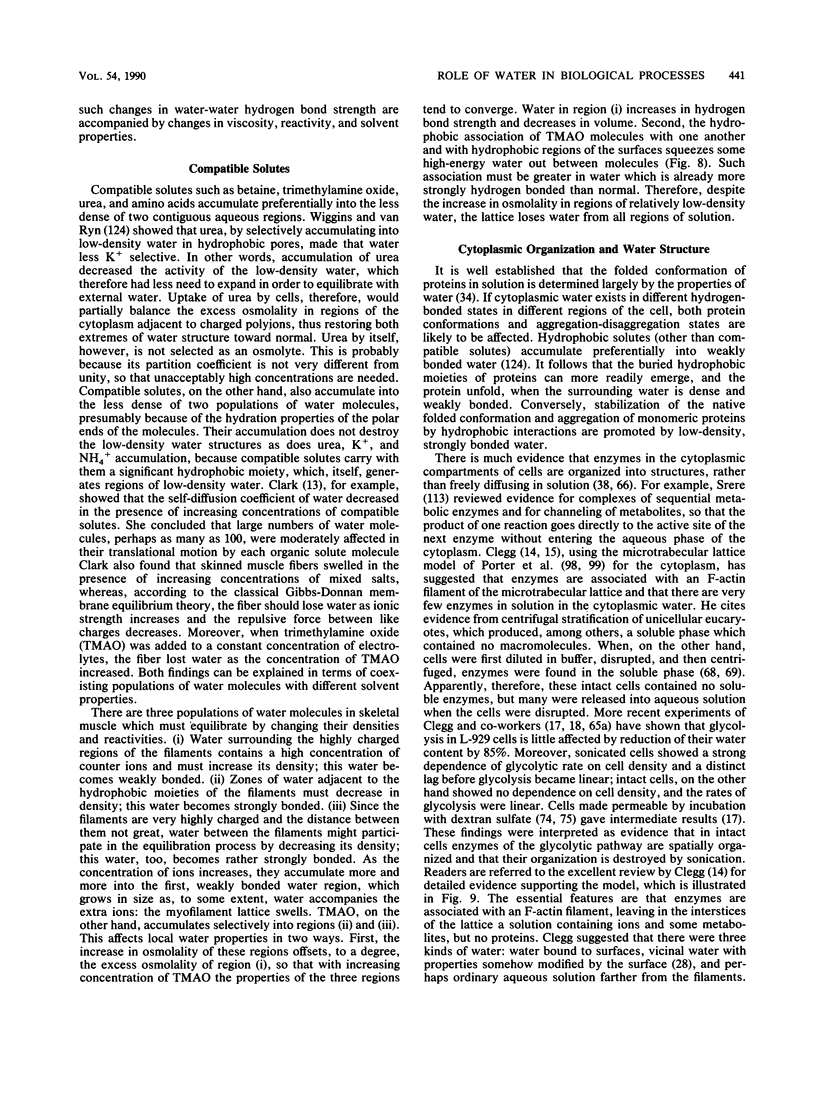
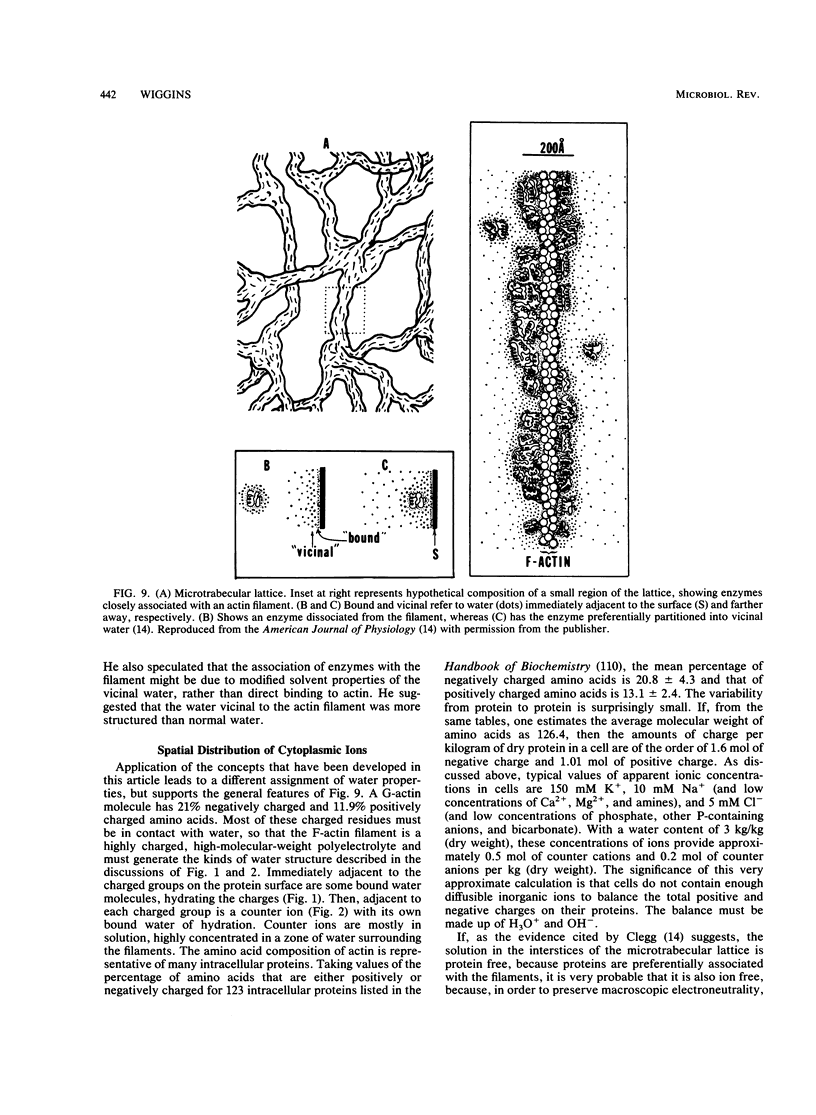
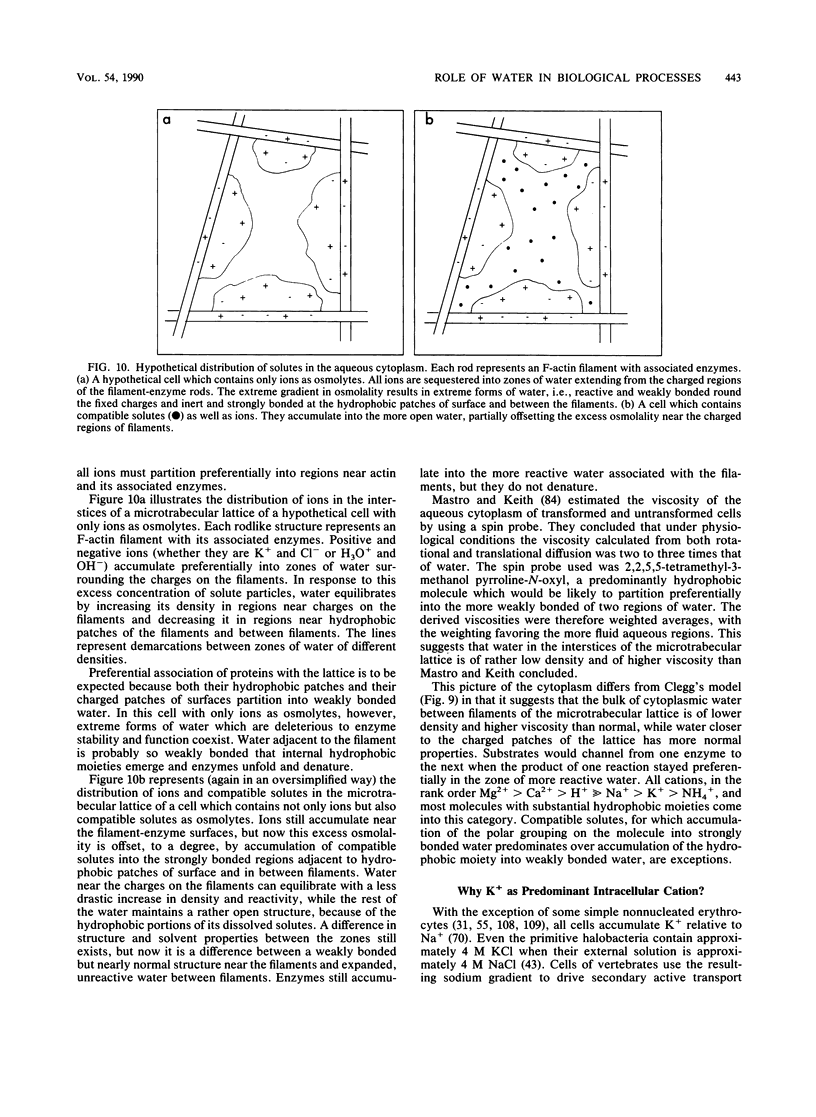
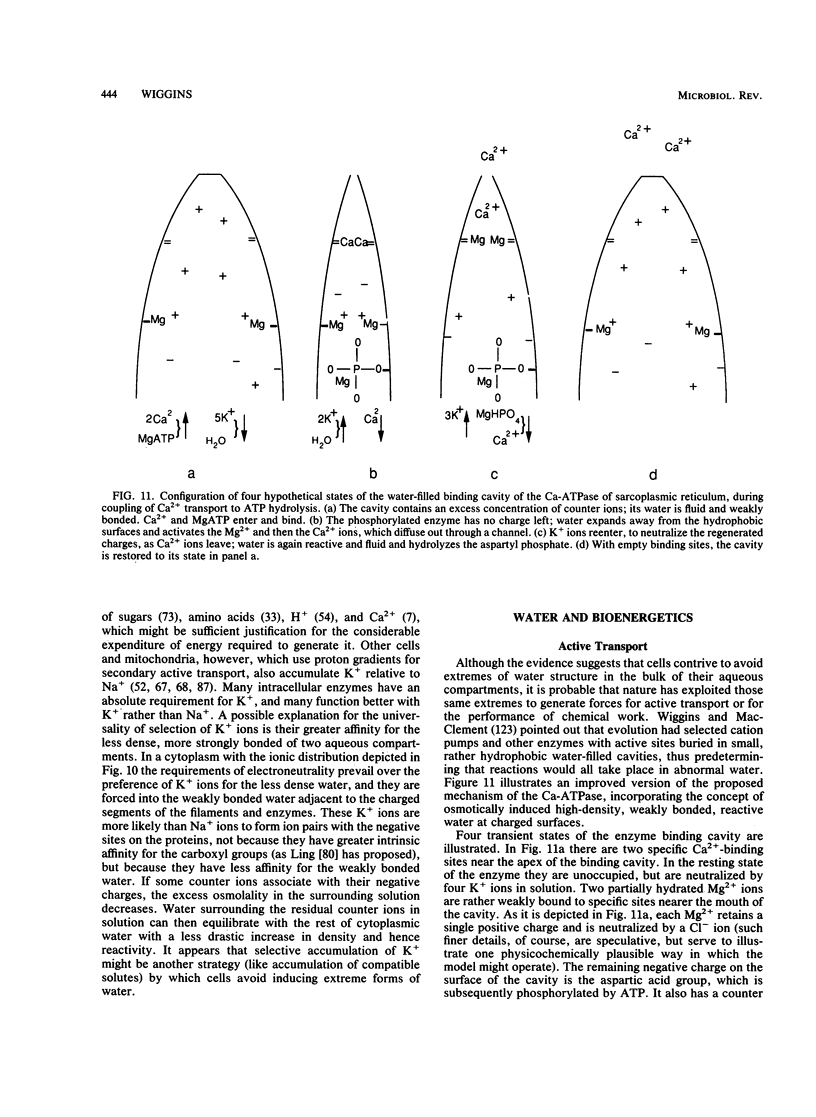
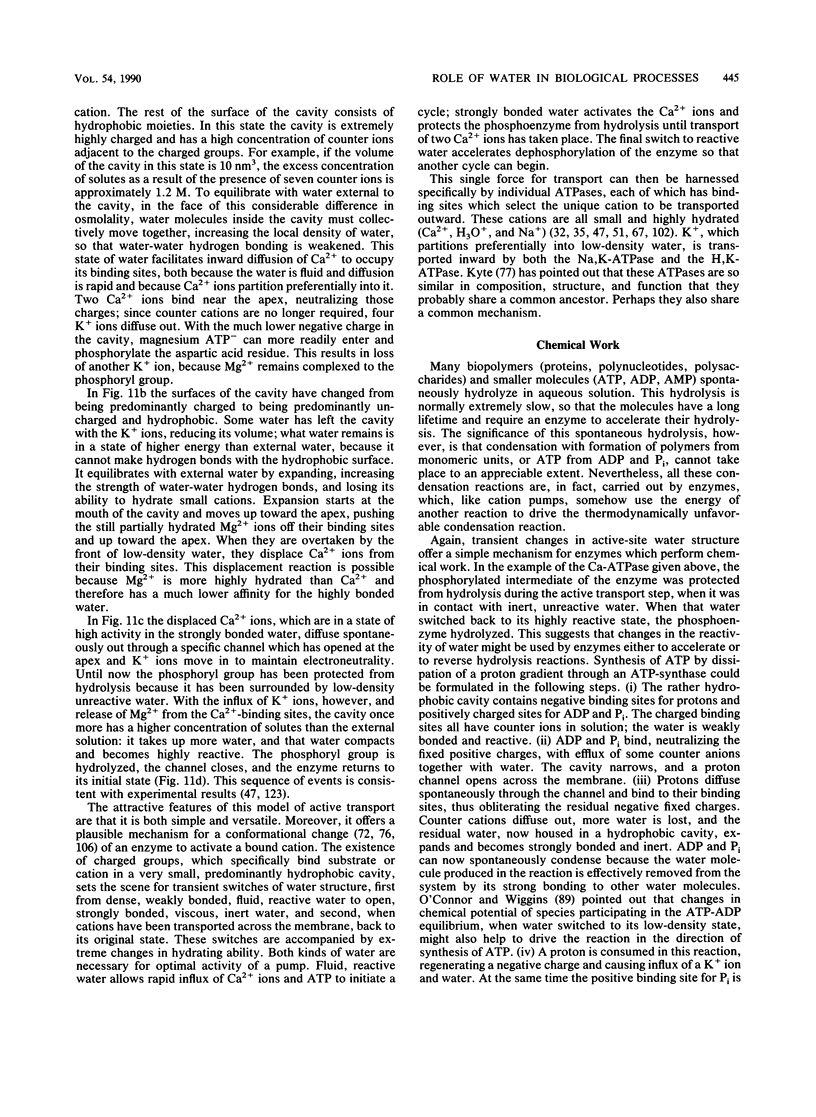
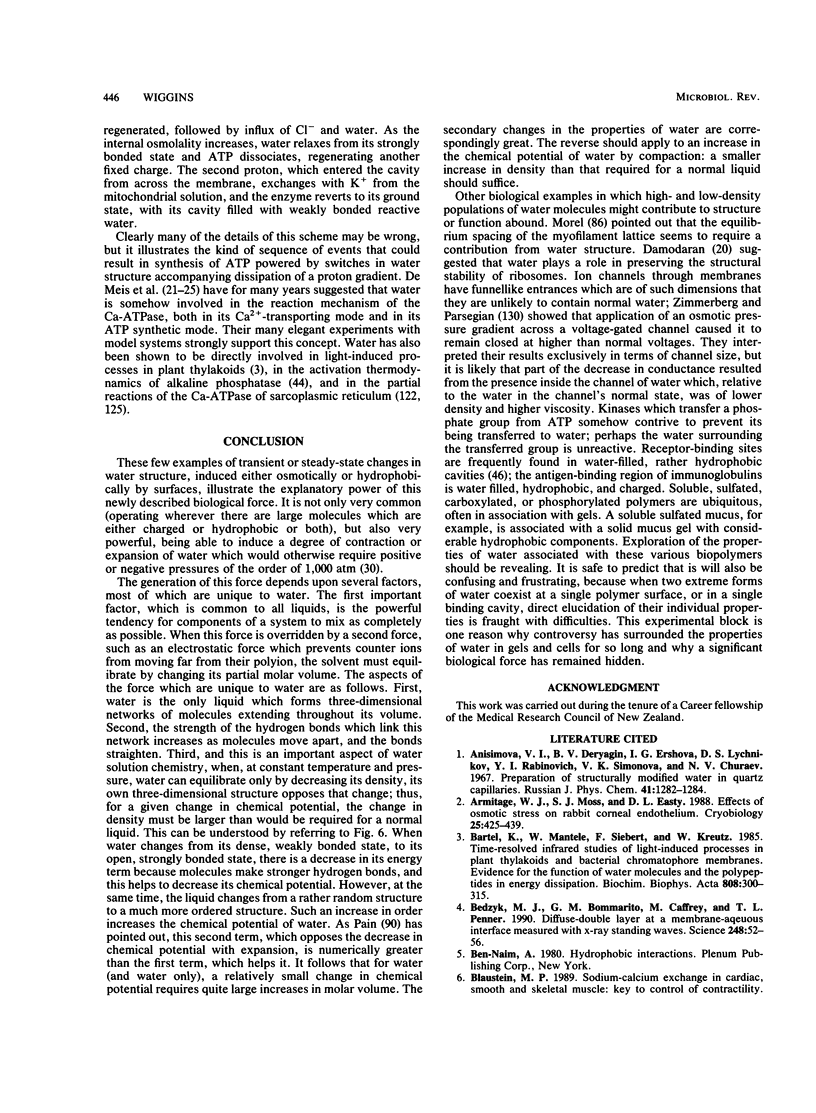
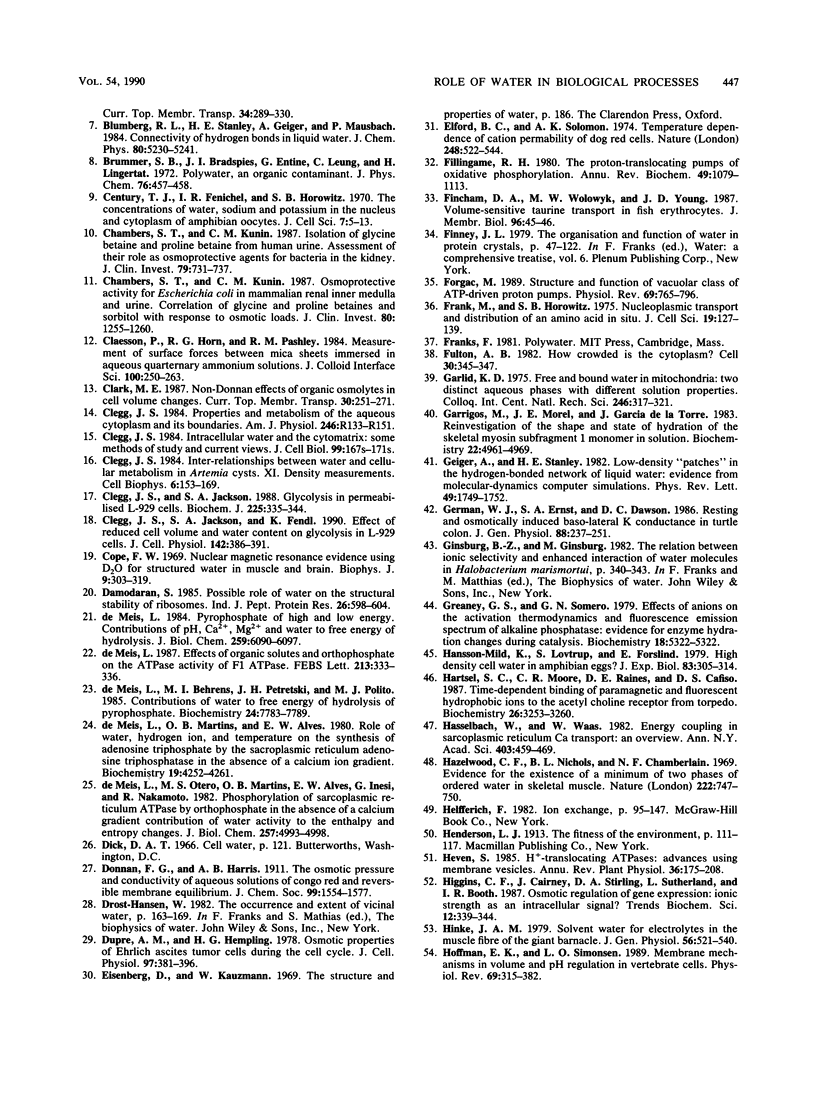
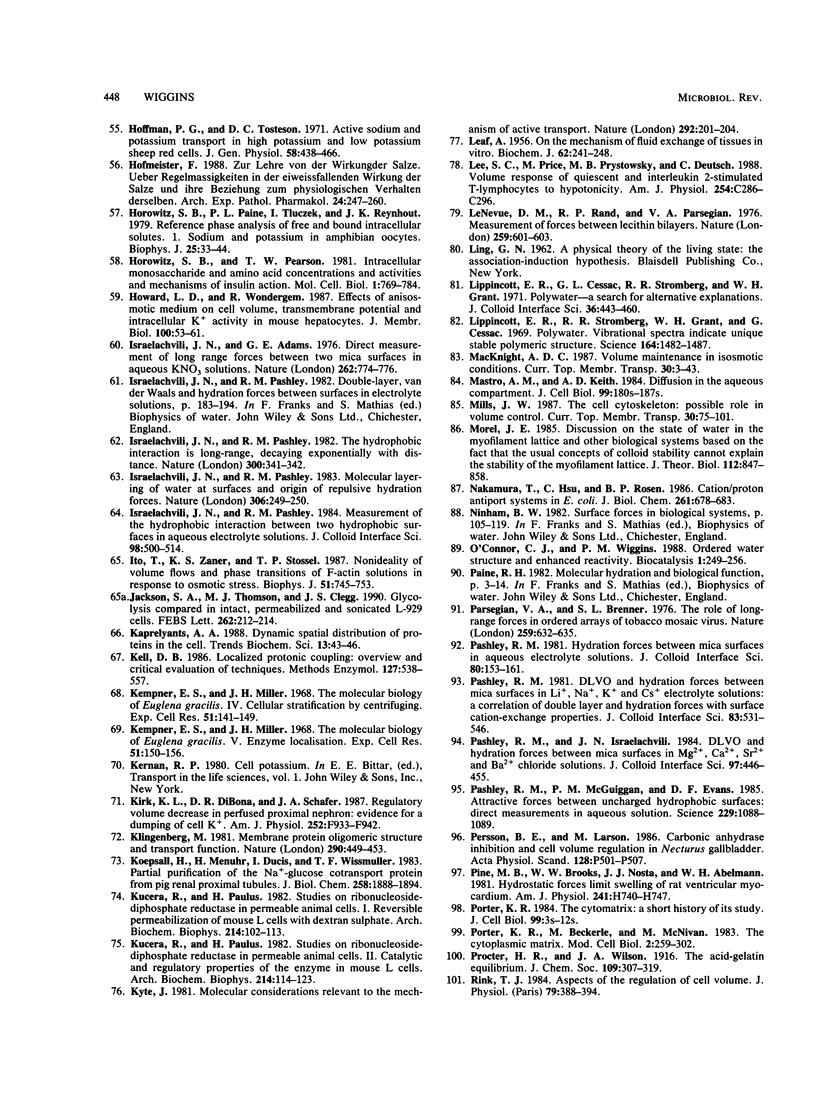
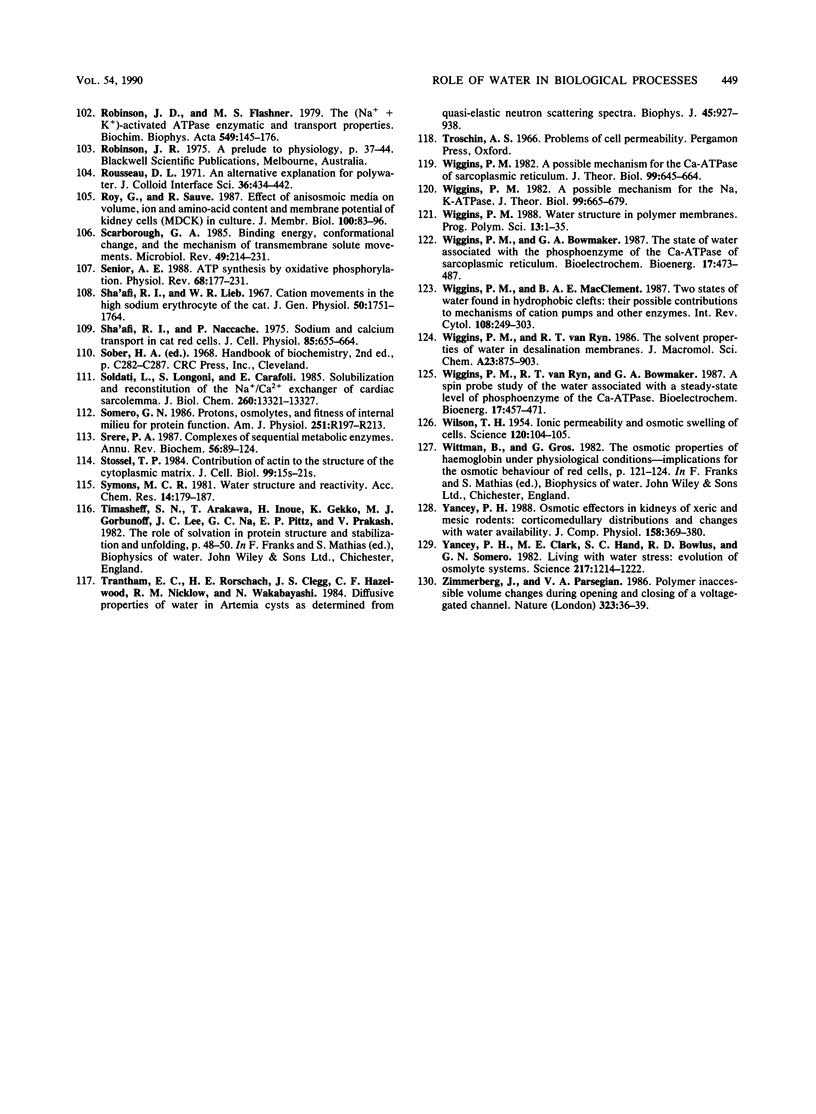
Images in this article
Selected References
These references are in PubMed. This may not be the complete list of references from this article.
- Armitage W. J., Moss S. J., Easty D. L. Effects of osmotic stress on rabbit corneal endothelium. Cryobiology. 1988 Oct;25(5):425–439. doi: 10.1016/0011-2240(88)90050-8. [DOI] [PubMed] [Google Scholar]
- Bedzyk M. J., Bommarito G. M., Caffrey M., Penner T. L. Diffuse-double layer at a membrane-aqueous interface measured with x-ray standing waves. Science. 1990 Apr 6;248(4951):52–56. doi: 10.1126/science.2321026. [DOI] [PubMed] [Google Scholar]
- Century T. J., Fenichel I. R., Horowitz S. B. The concentrations of water, sodium and potassium in the nucleus and cytoplasm of amphibian oocytes. J Cell Sci. 1970 Jul;7(1):5–13. doi: 10.1242/jcs.7.1.5. [DOI] [PubMed] [Google Scholar]
- Chambers S. T., Kunin C. M. Isolation of glycine betaine and proline betaine from human urine. Assessment of their role as osmoprotective agents for bacteria and the kidney. J Clin Invest. 1987 Mar;79(3):731–737. doi: 10.1172/JCI112878. [DOI] [PMC free article] [PubMed] [Google Scholar]
- Chambers S. T., Kunin C. M. Osmoprotective activity for Escherichia coli in mammalian renal inner medulla and urine. Correlation of glycine and proline betaines and sorbitol with response to osmotic loads. J Clin Invest. 1987 Nov;80(5):1255–1260. doi: 10.1172/JCI113200. [DOI] [PMC free article] [PubMed] [Google Scholar]
- Clegg J. S. Interrelationships between water and cellular metabolism in Artemia cysts. XI. Density measurements. Cell Biophys. 1984 Sep;6(3):153–169. doi: 10.1007/BF02788616. [DOI] [PubMed] [Google Scholar]
- Clegg J. S. Intracellular water and the cytomatrix: some methods of study and current views. J Cell Biol. 1984 Jul;99(1 Pt 2):167s–171s. doi: 10.1083/jcb.99.1.167s. [DOI] [PMC free article] [PubMed] [Google Scholar]
- Clegg J. S., Jackson S. A., Fendl K. Effects of reduced cell volume and water content on glycolysis in L-929 cells. J Cell Physiol. 1990 Feb;142(2):386–391. doi: 10.1002/jcp.1041420223. [DOI] [PubMed] [Google Scholar]
- Clegg J. S., Jackson S. A. Glycolysis in permeabilized L-929 cells. Biochem J. 1988 Oct 1;255(1):335–344. [PMC free article] [PubMed] [Google Scholar]
- Clegg J. S. Properties and metabolism of the aqueous cytoplasm and its boundaries. Am J Physiol. 1984 Feb;246(2 Pt 2):R133–R151. doi: 10.1152/ajpregu.1984.246.2.R133. [DOI] [PubMed] [Google Scholar]
- Cope F. W. Nuclear magnetic resonance evidence using D2O for structured water in muscle and brain. Biophys J. 1969 Mar;9(3):303–319. doi: 10.1016/S0006-3495(69)86388-5. [DOI] [PMC free article] [PubMed] [Google Scholar]
- DuPre A. M., Hempling H. G. Osmotic properties of Ehrlich ascites tumor cells during the cell cycle. J Cell Physiol. 1978 Dec;97(3 Pt 2 Suppl 1):381–396. doi: 10.1002/jcp.1040970313. [DOI] [PubMed] [Google Scholar]
- Elford B. C., Solomon A. K. Temperature dependence of cation permeability of dog red cells. Nature. 1974 Apr 5;248(448):522–524. doi: 10.1038/248522a0. [DOI] [PubMed] [Google Scholar]
- Fillingame R. H. The proton-translocating pumps of oxidative phosphorylation. Annu Rev Biochem. 1980;49:1079–1113. doi: 10.1146/annurev.bi.49.070180.005243. [DOI] [PubMed] [Google Scholar]
- Fincham D. A., Wolowyk M. W., Young J. D. Volume-sensitive taurine transport in fish erythrocytes. J Membr Biol. 1987;96(1):45–56. doi: 10.1007/BF01869333. [DOI] [PubMed] [Google Scholar]
- Forgac M. Structure and function of vacuolar class of ATP-driven proton pumps. Physiol Rev. 1989 Jul;69(3):765–796. doi: 10.1152/physrev.1989.69.3.765. [DOI] [PubMed] [Google Scholar]
- Frank M., Horowitz S. B. Nucleocytoplasmic transport and distribution of an amino acid, in situ. J Cell Sci. 1975 Oct;19(1):127–139. doi: 10.1242/jcs.19.1.127. [DOI] [PubMed] [Google Scholar]
- Fulton A. B. How crowded is the cytoplasm? Cell. 1982 Sep;30(2):345–347. doi: 10.1016/0092-8674(82)90231-8. [DOI] [PubMed] [Google Scholar]
- Garrigos M., Morel J. E., Garcia de la Torre J. Reinvestigation of the shape and state of hydration of the skeletal myosin subfragment 1 monomer in solution. Biochemistry. 1983 Oct 11;22(21):4961–4969. doi: 10.1021/bi00290a013. [DOI] [PubMed] [Google Scholar]
- Germann W. J., Lowy M. E., Ernst S. A., Dawson D. C. Differentiation of two distinct K conductances in the basolateral membrane of turtle colon. J Gen Physiol. 1986 Aug;88(2):237–251. doi: 10.1085/jgp.88.2.237. [DOI] [PMC free article] [PubMed] [Google Scholar]
- Greaney G. S., Somero G. N. Effects of anions on the activation thermodynamics and fluorescence emission spectrum of alkaline phosphatase: evidence for enzyme hydration changes during catalysis. Biochemistry. 1979 Nov 27;18(24):5322–5332. doi: 10.1021/bi00591a010. [DOI] [PubMed] [Google Scholar]
- Hartsel S. C., Moore C. R., Raines D. E., Cafiso D. S. Time-dependent binding of paramagnetic and fluorescent hydrophobic ions to the acetylcholine receptor from Torpedo. Biochemistry. 1987 Jun 16;26(12):3253–3260. doi: 10.1021/bi00386a003. [DOI] [PubMed] [Google Scholar]
- Hasselbach W., Waas W. Energy coupling in sarcoplasmic reticulum Ca2+ transport: an overview. Ann N Y Acad Sci. 1982;402:459–469. doi: 10.1111/j.1749-6632.1982.tb25768.x. [DOI] [PubMed] [Google Scholar]
- Hazlewood C. F., Nichols B. L., Chamberlain N. F. Evidence for the existence of a minimum of two phases of ordered water in skeletal muscle. Nature. 1969 May 24;222(5195):747–750. doi: 10.1038/222747a0. [DOI] [PubMed] [Google Scholar]
- Hinke J. A. Solvent water for electrolytes in the muscle fiber of the giant barnacle. J Gen Physiol. 1970 Oct;56(4):521–541. doi: 10.1085/jgp.56.4.521. [DOI] [PMC free article] [PubMed] [Google Scholar]
- Hoffman P. G., Tosteson D. C. Active sodium and potassium transport in high potassium and low potassium sheep red cells. J Gen Physiol. 1971 Oct;58(4):438–466. doi: 10.1085/jgp.58.4.438. [DOI] [PMC free article] [PubMed] [Google Scholar]
- Hoffmann E. K., Simonsen L. O. Membrane mechanisms in volume and pH regulation in vertebrate cells. Physiol Rev. 1989 Apr;69(2):315–382. doi: 10.1152/physrev.1989.69.2.315. [DOI] [PubMed] [Google Scholar]
- Horowitz S. B., Paine P. L., Tluczek L., Reynhout J. K. Reference phase analysis of free and bound intracellular solutes. I. Sodium and potassium in amphibian oocytes. Biophys J. 1979 Jan;25(1):33–44. doi: 10.1016/S0006-3495(79)85276-5. [DOI] [PMC free article] [PubMed] [Google Scholar]
- Horowitz S. B., Pearson T. W. Intracellular monosaccharide and amino acid concentrations and activities and the mechanisms of insulin action. Mol Cell Biol. 1981 Sep;1(9):769–784. doi: 10.1128/mcb.1.9.769. [DOI] [PMC free article] [PubMed] [Google Scholar]
- Howard L. D., Wondergem R. Effects of anisosmotic medium on cell volume, transmembrane potential and intracellular K+ activity in mouse hepatocytes. J Membr Biol. 1987;100(1):53–61. doi: 10.1007/BF02209140. [DOI] [PubMed] [Google Scholar]
- Israelachvili J., Pashley R. The hydrophobic interaction is long range, decaying exponentially with distance. Nature. 1982 Nov 25;300(5890):341–342. doi: 10.1038/300341a0. [DOI] [PubMed] [Google Scholar]
- Ito T., Zaner K. S., Stossel T. P. Nonideality of volume flows and phase transitions of F-actin solutions in response to osmotic stress. Biophys J. 1987 May;51(5):745–753. doi: 10.1016/S0006-3495(87)83401-X. [DOI] [PMC free article] [PubMed] [Google Scholar]
- Jackson S. A., Thomson M. J., Clegg J. S. Glycolysis compared in intact, permeabilized and sonicated L-929 cells. FEBS Lett. 1990 Mar 26;262(2):212–214. doi: 10.1016/0014-5793(90)80192-l. [DOI] [PubMed] [Google Scholar]
- Kaprelyants A. S. Dynamic spatial distribution of proteins in the cell. Trends Biochem Sci. 1988 Feb;13(2):43–46. doi: 10.1016/0968-0004(88)90024-2. [DOI] [PubMed] [Google Scholar]
- Kell D. B. Localized protonic coupling: overview and critical evaluation of techniques. Methods Enzymol. 1986;127:538–557. doi: 10.1016/0076-6879(86)27043-3. [DOI] [PubMed] [Google Scholar]
- Kempner E. S., Miller J. H. The molecular biology of Euglena gracilis. IV. Cellular stratification by centrifuging. Exp Cell Res. 1968 Jul;51(1):141–149. doi: 10.1016/0014-4827(68)90164-x. [DOI] [PubMed] [Google Scholar]
- Kempner E. S., Miller J. H. The molecular biology of Euglena gracilis. V. Enzyme localization. Exp Cell Res. 1968 Jul;51(1):150–156. doi: 10.1016/0014-4827(68)90165-1. [DOI] [PubMed] [Google Scholar]
- Kirk K. L., DiBona D. R., Schafer J. A. Regulatory volume decrease in perfused proximal nephron: evidence for a dumping of cell K+. Am J Physiol. 1987 May;252(5 Pt 2):F933–F942. doi: 10.1152/ajprenal.1987.252.5.F933. [DOI] [PubMed] [Google Scholar]
- Klingenberg M. Membrane protein oligomeric structure and transport function. Nature. 1981 Apr 9;290(5806):449–454. doi: 10.1038/290449a0. [DOI] [PubMed] [Google Scholar]
- Koepsell H., Menuhr H., Ducis I., Wissmüller T. F. Partial purification and reconstitution of the Na+-D-glucose cotransport protein from pig renal proximal tubules. J Biol Chem. 1983 Feb 10;258(3):1888–1894. [PubMed] [Google Scholar]
- Kucera R., Paulus H. Studied on ribonucleoside-diphosphate reductase in permeable animal cells. I. Reversible permeabilization of mouse L cells with dextran sulfate. Arch Biochem Biophys. 1982 Mar;214(1):102–113. doi: 10.1016/0003-9861(82)90012-1. [DOI] [PubMed] [Google Scholar]
- Kucera R., Paulus H. Studies on ribonucleoside-diphosphate reductase in permeable animal cells. II. Catalytic and regulatory properties of the enzyme in mouse L cells. Arch Biochem Biophys. 1982 Mar;214(1):114–123. doi: 10.1016/0003-9861(82)90013-3. [DOI] [PubMed] [Google Scholar]
- Kyte J. Molecular considerations relevant to the mechanism of active transport. Nature. 1981 Jul 16;292(5820):201–204. doi: 10.1038/292201a0. [DOI] [PubMed] [Google Scholar]
- LEAF A. On the mechanism of fluid exchange of tissues in vitro. Biochem J. 1956 Feb;62(2):241–248. doi: 10.1042/bj0620241. [DOI] [PMC free article] [PubMed] [Google Scholar]
- LeNeveu D. M., Rand R. P., Parsegian V. A. Measurement of forces between lecithin bilayers. Nature. 1976 Feb 19;259(5544):601–603. doi: 10.1038/259601a0. [DOI] [PubMed] [Google Scholar]
- Lee S. C., Price M., Prystowsky M. B., Deutsch C. Volume response of quiescent and interleukin 2-stimulated T-lymphocytes to hypotonicity. Am J Physiol. 1988 Feb;254(2 Pt 1):C286–C296. doi: 10.1152/ajpcell.1988.254.2.C286. [DOI] [PubMed] [Google Scholar]
- Lippincott E. R., Stromberg R. R., Grant W. H., Cessac G. L. Polywater. Science. 1969 Jun 27;164(3887):1482–1487. doi: 10.1126/science.164.3887.1482. [DOI] [PubMed] [Google Scholar]
- Mastro A. M., Keith A. D. Diffusion in the aqueous compartment. J Cell Biol. 1984 Jul;99(1 Pt 2):180s–187s. doi: 10.1083/jcb.99.1.180s. [DOI] [PMC free article] [PubMed] [Google Scholar]
- Mild K. H., Løvtrup S., Forslind E. High density cell water in amphibian eggs? J Exp Biol. 1979 Dec;83:305–314. doi: 10.1242/jeb.83.1.305. [DOI] [PubMed] [Google Scholar]
- Morel J. E. Discussion on the state of water in the myofilament lattice and other biological systems, based on the fact that the usual concepts of colloid stability can not explain the stability of the myofilament lattice. J Theor Biol. 1985 Feb 21;112(4):847–858. doi: 10.1016/s0022-5193(85)80066-7. [DOI] [PubMed] [Google Scholar]
- Nakamura T., Hsu C., Rosen B. P. Cation/proton antiport systems in Escherichia coli. Solubilization and reconstitution of delta pH-driven sodium/proton and calcium/proton antiporters. J Biol Chem. 1986 Jan 15;261(2):678–683. [PubMed] [Google Scholar]
- Parsegian V. A., Brenner S. L. The role of long range forces in ordered arrays of tobacco mosaic virus. Nature. 1976 Feb 26;259(5545):632–635. doi: 10.1038/259632a0. [DOI] [PubMed] [Google Scholar]
- Pashley R. M., McGuiggan P. M., Ninham B. W., Evans D. F. Attractive forces between uncharged hydrophobic surfaces: direct measurements in aqueous solution. Science. 1985 Sep 13;229(4718):1088–1089. doi: 10.1126/science.4035349. [DOI] [PubMed] [Google Scholar]
- Pine M. B., Brooks W. W., Nosta J. J., Abelmann W. H. Hydrostatic forces limit swelling of rat ventricular myocardium. Am J Physiol. 1981 Nov;241(5):H740–H747. doi: 10.1152/ajpheart.1981.241.5.H740. [DOI] [PubMed] [Google Scholar]
- Porter K. R. The cytomatrix: a short history of its study. J Cell Biol. 1984 Jul;99(1 Pt 2):3s–12s. doi: 10.1083/jcb.99.1.3s. [DOI] [PMC free article] [PubMed] [Google Scholar]
- Rink T. J. Aspects of the regulation of cell volume. J Physiol (Paris) 1984;79(6):388–394. [PubMed] [Google Scholar]
- Robinson J. D., Flashner M. S. The (Na+ + K+)-activated ATPase. Enzymatic and transport properties. Biochim Biophys Acta. 1979 Aug 17;549(2):145–176. doi: 10.1016/0304-4173(79)90013-2. [DOI] [PubMed] [Google Scholar]
- Roy G., Sauvé R. Effect of anisotonic media on volume, ion and amino-acid content and membrane potential of kidney cells (MDCK) in culture. J Membr Biol. 1987;100(1):83–96. doi: 10.1007/BF02209143. [DOI] [PubMed] [Google Scholar]
- Scarborough G. A. Binding energy, conformational change, and the mechanism of transmembrane solute movements. Microbiol Rev. 1985 Sep;49(3):214–231. doi: 10.1128/mr.49.3.214-231.1985. [DOI] [PMC free article] [PubMed] [Google Scholar]
- Senior A. E. ATP synthesis by oxidative phosphorylation. Physiol Rev. 1988 Jan;68(1):177–231. doi: 10.1152/physrev.1988.68.1.177. [DOI] [PubMed] [Google Scholar]
- Sha'afi R. I., Lieb W. R. Cation movements in the high sodium erythrocyte of the cat. J Gen Physiol. 1967 Jul;50(6):1751–1764. doi: 10.1085/jgp.50.6.1751. [DOI] [PMC free article] [PubMed] [Google Scholar]
- Sha'afi R. I., Naccache P. Sodium and calcium transport in cat red cells. J Cell Physiol. 1975 Jun;85(3):655–664. doi: 10.1002/jcp.1040850318. [DOI] [PubMed] [Google Scholar]
- Soldati L., Longoni S., Carafoli E. Solubilization and reconstitution of the Na+/Ca2+ exchanger of cardiac sarcolemma. Properties of the reconstituted system and tentative identification of the protein(s) responsible for the exchange activity. J Biol Chem. 1985 Oct 25;260(24):13321–13327. [PubMed] [Google Scholar]
- Somero G. N. Protons, osmolytes, and fitness of internal milieu for protein function. Am J Physiol. 1986 Aug;251(2 Pt 2):R197–R213. doi: 10.1152/ajpregu.1986.251.2.R197. [DOI] [PubMed] [Google Scholar]
- Srere P. A. Complexes of sequential metabolic enzymes. Annu Rev Biochem. 1987;56:89–124. doi: 10.1146/annurev.bi.56.070187.000513. [DOI] [PubMed] [Google Scholar]
- Stossel T. P. Contribution of actin to the structure of the cytoplasmic matrix. J Cell Biol. 1984 Jul;99(1 Pt 2):15s–21s. doi: 10.1083/jcb.99.1.15s. [DOI] [PMC free article] [PubMed] [Google Scholar]
- Trantham E. C., Rorschach H. E., Clegg J. S., Hazlewood C. F., Nicklow R. M., Wakabayashi N. Diffusive properties of water in Artemia cysts as determined from quasi-elastic neutron scattering spectra. Biophys J. 1984 May;45(5):927–938. doi: 10.1016/S0006-3495(84)84239-3. [DOI] [PMC free article] [PubMed] [Google Scholar]
- WILSON T. H. Ionic permeability and osmotic swelling of cells. Science. 1954 Jul 16;120(3107):104–105. doi: 10.1126/science.120.3107.104. [DOI] [PubMed] [Google Scholar]
- Wiggins P. M. A possible mechanism for the Ca-ATPase of sarcoplasmic reticulum. J Theor Biol. 1982 Dec 21;99(4):645–664. doi: 10.1016/0022-5193(82)90192-8. [DOI] [PubMed] [Google Scholar]
- Wiggins P. M. A possible mechanism for the Na,K-ATPase. J Theor Biol. 1982 Dec 21;99(4):665–679. doi: 10.1016/0022-5193(82)90193-x. [DOI] [PubMed] [Google Scholar]
- Wiggins P. M., MacClement B. A. Two states of water found in hydrophobic clefts: their possible contribution to mechanisms of cation pumps and other enzymes. Int Rev Cytol. 1987;108:249–303. doi: 10.1016/s0074-7696(08)61440-0. [DOI] [PubMed] [Google Scholar]
- Yancey P. H., Clark M. E., Hand S. C., Bowlus R. D., Somero G. N. Living with water stress: evolution of osmolyte systems. Science. 1982 Sep 24;217(4566):1214–1222. doi: 10.1126/science.7112124. [DOI] [PubMed] [Google Scholar]
- Yancey P. H. Osmotic effectors in kidneys of xeric and mesic rodents: corticomedullary distributions and changes with water availability. J Comp Physiol B. 1988;158(3):369–380. doi: 10.1007/BF00695336. [DOI] [PubMed] [Google Scholar]
- Zimmerberg J., Parsegian V. A. Polymer inaccessible volume changes during opening and closing of a voltage-dependent ionic channel. Nature. 1986 Sep 4;323(6083):36–39. doi: 10.1038/323036a0. [DOI] [PubMed] [Google Scholar]
- de Meis L., Behrens M. I., Petretski J. H., Politi M. J. Contribution of water to free energy of hydrolysis of pyrophosphate. Biochemistry. 1985 Dec 17;24(26):7783–7789. doi: 10.1021/bi00347a042. [DOI] [PubMed] [Google Scholar]
- de Meis L. Effects of organic solvents and orthophosphate on the ATPase activity of F1 ATPase. FEBS Lett. 1987 Mar 23;213(2):333–336. doi: 10.1016/0014-5793(87)81517-x. [DOI] [PubMed] [Google Scholar]
- de Meis L., Martins O. B., Alves E. W. Role of water, hydrogen ion, and temperature on the synthesis of adenosine triphosphate by the sarcoplasmic reticulum adenosine triphosphatase in the absence of a calcium ion gradient. Biochemistry. 1980 Sep 2;19(18):4252–4261. doi: 10.1021/bi00559a017. [DOI] [PubMed] [Google Scholar]
- de Meis L. Pyrophosphate of high and low energy. Contributions of pH, Ca2+, Mg2+, and water to free energy of hydrolysis. J Biol Chem. 1984 May 25;259(10):6090–6097. [PubMed] [Google Scholar]
- de Meis L., de Souza Otero A., Martins O. B., Alves E. W., Inesi G., Nakamoto R. Phosphorylation of sarcoplasmic reticulum ATPase by orthophosphate in the absence of Ca2+ gradient. Contribution of water activity to the enthalpy and the entropy changes. J Biol Chem. 1982 May 10;257(9):4993–4998. [PubMed] [Google Scholar]