Abstract
All living cells must conduct protein synthesis with a high degree of accuracy maintained in the transmission and flow of information from gene to finished protein product. One crucial "quality control" point in maintaining a high level of accuracy is the selectivity by which aminoacyl-tRNA synthetases furnish correctly activated amino acids, attached to tRNA species, as the building blocks for growing protein chains. During selection of amino acids, synthetases very often have to distinguish the cognate substrate from a homolog having just one fewer methyl group in its structure. The binding energy of a methyl group is estimated to contribute only a factor of 100 to the specificity of binding, yet synthetases distinguish such closely related amino acids with a discrimination factor of 10,000 to 100,000. Examples of this include methionine versus homocysteine, isoleucine versus valine, alanine versus glycine, and threonine versus serine. Many investigators have demonstrated in vitro the ability of certain aminoacyl-tRNA synthetases to edit, that is, correct or prevent incorrect attachment of amino acids to tRNA molecules. Several major editing pathways are now established from in vitro data. Further, at least some aminoacyl-tRNA synthetases have recently been shown to carry out the editing function in vivo. Editing has been demonstrated to occur in both Escherichia coli and Saccharomyces cerevisiae. Significant energy is expended by the cell for editing of misactivated amino acids, which can be reflected in the growth rate. Because of this, cellular levels of aminoacyl-tRNA synthetases, as well as amino acid biosynthetic pathways which yield competing substrates for protein synthesis, must be carefully regulated to prevent excessive editing. High-level expression of recombinant proteins imposes a strain on the biosynthetic capacity of the cell which frequently results in misincorporation of abnormal or wrong amino acids owing in part to limited editing by synthetases. Unbalanced amino acid pools associated with some genetic disorders in humans may also lead to errors in tRNA aminoacylation. The availability of X-ray crystallographic structures of some synthetases, combined with site-directed mutagenesis, allows insights into molecular details of the extraordinary selectivity of synthetases, including the editing function.
Full text
PDF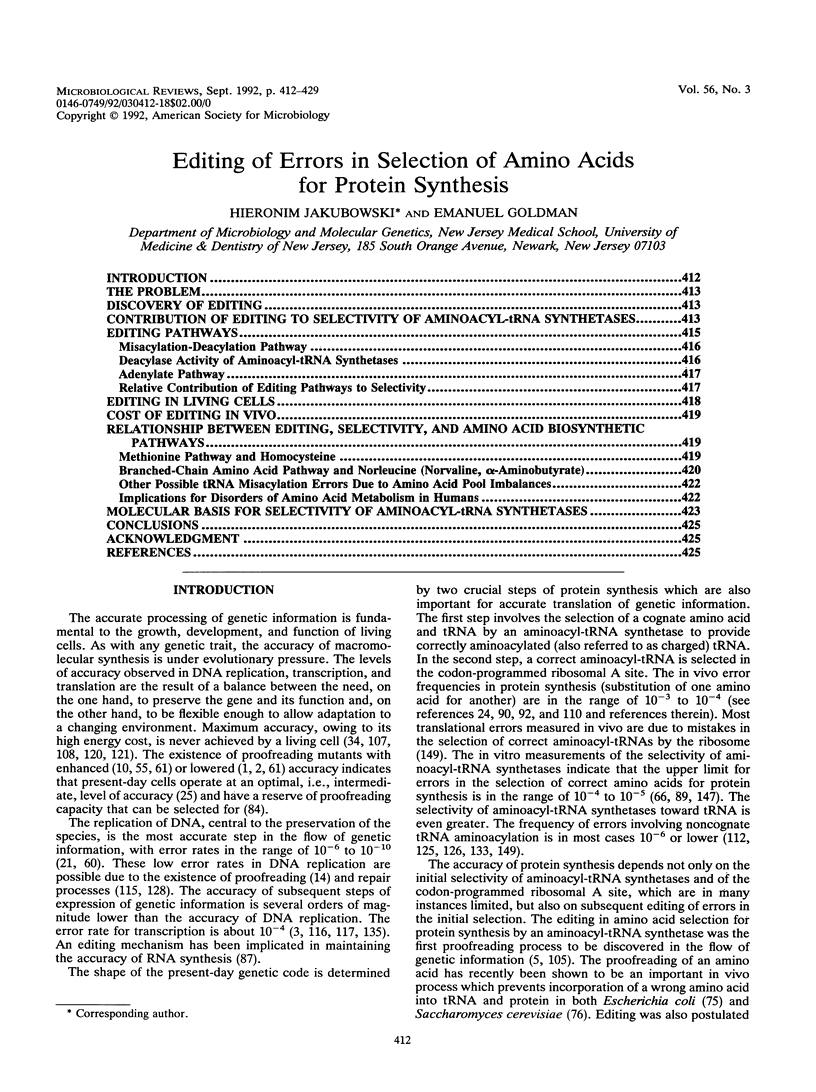
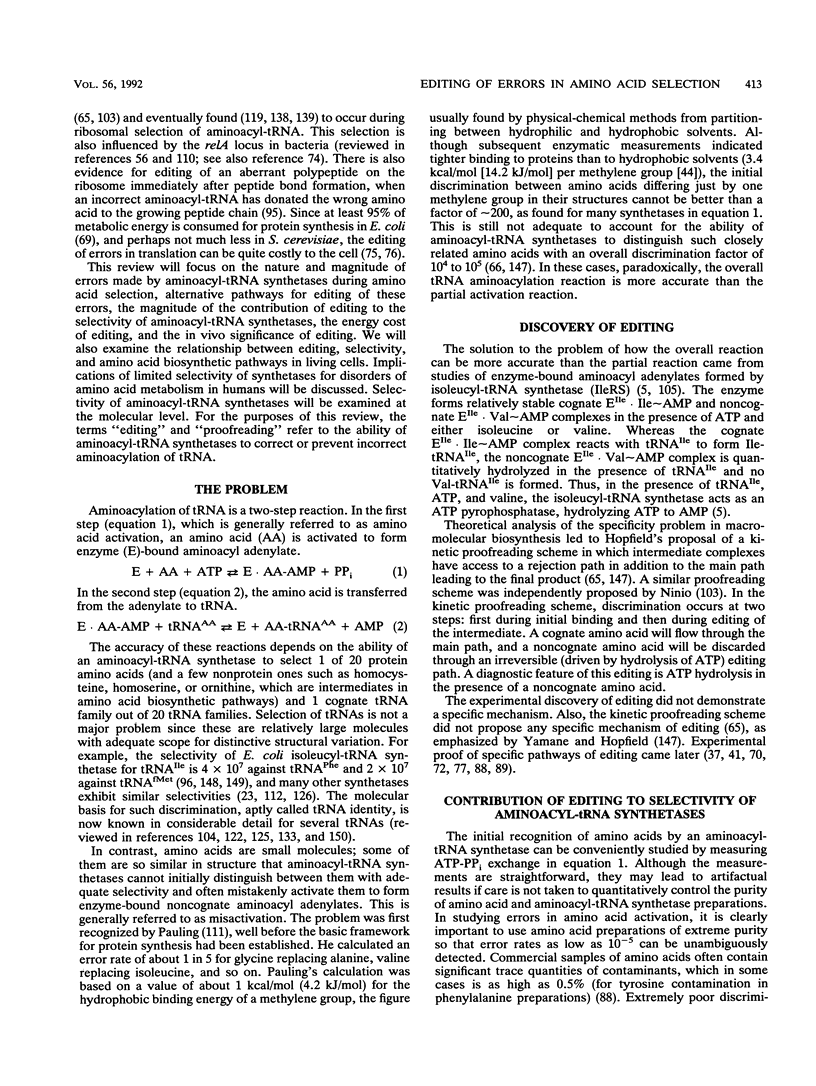
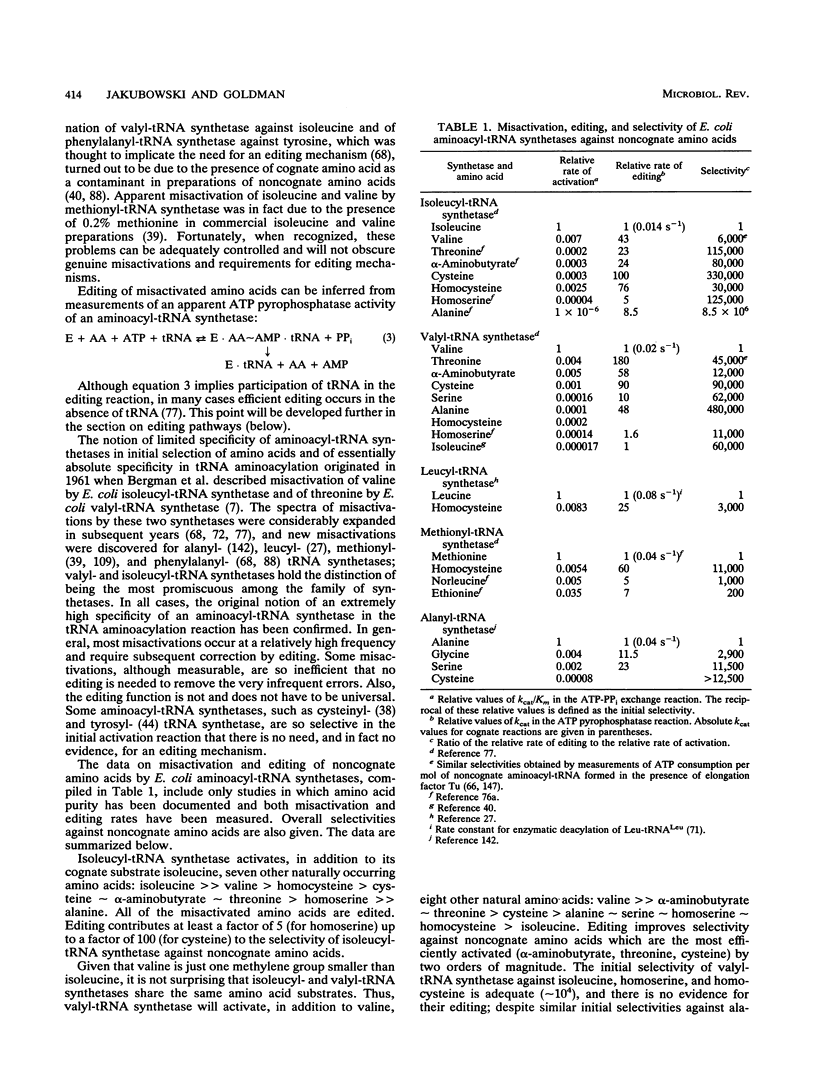
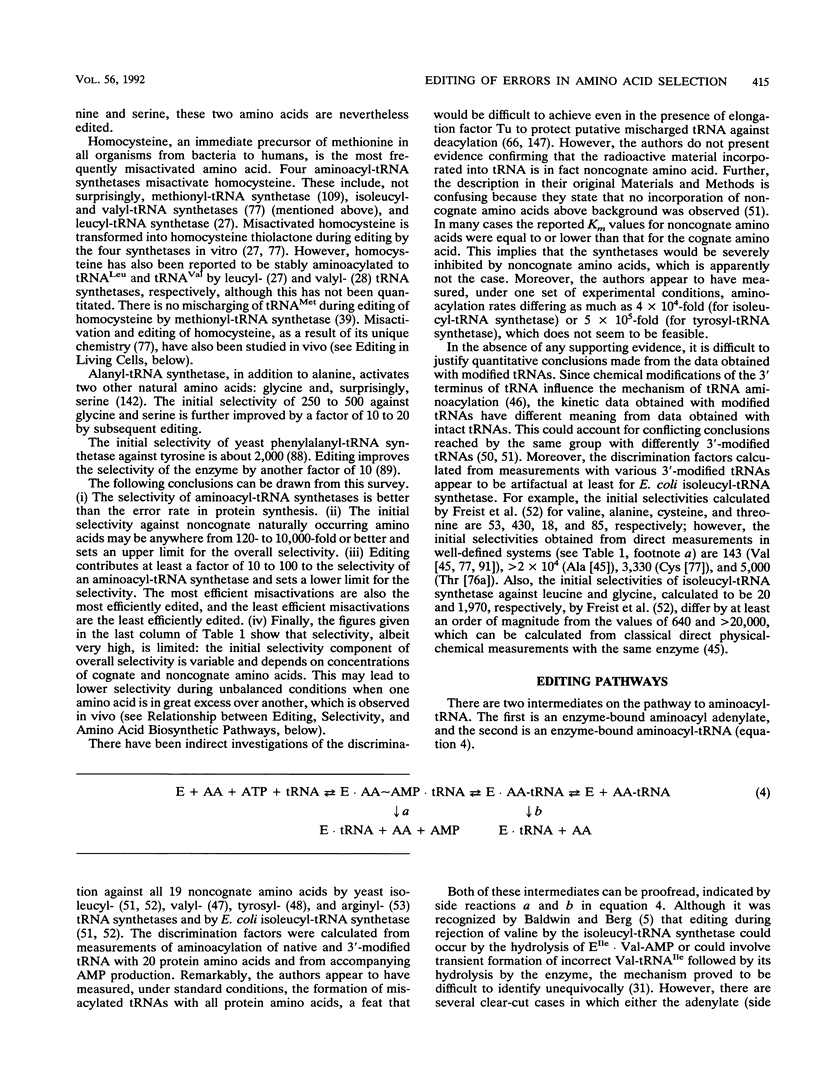
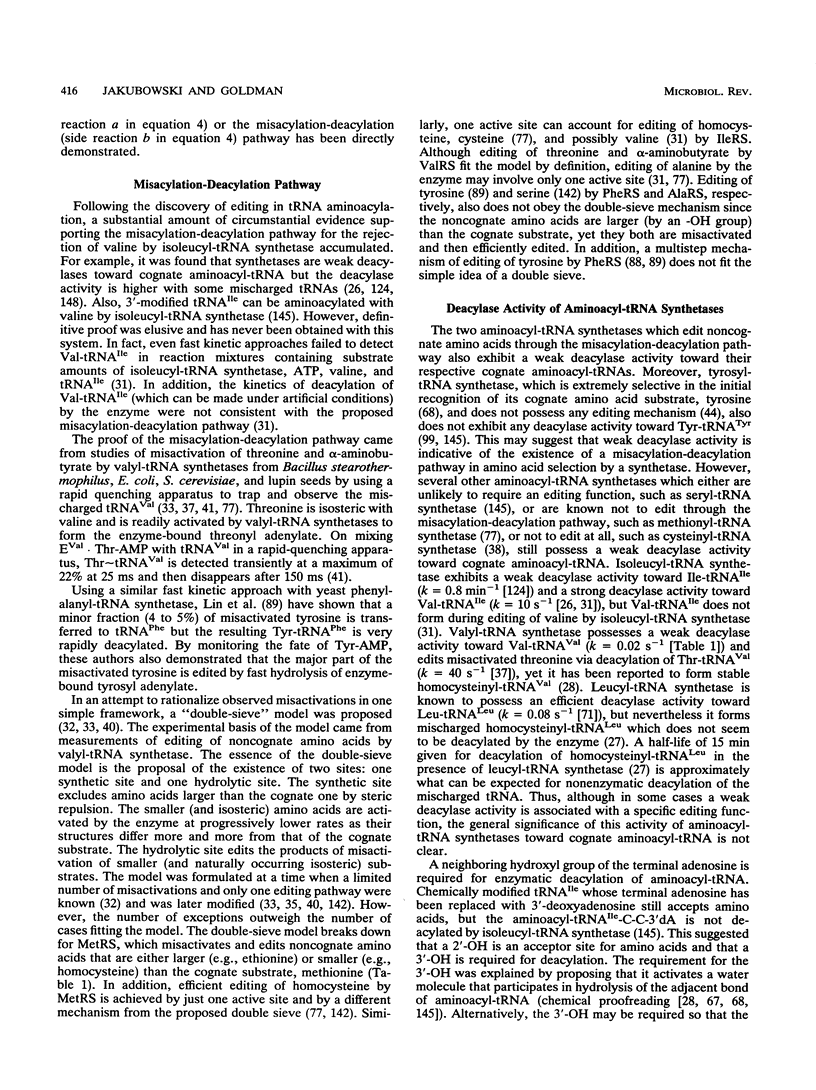
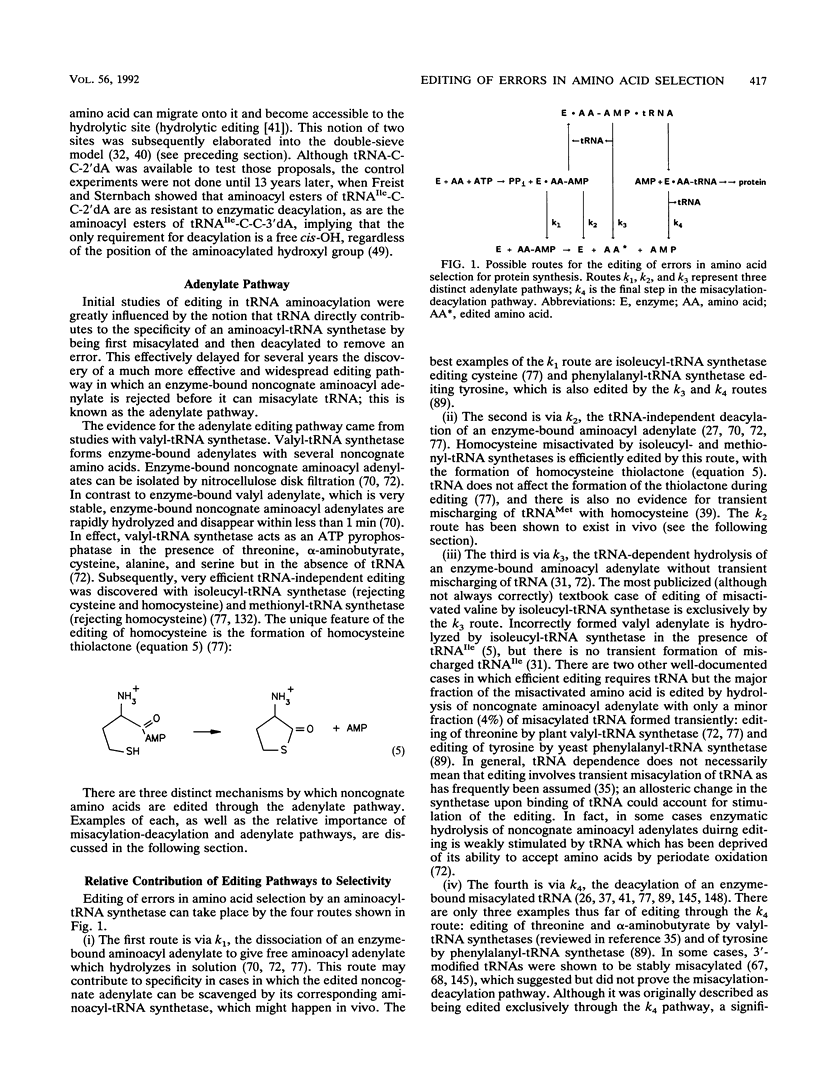
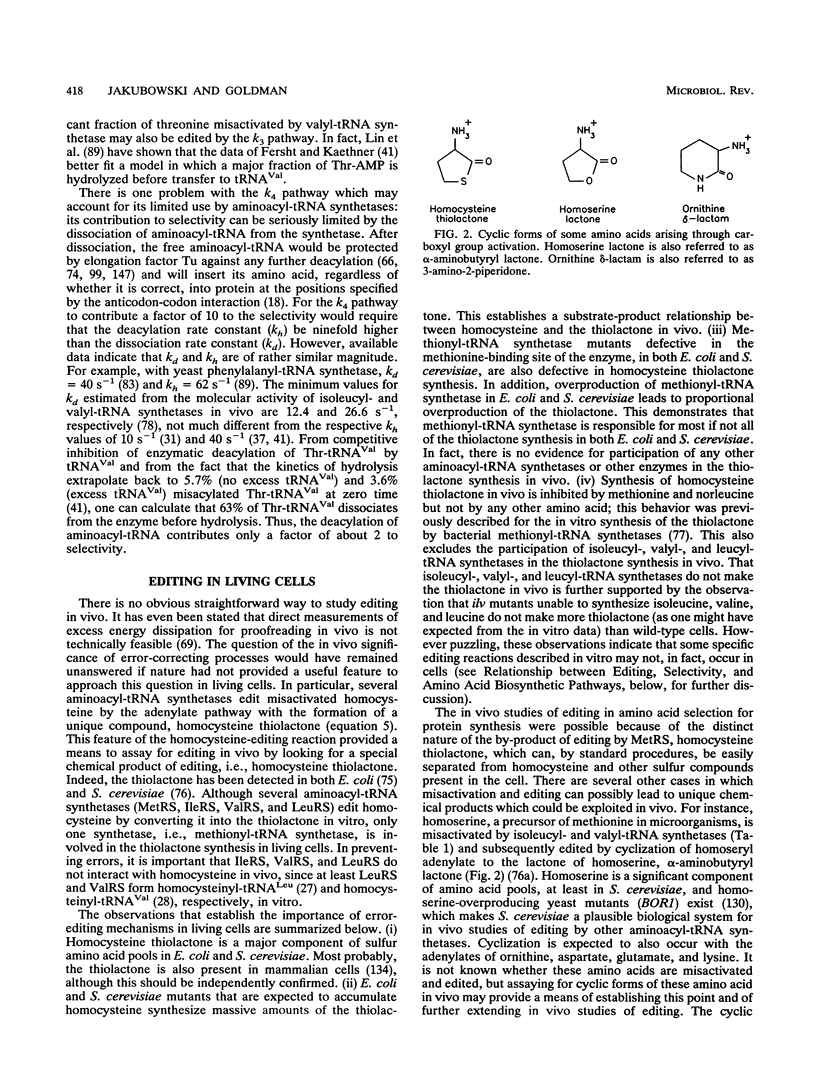
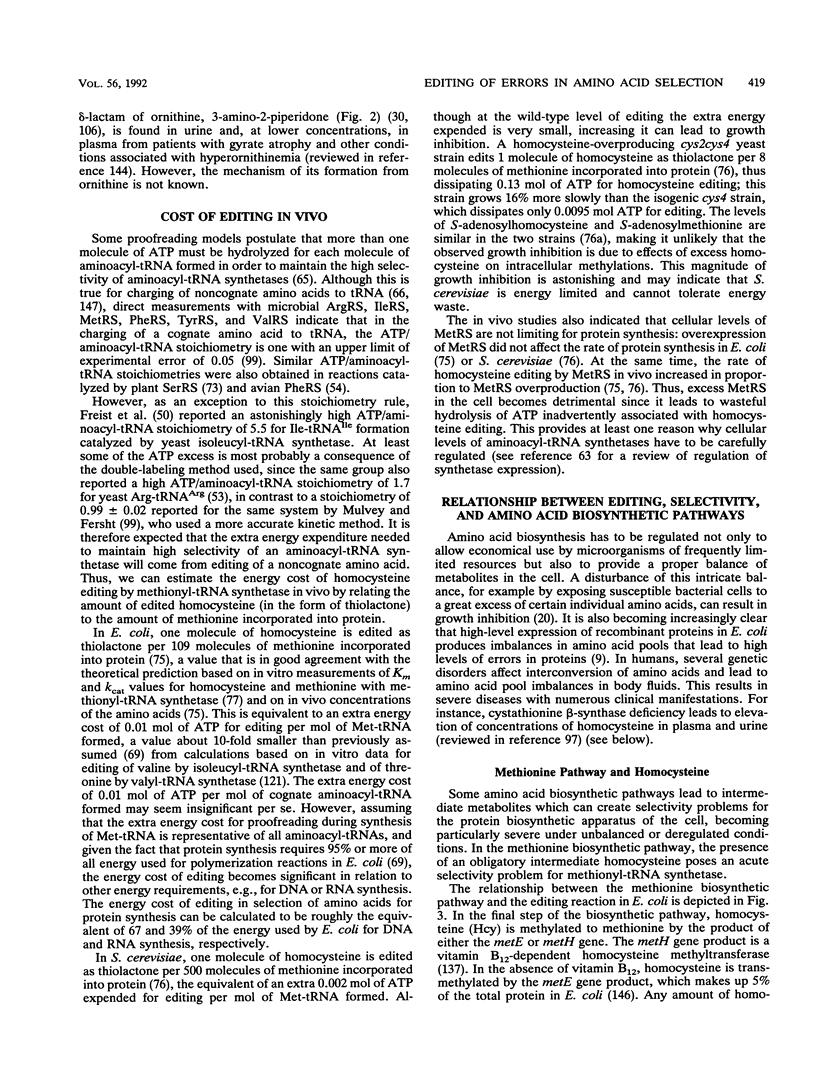
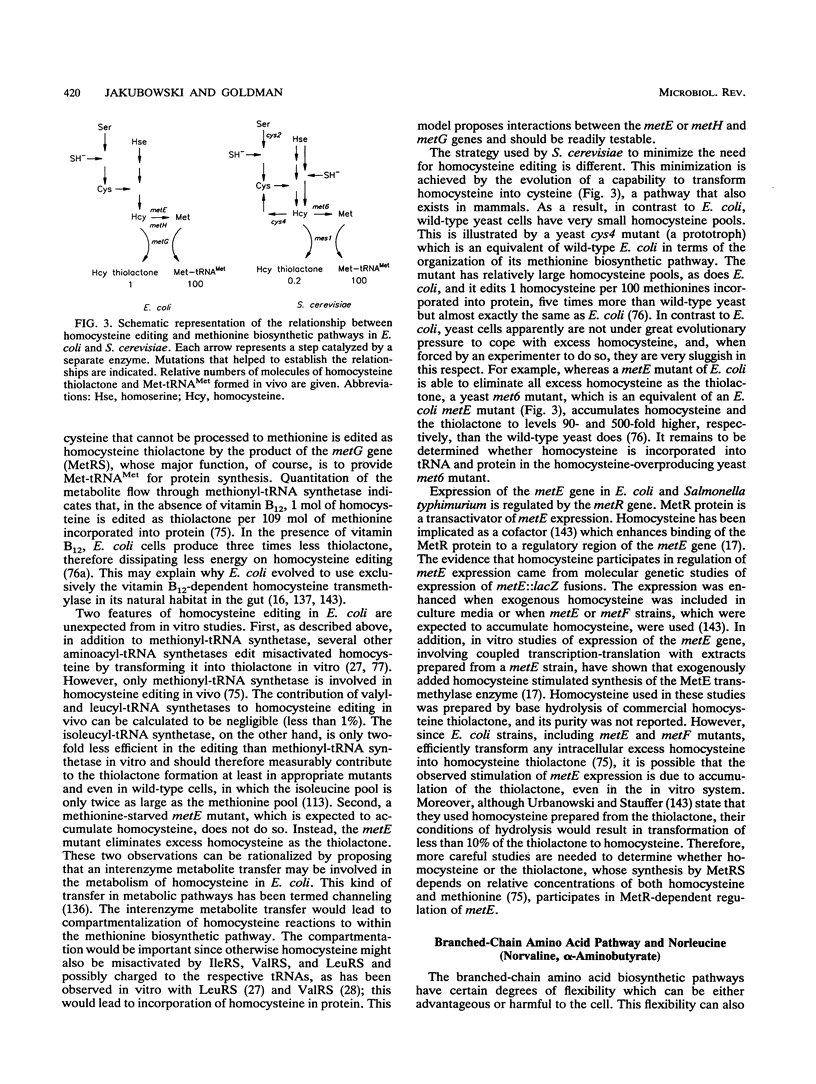
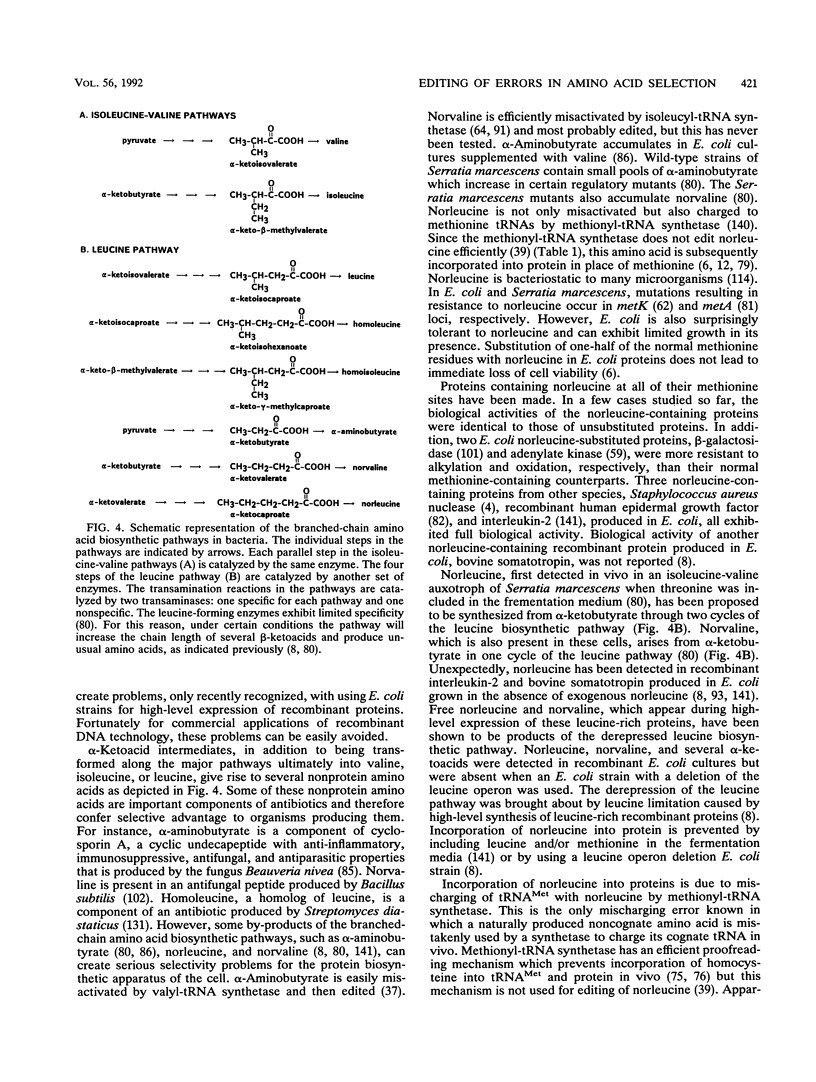
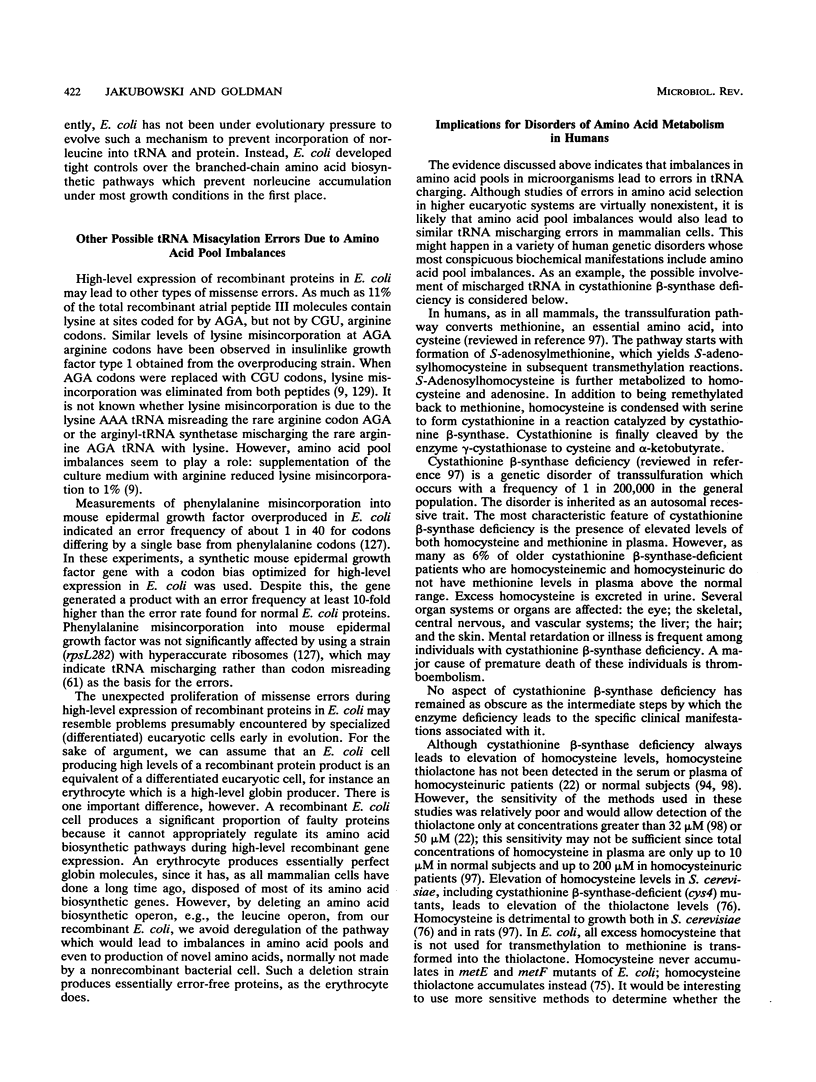
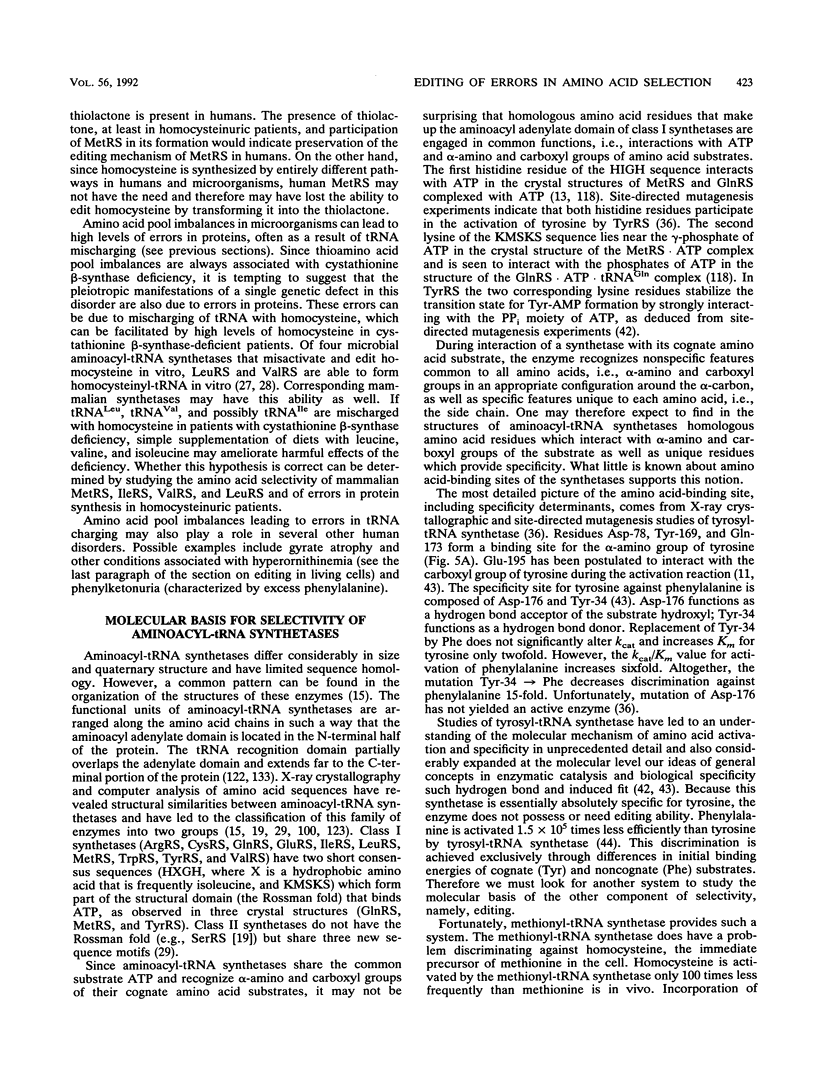
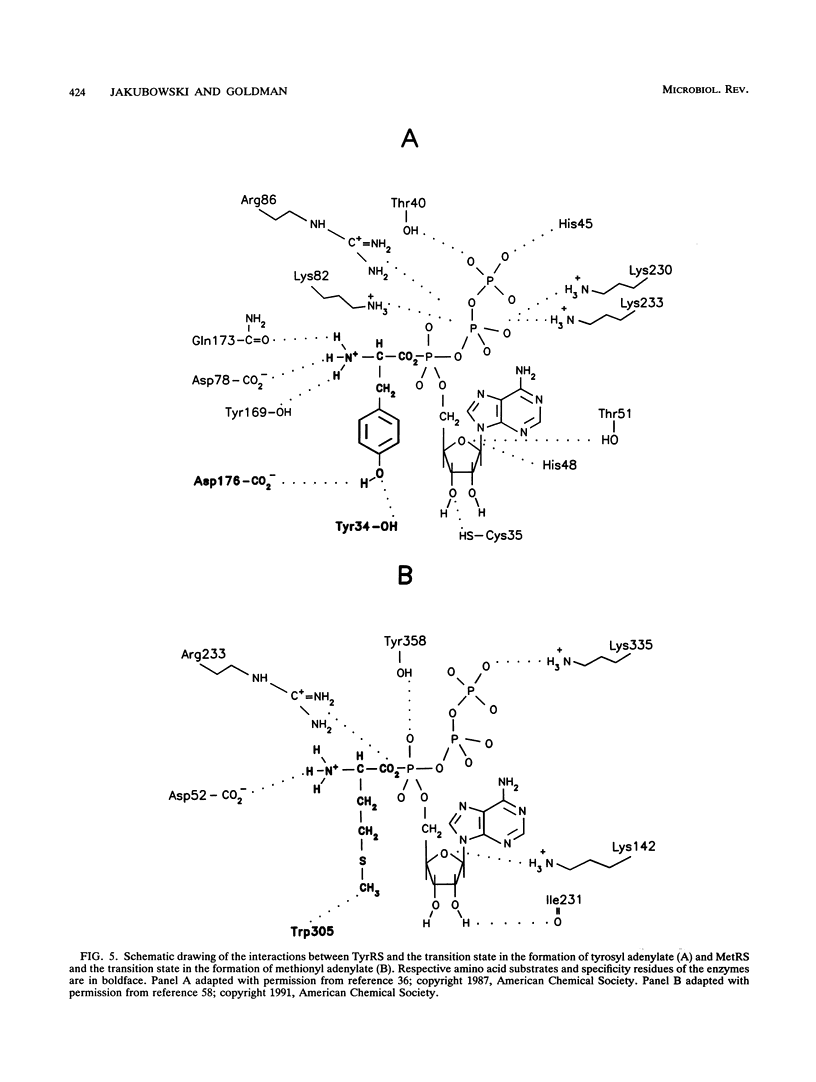
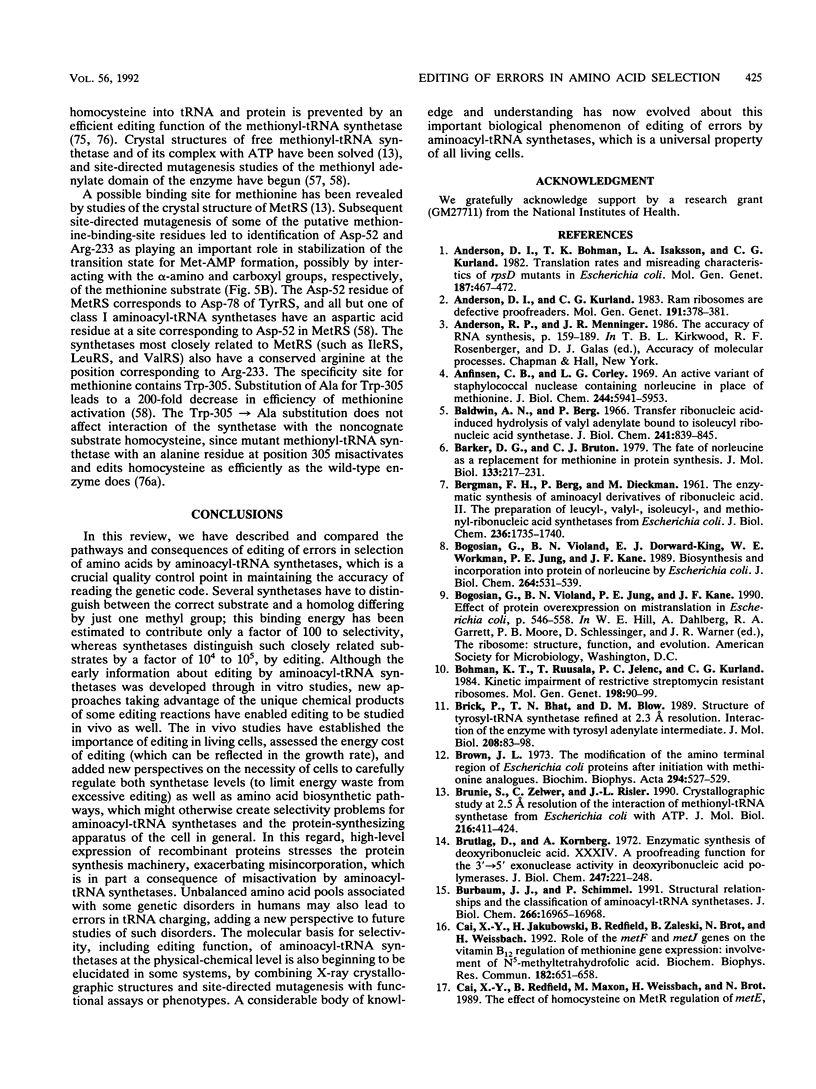
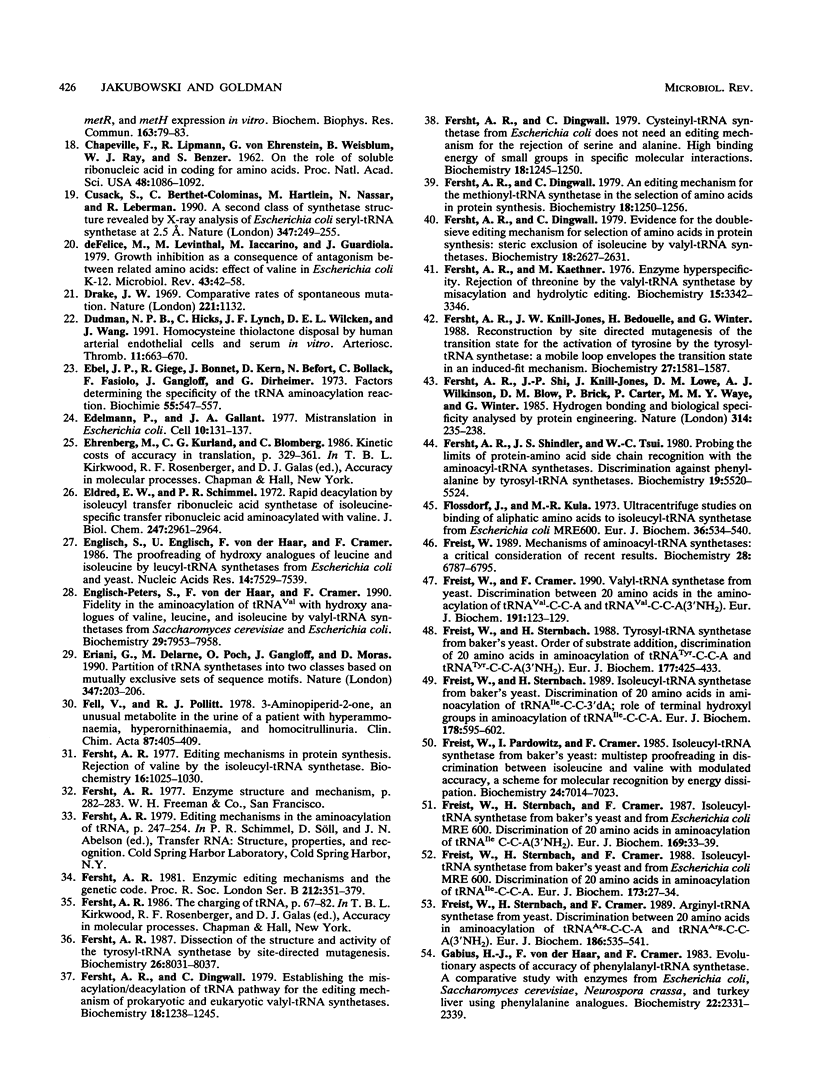
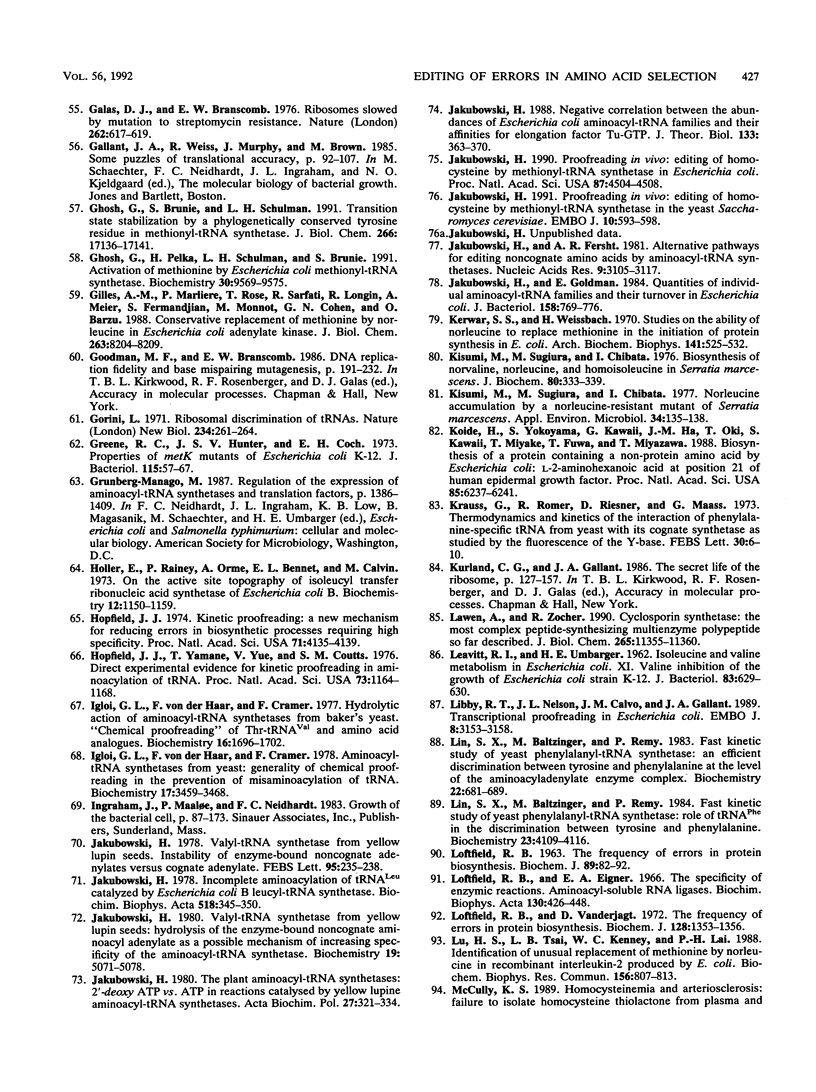
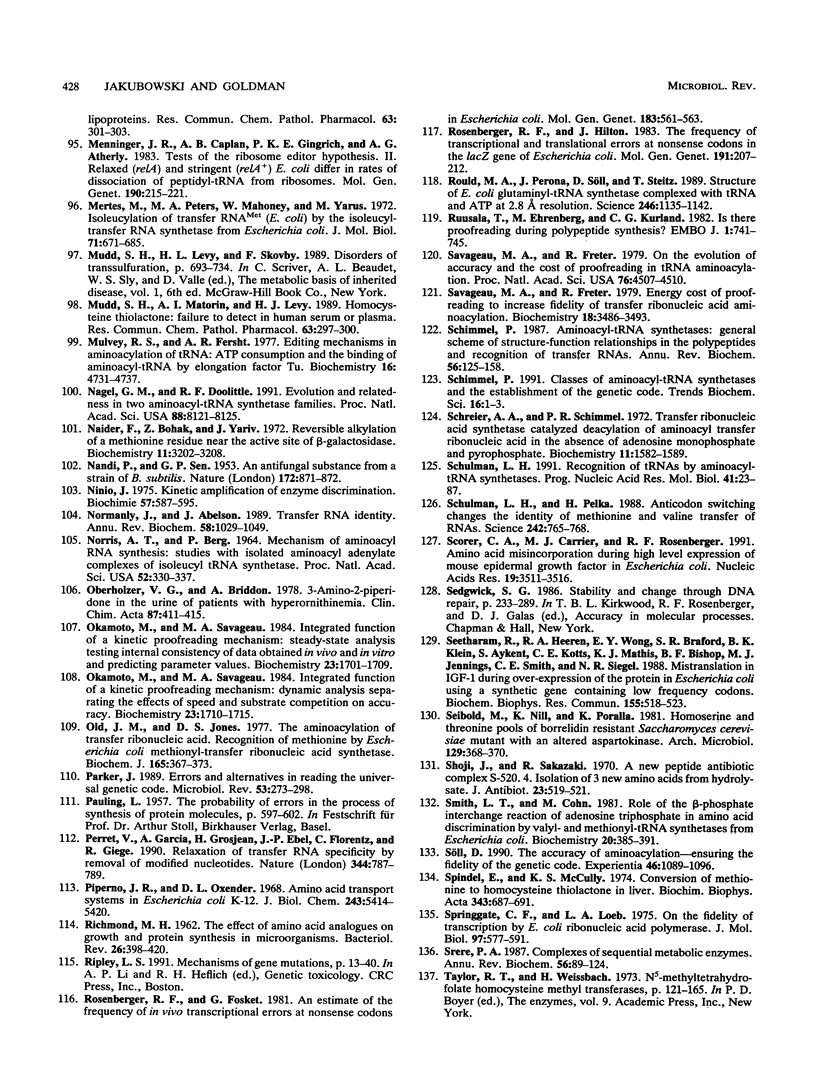
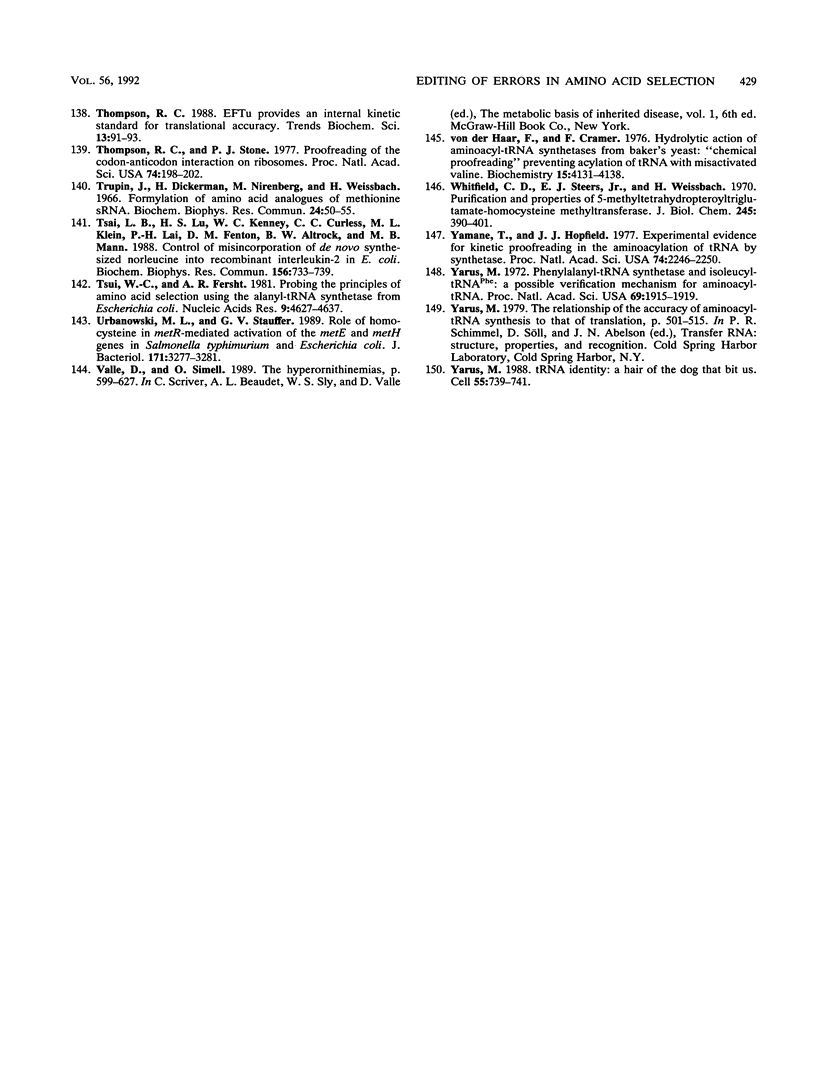
Selected References
These references are in PubMed. This may not be the complete list of references from this article.
- Andersson D. I., Bohman K., Isaksson L. A., Kurland C. G. Translation rates and misreading characteristics of rpsD mutants in Escherichia coli. Mol Gen Genet. 1982;187(3):467–472. doi: 10.1007/BF00332630. [DOI] [PubMed] [Google Scholar]
- Andersson D. I., Kurland C. G. Ram ribosomes are defective proofreaders. Mol Gen Genet. 1983;191(3):378–381. doi: 10.1007/BF00425749. [DOI] [PubMed] [Google Scholar]
- Baldwin A. N., Berg P. Transfer ribonucleic acid-induced hydrolysis of valyladenylate bound to isoleucyl ribonucleic acid synthetase. J Biol Chem. 1966 Feb 25;241(4):839–845. [PubMed] [Google Scholar]
- Barker D. G., Bruton C. J. The fate of norleucine as a replacement for methionine in protein synthesis. J Mol Biol. 1979 Sep 15;133(2):217–231. doi: 10.1016/0022-2836(79)90531-x. [DOI] [PubMed] [Google Scholar]
- Bogosian G., Violand B. N., Dorward-King E. J., Workman W. E., Jung P. E., Kane J. F. Biosynthesis and incorporation into protein of norleucine by Escherichia coli. J Biol Chem. 1989 Jan 5;264(1):531–539. [PubMed] [Google Scholar]
- Bohman K., Ruusala T., Jelenc P. C., Kurland C. G. Kinetic impairment of restrictive streptomycin-resistant ribosomes. Mol Gen Genet. 1984;198(2):90–99. doi: 10.1007/BF00328706. [DOI] [PubMed] [Google Scholar]
- Brick P., Bhat T. N., Blow D. M. Structure of tyrosyl-tRNA synthetase refined at 2.3 A resolution. Interaction of the enzyme with the tyrosyl adenylate intermediate. J Mol Biol. 1989 Jul 5;208(1):83–98. doi: 10.1016/0022-2836(89)90090-9. [DOI] [PubMed] [Google Scholar]
- Brown J. L. The modification of the amino terminal region of Escherichia coli proteins after initiation with methionine analogues. Biochim Biophys Acta. 1973 Feb 4;294(1):527–529. doi: 10.1016/0005-2787(73)90108-1. [DOI] [PubMed] [Google Scholar]
- Brunie S., Zelwer C., Risler J. L. Crystallographic study at 2.5 A resolution of the interaction of methionyl-tRNA synthetase from Escherichia coli with ATP. J Mol Biol. 1990 Nov 20;216(2):411–424. doi: 10.1016/S0022-2836(05)80331-6. [DOI] [PubMed] [Google Scholar]
- Brutlag D., Kornberg A. Enzymatic synthesis of deoxyribonucleic acid. 36. A proofreading function for the 3' leads to 5' exonuclease activity in deoxyribonucleic acid polymerases. J Biol Chem. 1972 Jan 10;247(1):241–248. [PubMed] [Google Scholar]
- Burbaum J. J., Schimmel P. Structural relationships and the classification of aminoacyl-tRNA synthetases. J Biol Chem. 1991 Sep 15;266(26):16965–16968. [PubMed] [Google Scholar]
- CHAPEVILLE F., LIPMANN F., VON EHRENSTEIN G., WEISBLUM B., RAY W. J., Jr, BENZER S. On the role of soluble ribonucleic acid in coding for amino acids. Proc Natl Acad Sci U S A. 1962 Jun 15;48:1086–1092. doi: 10.1073/pnas.48.6.1086. [DOI] [PMC free article] [PubMed] [Google Scholar]
- Cai X. Y., Jakubowski H., Redfield B., Zaleski B., Brot N., Weissbach H. Role of the metF and metJ genes on the vitamin B12 regulation of methionine gene expression: involvement of N5-methyltetrahydrofolic acid. Biochem Biophys Res Commun. 1992 Jan 31;182(2):651–658. doi: 10.1016/0006-291x(92)91782-l. [DOI] [PubMed] [Google Scholar]
- Cusack S., Berthet-Colominas C., Härtlein M., Nassar N., Leberman R. A second class of synthetase structure revealed by X-ray analysis of Escherichia coli seryl-tRNA synthetase at 2.5 A. Nature. 1990 Sep 20;347(6290):249–255. doi: 10.1038/347249a0. [DOI] [PubMed] [Google Scholar]
- De Felice M., Levinthal M., Iaccarino M., Guardiola J. Growth inhibition as a consequence of antagonism between related amino acids: effect of valine in Escherichia coli K-12. Microbiol Rev. 1979 Mar;43(1):42–58. doi: 10.1128/mr.43.1.42-58.1979. [DOI] [PMC free article] [PubMed] [Google Scholar]
- Drake J. W. Comparative rates of spontaneous mutation. Nature. 1969 Mar 22;221(5186):1132–1132. doi: 10.1038/2211132a0. [DOI] [PubMed] [Google Scholar]
- Dudman N. P., Hicks C., Lynch J. F., Wilcken D. E., Wang J. Homocysteine thiolactone disposal by human arterial endothelial cells and serum in vitro. Arterioscler Thromb. 1991 May-Jun;11(3):663–670. doi: 10.1161/01.atv.11.3.663. [DOI] [PubMed] [Google Scholar]
- Ebel J. P., Giegé R., Bonnet J., Kern D., Befort N., Bollack C., Fasiolo F., Gangloff J., Dirheimer G. Factors determining the specificity of the tRNA aminoacylation reaction. Non-absolute specificity of tRNA-aminoacyl-tRNA synthetase recognition and particular importance of the maximal velocity. Biochimie. 1973 May;55(5):547–557. doi: 10.1016/s0300-9084(73)80415-8. [DOI] [PubMed] [Google Scholar]
- Edelmann P., Gallant J. Mistranslation in E. coli. Cell. 1977 Jan;10(1):131–137. doi: 10.1016/0092-8674(77)90147-7. [DOI] [PubMed] [Google Scholar]
- Eldred E. W., Schimmel P. R. Rapid deacylation by isoleucyl transfer ribonucleic acid synthetase of isoleucine-specific transfer ribonucleic acid aminoacylated with valine. J Biol Chem. 1972 May 10;247(9):2961–2964. [PubMed] [Google Scholar]
- Englisch-Peters S., von der Haar F., Cramer F. Fidelity in the aminoacylation of tRNA(Val) with hydroxy analogues of valine, leucine, and isoleucine by valyl-tRNA synthetases from Saccharomyces cerevisiae and Escherichia coli. Biochemistry. 1990 Aug 28;29(34):7953–7958. doi: 10.1021/bi00486a025. [DOI] [PubMed] [Google Scholar]
- Englisch S., Englisch U., von der Haar F., Cramer F. The proofreading of hydroxy analogues of leucine and isoleucine by leucyl-tRNA synthetases from E. coli and yeast. Nucleic Acids Res. 1986 Oct 10;14(19):7529–7539. doi: 10.1093/nar/14.19.7529. [DOI] [PMC free article] [PubMed] [Google Scholar]
- Eriani G., Delarue M., Poch O., Gangloff J., Moras D. Partition of tRNA synthetases into two classes based on mutually exclusive sets of sequence motifs. Nature. 1990 Sep 13;347(6289):203–206. doi: 10.1038/347203a0. [DOI] [PubMed] [Google Scholar]
- Fell V., Pollitt R. J. 3-Aminopiperid-2-one, an unusual metabolite in the urine of a patient with hyperammonaemia, hyperornithinaemia and homocitrullinuria. Clin Chim Acta. 1978 Aug 1;87(3):405–409. doi: 10.1016/0009-8981(78)90185-7. [DOI] [PubMed] [Google Scholar]
- Fersht A. R., Dingwall C. An editing mechanism for the methionyl-tRNA synthetase in the selection of amino acids in protein synthesis. Biochemistry. 1979 Apr 3;18(7):1250–1256. doi: 10.1021/bi00574a021. [DOI] [PubMed] [Google Scholar]
- Fersht A. R., Dingwall C. Cysteinyl-tRNA synthetase from Escherichia coli does not need an editing mechanism to reject serine and alanine. High binding energy of small groups in specific molecular interactions. Biochemistry. 1979 Apr 3;18(7):1245–1249. doi: 10.1021/bi00574a020. [DOI] [PubMed] [Google Scholar]
- Fersht A. R., Dingwall C. Establishing the misacylation/deacylation of the tRNA pathway for the editing mechanism of prokaryotic and eukaryotic valyl-tRNA synthetases. Biochemistry. 1979 Apr 3;18(7):1238–1245. doi: 10.1021/bi00574a019. [DOI] [PubMed] [Google Scholar]
- Fersht A. R., Dingwall C. Evidence for the double-sieve editing mechanism in protein synthesis. Steric exclusion of isoleucine by valyl-tRNA synthetases. Biochemistry. 1979 Jun 12;18(12):2627–2631. doi: 10.1021/bi00579a030. [DOI] [PubMed] [Google Scholar]
- Fersht A. R. Dissection of the structure and activity of the tyrosyl-tRNA synthetase by site-directed mutagenesis. Biochemistry. 1987 Dec 15;26(25):8031–8037. doi: 10.1021/bi00399a001. [DOI] [PubMed] [Google Scholar]
- Fersht A. R. Editing mechanisms in protein synthesis. Rejection of valine by the isoleucyl-tRNA synthetase. Biochemistry. 1977 Mar 8;16(5):1025–1030. doi: 10.1021/bi00624a034. [DOI] [PubMed] [Google Scholar]
- Fersht A. R. Enzymic editing mechanisms and the genetic code. Proc R Soc Lond B Biol Sci. 1981 Aug 19;212(1189):351–379. doi: 10.1098/rspb.1981.0044. [DOI] [PubMed] [Google Scholar]
- Fersht A. R., Kaethner M. M. Enzyme hyperspecificity. Rejection of threonine by the valyl-tRNA synthetase by misacylation and hydrolytic editing. Biochemistry. 1976 Jul 27;15(15):3342–3346. doi: 10.1021/bi00660a026. [DOI] [PubMed] [Google Scholar]
- Fersht A. R., Knill-Jones J. W., Bedouelle H., Winter G. Reconstruction by site-directed mutagenesis of the transition state for the activation of tyrosine by the tyrosyl-tRNA synthetase: a mobile loop envelopes the transition state in an induced-fit mechanism. Biochemistry. 1988 Mar 8;27(5):1581–1587. doi: 10.1021/bi00405a028. [DOI] [PubMed] [Google Scholar]
- Fersht A. R., Shi J. P., Knill-Jones J., Lowe D. M., Wilkinson A. J., Blow D. M., Brick P., Carter P., Waye M. M., Winter G. Hydrogen bonding and biological specificity analysed by protein engineering. Nature. 1985 Mar 21;314(6008):235–238. doi: 10.1038/314235a0. [DOI] [PubMed] [Google Scholar]
- Fersht A. R., Shindler J. S., Tsui W. C. Probing the limits of protein-amino acid side chain recognition with the aminoacyl-tRNA synthetases. Discrimination against phenylalanine by tyrosyl-tRNA synthetases. Biochemistry. 1980 Nov 25;19(24):5520–5524. doi: 10.1021/bi00565a009. [DOI] [PubMed] [Google Scholar]
- Flossdorf J., Kula M. R. Ultracentrifuge studies on binding of aliphatic amino acids to isoleucyl-tRNA synthetase from Escherichia coli MRE 600. Eur J Biochem. 1973 Jul 16;36(2):534–540. doi: 10.1111/j.1432-1033.1973.tb02940.x. [DOI] [PubMed] [Google Scholar]
- Freist W., Cramer F. Valyl-tRNA synthetase from yeast. Discrimination between 20 amino acids in aminoacylation of tRNA(Val)-C-C-A and tRNA(Val)-C-C-A(3'NH2). Eur J Biochem. 1990 Jul 20;191(1):123–129. doi: 10.1111/j.1432-1033.1990.tb19101.x. [DOI] [PubMed] [Google Scholar]
- Freist W. Mechanisms of aminoacyl-tRNA synthetases: a critical consideration of recent results. Biochemistry. 1989 Aug 22;28(17):6787–6795. doi: 10.1021/bi00443a001. [DOI] [PubMed] [Google Scholar]
- Freist W., Pardowitz I., Cramer F. Isoleucyl-tRNA synthetase from bakers' yeast: multistep proofreading in discrimination between isoleucine and valine with modulated accuracy, a scheme for molecular recognition by energy dissipation. Biochemistry. 1985 Nov 19;24(24):7014–7023. doi: 10.1021/bi00345a040. [DOI] [PubMed] [Google Scholar]
- Freist W., Sternbach H., Cramer F. Arginyl-tRNA synthetase from yeast. Discrimination between 20 amino acids in aminoacylation of tRNA(Arg)-C-C-A and tRNA(Arg)-C-C-A(3'NH2). Eur J Biochem. 1989 Dec 22;186(3):535–541. doi: 10.1111/j.1432-1033.1989.tb15239.x. [DOI] [PubMed] [Google Scholar]
- Freist W., Sternbach H., Cramer F. Isoleucyl-tRNA synthetase from baker's yeast and from Escherichia coli MRE 600. Discrimination of 20 amino acids in aminoacylation of tRNA(Ile)-C-C-A(3'NH2). Eur J Biochem. 1987 Nov 16;169(1):33–39. doi: 10.1111/j.1432-1033.1987.tb13577.x. [DOI] [PubMed] [Google Scholar]
- Freist W., Sternbach H., Cramer F. Isoleucyl-tRNA synthetase from baker's yeast and from Escherichia coli MRE 600. Discrimination of 20 amino acids in aminoacylation of tRNA(Ile)-C-C-A. Eur J Biochem. 1988 Apr 5;173(1):27–34. doi: 10.1111/j.1432-1033.1988.tb13962.x. [DOI] [PubMed] [Google Scholar]
- Freist W., Sternbach H. Isoleucyl-tRNA synthetase from baker's yeast. Discrimination of 20 amino acids in aminoacylation of tRNA(Ile)-C-C-3'dA; role of terminal hydroxyl groups aminoacylation of tRNA(Ile)-C-C-A. Eur J Biochem. 1989 Jan 2;178(3):595–602. doi: 10.1111/j.1432-1033.1989.tb14487.x. [DOI] [PubMed] [Google Scholar]
- Freist W., Sternbach H. Tyrosyl-tRNA synthetase from baker's yeast. Order of substrate addition, discrimination of 20 amino acids in aminoacylation of tRNATyr-C-C-A and tRNATyr-C-C-A(3'NH2). Eur J Biochem. 1988 Nov 1;177(2):425–433. doi: 10.1111/j.1432-1033.1988.tb14392.x. [DOI] [PubMed] [Google Scholar]
- Gabius H. J., von der Haar F., Cramer F. Evolutionary aspects of accuracy of phenylalanyl-tRNA synthetase. A comparative study with enzymes from Escherichia coli, Saccharomyces cerevisiae, Neurospora crassa, and turkey liver using phenylalanine analogues. Biochemistry. 1983 May 10;22(10):2331–2339. doi: 10.1021/bi00279a005. [DOI] [PubMed] [Google Scholar]
- Galas D. J., Branscomb E. W. Ribosome slowed by mutation to streptomycin resistance. Nature. 1976 Aug 12;262(5569):617–619. doi: 10.1038/262617b0. [DOI] [PubMed] [Google Scholar]
- Ghosh G., Brunie S., Schulman L. H. Transition state stabilization by a phylogenetically conserved tyrosine residue in methionyl-tRNA synthetase. J Biol Chem. 1991 Sep 15;266(26):17136–17141. [PubMed] [Google Scholar]
- Ghosh G., Pelka H., Schulman L. H., Brunie S. Activation of methionine by Escherichia coli methionyl-tRNA synthetase. Biochemistry. 1991 Oct 8;30(40):9569–9575. doi: 10.1021/bi00104a002. [DOI] [PubMed] [Google Scholar]
- Gilles A. M., Marlière P., Rose T., Sarfati R., Longin R., Meier A., Fermandjian S., Monnot M., Cohen G. N., Bârzu O. Conservative replacement of methionine by norleucine in Escherichia coli adenylate kinase. J Biol Chem. 1988 Jun 15;263(17):8204–8209. [PubMed] [Google Scholar]
- Gorini L. Ribosomal discrimination of tRNAs. Nat New Biol. 1971 Dec 29;234(52):261–264. doi: 10.1038/newbio234261a0. [DOI] [PubMed] [Google Scholar]
- Greene R. C., Hunter J. S., Coch E. H. Properties of metK mutants of Escherichia coli K-12. J Bacteriol. 1973 Jul;115(1):57–67. doi: 10.1128/jb.115.1.57-67.1973. [DOI] [PMC free article] [PubMed] [Google Scholar]
- Holler E., Rainey P., Orme A., Bennett E. L., Calvin M. On the active site topography of isoleucyl transfer ribonucleic acid synthetase of Escherichia coli B. Biochemistry. 1973 Mar 13;12(6):1150–1159. doi: 10.1021/bi00730a021. [DOI] [PubMed] [Google Scholar]
- Hopfield J. J. Kinetic proofreading: a new mechanism for reducing errors in biosynthetic processes requiring high specificity. Proc Natl Acad Sci U S A. 1974 Oct;71(10):4135–4139. doi: 10.1073/pnas.71.10.4135. [DOI] [PMC free article] [PubMed] [Google Scholar]
- Hopfield J. J., Yamane T., Yue V., Coutts S. M. Direct experimental evidence for kinetic proofreading in amino acylation of tRNAIle. Proc Natl Acad Sci U S A. 1976 Apr;73(4):1164–1168. doi: 10.1073/pnas.73.4.1164. [DOI] [PMC free article] [PubMed] [Google Scholar]
- Igloi G. L., von der Haar F., Cramer F. Aminoacyl-tRNA synthetases from yeast: generality of chemical proofreading in the prevention of misaminoacylation of tRNA. Biochemistry. 1978 Aug 22;17(17):3459–3468. doi: 10.1021/bi00610a006. [DOI] [PubMed] [Google Scholar]
- Igloi G. L., von der Haar F., Cramer F. Hydrolytic action of aminoacyl-tRNA synthetases from baker's yeast. "Chemical proofreading" of Thr-tRNA Val by valyl-tRNA synthetase studied with modified tRNA Val and amino acid analogues. Biochemistry. 1977 Apr 19;16(8):1696–1702. doi: 10.1021/bi00627a027. [DOI] [PubMed] [Google Scholar]
- Jakubowski H., Fersht A. R. Alternative pathways for editing non-cognate amino acids by aminoacyl-tRNA synthetases. Nucleic Acids Res. 1981 Jul 10;9(13):3105–3117. doi: 10.1093/nar/9.13.3105. [DOI] [PMC free article] [PubMed] [Google Scholar]
- Jakubowski H., Goldman E. Quantities of individual aminoacyl-tRNA families and their turnover in Escherichia coli. J Bacteriol. 1984 Jun;158(3):769–776. doi: 10.1128/jb.158.3.769-776.1984. [DOI] [PMC free article] [PubMed] [Google Scholar]
- Jakubowski H. Incomplete aminoacylation of tRNALeu catalyzed in vitro by leucyl-tRNA synthetase from Escherichia coli B. Biochim Biophys Acta. 1978 Apr 27;518(2):345–350. doi: 10.1016/0005-2787(78)90191-0. [DOI] [PubMed] [Google Scholar]
- Jakubowski H. Negative correlation between the abundance of Escherichia coli aminoacyl-tRNA families and their affinities for elongation factor Tu-GTP. J Theor Biol. 1988 Aug 8;133(3):363–370. doi: 10.1016/s0022-5193(88)80327-8. [DOI] [PubMed] [Google Scholar]
- Jakubowski H. Proofreading in vivo: editing of homocysteine by methionyl-tRNA synthetase in Escherichia coli. Proc Natl Acad Sci U S A. 1990 Jun;87(12):4504–4508. doi: 10.1073/pnas.87.12.4504. [DOI] [PMC free article] [PubMed] [Google Scholar]
- Jakubowski H. Proofreading in vivo: editing of homocysteine by methionyl-tRNA synthetase in the yeast Saccharomyces cerevisiae. EMBO J. 1991 Mar;10(3):593–598. doi: 10.1002/j.1460-2075.1991.tb07986.x. [DOI] [PMC free article] [PubMed] [Google Scholar]
- Jakubowski H. The plant aminoacyl-tRNA synthetases. 2'-DeoxyATP and ATP in reactions catalysed by yellow lupin aminoacyl-tRNA synthetases. Acta Biochim Pol. 1980;27(3-4):321–333. [PubMed] [Google Scholar]
- Jakubowski H. Valyl-tRNA synthetase form yellow lupin seeds: hydrolysis of the enzyme-bound noncognate aminoacyl adenylate as a possible mechanism of increasing specificity of the aminoacyl-tRNA synthetase. Biochemistry. 1980 Oct 28;19(22):5071–5078. doi: 10.1021/bi00563a021. [DOI] [PubMed] [Google Scholar]
- Jakubowski H. Valyl-tRNA synthetase from yellow lupin seeds. Instability of enzyme-bound noncognate adenylates versus cognate adenylate. FEBS Lett. 1978 Nov 15;95(2):235–238. doi: 10.1016/0014-5793(78)81001-1. [DOI] [PubMed] [Google Scholar]
- Kerwar S. S., Weissbach H. Studies on the ability of norleucine to replace methionine in the initiation of protein synthesis of E. coli. Arch Biochem Biophys. 1970 Dec;141(2):525–532. doi: 10.1016/0003-9861(70)90170-0. [DOI] [PubMed] [Google Scholar]
- Kisumi M., Sugiura M., Chibata I. Biosynthesis of norvaline, norleucine, and homoisoleucine in Serratia marcescens. J Biochem. 1976 Aug;80(2):333–339. doi: 10.1093/oxfordjournals.jbchem.a131281. [DOI] [PubMed] [Google Scholar]
- Kisumi M., Sugiura M., Chibata I. Norleucine accumulation by a norleucine-resistant mutant of Serratia marcescens. Appl Environ Microbiol. 1977 Aug;34(2):135–138. doi: 10.1128/aem.34.2.135-138.1977. [DOI] [PMC free article] [PubMed] [Google Scholar]
- Koide H., Yokoyama S., Kawai G., Ha J. M., Oka T., Kawai S., Miyake T., Fuwa T., Miyazawa T. Biosynthesis of a protein containing a nonprotein amino acid by Escherichia coli: L-2-aminohexanoic acid at position 21 in human epidermal growth factor. Proc Natl Acad Sci U S A. 1988 Sep;85(17):6237–6241. doi: 10.1073/pnas.85.17.6237. [DOI] [PMC free article] [PubMed] [Google Scholar]
- Krauss G., Römer R., Riesner D., Maass G. Thermodynamics and kinetics of the interaction of phenylalanine-specific tRNA from yeast with its cognate synthetase as studied by the flourescence of the Y-base. FEBS Lett. 1973 Feb 15;30(1):6–10. doi: 10.1016/0014-5793(73)80606-4. [DOI] [PubMed] [Google Scholar]
- LEAVITT R. I., UMBARGER H. E. Isoleucine and valine metabolism in Escherichia coli. XI. Valine inhibition of the growth of Escherichia coli strain K-12. J Bacteriol. 1962 Mar;83:624–630. doi: 10.1128/jb.83.3.624-630.1962. [DOI] [PMC free article] [PubMed] [Google Scholar]
- LOFTFIELD R. B. THE FREQUENCY OF ERRORS IN PROTEIN BIOSYNTHESIS. Biochem J. 1963 Oct;89:82–92. doi: 10.1042/bj0890082. [DOI] [PMC free article] [PubMed] [Google Scholar]
- Lawen A., Zocher R. Cyclosporin synthetase. The most complex peptide synthesizing multienzyme polypeptide so far described. J Biol Chem. 1990 Jul 5;265(19):11355–11360. [PubMed] [Google Scholar]
- Libby R. T., Nelson J. L., Calvo J. M., Gallant J. A. Transcriptional proofreading in Escherichia coli. EMBO J. 1989 Oct;8(10):3153–3158. doi: 10.1002/j.1460-2075.1989.tb08469.x. [DOI] [PMC free article] [PubMed] [Google Scholar]
- Lin S. X., Baltzinger M., Remy P. Fast kinetic study of yeast phenylalanyl-tRNA synthetase: an efficient discrimination between tyrosine and phenylalanine at the level of the aminoacyladenylate-enzyme complex. Biochemistry. 1983 Feb 1;22(3):681–689. doi: 10.1021/bi00272a024. [DOI] [PubMed] [Google Scholar]
- Lin S. X., Baltzinger M., Remy P. Fast kinetic study of yeast phenylalanyl-tRNA synthetase: role of tRNAPhe in the discrimination between tyrosine and phenylalanine. Biochemistry. 1984 Aug 28;23(18):4109–4116. doi: 10.1021/bi00313a015. [DOI] [PubMed] [Google Scholar]
- Loftfield R. B., Eigner E. A. The specificity of enzymic reactions. Aminoacyl-soluble RNA ligases. Biochim Biophys Acta. 1966 Dec 28;130(2):426–448. doi: 10.1016/0304-4165(66)90239-x. [DOI] [PubMed] [Google Scholar]
- Loftfield R. B., Vanderjagt D. The frequency of errors in protein biosynthesis. Biochem J. 1972 Aug;128(5):1353–1356. doi: 10.1042/bj1281353. [DOI] [PMC free article] [PubMed] [Google Scholar]
- Lu H. S., Tsai L. B., Kenney W. C., Lai P. H. Identification of unusual replacement of methionine by norleucine in recombinant interleukin-2 produced by E. coli. Biochem Biophys Res Commun. 1988 Oct 31;156(2):807–813. doi: 10.1016/s0006-291x(88)80916-1. [DOI] [PubMed] [Google Scholar]
- Menninger J. R., Caplan A. B., Gingrich P. K., Atherly A. G. Tests of the ribosome editor hypothesis. II. Relaxed (relA) and stringent (relA+) E. coli differ in rates of dissociation of peptidyl-tRNA from ribosomes. Mol Gen Genet. 1983;190(2):215–221. doi: 10.1007/BF00330642. [DOI] [PubMed] [Google Scholar]
- Mertes M., Peters M. A., Mahoney W., Yarus M. Isoleucylation of transfer RNA f Met (E. coli) by isoleucyl-transfer RNA synthetase from Escherichia coli. J Mol Biol. 1972 Nov 28;71(3):671–685. doi: 10.1016/s0022-2836(72)80031-7. [DOI] [PubMed] [Google Scholar]
- Mudd S. H., Matorin A. I., Levy H. L. Homocysteine thiolactone: failure to detect in human serum or plasma. Res Commun Chem Pathol Pharmacol. 1989 Feb;63(2):297–300. [PubMed] [Google Scholar]
- Mulvey R. S., Fersht A. R. Editing mechanisms in aminoacylation of tRNA: ATP consumption and the binding of aminoacyl-tRNA by elongation factor Tu. Biochemistry. 1977 Oct 18;16(21):4731–4737. doi: 10.1021/bi00640a031. [DOI] [PubMed] [Google Scholar]
- NANDI P., SEN G. P. An antifungal substance from a strain of B subtilis. Nature. 1953 Nov 7;172(4384):871–872. doi: 10.1038/172871b0. [DOI] [PubMed] [Google Scholar]
- NORRIS A. T., BERG P. MECHANISM OF AMINOACYL RNA SYNTHESIS: STUDIES WITH ISOLATED AMINOACYL ADENYLATE COMPLEXES OF ISOLEUCYL RNA SYNTHETASE. Proc Natl Acad Sci U S A. 1964 Aug;52:330–337. doi: 10.1073/pnas.52.2.330. [DOI] [PMC free article] [PubMed] [Google Scholar]
- Nagel G. M., Doolittle R. F. Evolution and relatedness in two aminoacyl-tRNA synthetase families. Proc Natl Acad Sci U S A. 1991 Sep 15;88(18):8121–8125. doi: 10.1073/pnas.88.18.8121. [DOI] [PMC free article] [PubMed] [Google Scholar]
- Naider F., Bohak Z., Yariv J. Reversible alkylation of a methionyl residue near the active site of -galactosidase. Biochemistry. 1972 Aug 15;11(17):3202–3208. doi: 10.1021/bi00767a010. [DOI] [PubMed] [Google Scholar]
- Ninio J. Kinetic amplification of enzyme discrimination. Biochimie. 1975;57(5):587–595. doi: 10.1016/s0300-9084(75)80139-8. [DOI] [PubMed] [Google Scholar]
- Normanly J., Abelson J. tRNA identity. Annu Rev Biochem. 1989;58:1029–1049. doi: 10.1146/annurev.bi.58.070189.005121. [DOI] [PubMed] [Google Scholar]
- Oberholzer V. G., Briddon A. 3-Amino-2-piperidone in the urine of patients with hyperornithinemia. Clin Chim Acta. 1978 Aug 1;87(3):411–415. doi: 10.1016/0009-8981(78)90186-9. [DOI] [PubMed] [Google Scholar]
- Okamoto M., Savageau M. A. Integrated function of a kinetic proofreading mechanism: dynamic analysis separating the effects of speed and substrate competition on accuracy. Biochemistry. 1984 Apr 10;23(8):1710–1715. doi: 10.1021/bi00303a020. [DOI] [PubMed] [Google Scholar]
- Okamoto M., Savageau M. A. Integrated function of a kinetic proofreading mechanism: steady-state analysis testing internal consistency of data obtained in vivo and in vitro and predicting parameter values. Biochemistry. 1984 Apr 10;23(8):1701–1709. doi: 10.1021/bi00303a019. [DOI] [PubMed] [Google Scholar]
- Old J. M., Jones D. S. The aminoacylation of transfer ribonucleic acid. Recognition of methionine by Escherichia coli methionyl-transfer ribonucleic acid synthetase. Biochem J. 1977 Aug 1;165(2):367–373. doi: 10.1042/bj1650367. [DOI] [PMC free article] [PubMed] [Google Scholar]
- Parker J. Errors and alternatives in reading the universal genetic code. Microbiol Rev. 1989 Sep;53(3):273–298. doi: 10.1128/mr.53.3.273-298.1989. [DOI] [PMC free article] [PubMed] [Google Scholar]
- Perret V., Garcia A., Grosjean H., Ebel J. P., Florentz C., Giegé R. Relaxation of a transfer RNA specificity by removal of modified nucleotides. Nature. 1990 Apr 19;344(6268):787–789. doi: 10.1038/344787a0. [DOI] [PubMed] [Google Scholar]
- RICHMOND M. H. The effect of amino acid analogues on growth and protein synthesis in microorganisms. Bacteriol Rev. 1962 Dec;26:398–420. doi: 10.1128/br.26.4.398-420.1962. [DOI] [PMC free article] [PubMed] [Google Scholar]
- Rosenberger R. F., Foskett G. An estimate of the frequency of in vivo transcriptional errors at a nonsense codon in Escherichia coli. Mol Gen Genet. 1981;183(3):561–563. doi: 10.1007/BF00268784. [DOI] [PubMed] [Google Scholar]
- Rosenberger R. F., Hilton J. The frequency of transcriptional and translational errors at nonsense codons in the lacZ gene of Escherichia coli. Mol Gen Genet. 1983;191(2):207–212. doi: 10.1007/BF00334815. [DOI] [PubMed] [Google Scholar]
- Rould M. A., Perona J. J., Söll D., Steitz T. A. Structure of E. coli glutaminyl-tRNA synthetase complexed with tRNA(Gln) and ATP at 2.8 A resolution. Science. 1989 Dec 1;246(4934):1135–1142. doi: 10.1126/science.2479982. [DOI] [PubMed] [Google Scholar]
- Ruusala T., Ehrenberg M., Kurland C. G. Is there proofreading during polypeptide synthesis? EMBO J. 1982;1(6):741–745. doi: 10.1002/j.1460-2075.1982.tb01240.x. [DOI] [PMC free article] [PubMed] [Google Scholar]
- Savageau M. A., Freter R. R. Energy cost of proofreading to increase fidelity of transfer ribonucleic acid aminoacylation. Biochemistry. 1979 Aug 7;18(16):3486–3493. doi: 10.1021/bi00583a008. [DOI] [PubMed] [Google Scholar]
- Savageau M. A., Freter R. R. On the evolution of accuracy and cost of proofreading tRNA aminoacylation. Proc Natl Acad Sci U S A. 1979 Sep;76(9):4507–4510. doi: 10.1073/pnas.76.9.4507. [DOI] [PMC free article] [PubMed] [Google Scholar]
- Schimmel P. Aminoacyl tRNA synthetases: general scheme of structure-function relationships in the polypeptides and recognition of transfer RNAs. Annu Rev Biochem. 1987;56:125–158. doi: 10.1146/annurev.bi.56.070187.001013. [DOI] [PubMed] [Google Scholar]
- Schimmel P. Classes of aminoacyl-tRNA synthetases and the establishment of the genetic code. Trends Biochem Sci. 1991 Jan;16(1):1–3. doi: 10.1016/0968-0004(91)90002-d. [DOI] [PubMed] [Google Scholar]
- Schreier A. A., Schimmel P. R. Transfer ribonucleic acid synthetase catalyzed deacylation of aminoacyl transfer ribonucleic acid in the absence of adenosine monophosphate and pyrophosphate. Biochemistry. 1972 Apr 25;11(9):1582–1589. doi: 10.1021/bi00759a006. [DOI] [PubMed] [Google Scholar]
- Schulman L. H., Pelka H. Anticodon switching changes the identity of methionine and valine transfer RNAs. Science. 1988 Nov 4;242(4879):765–768. doi: 10.1126/science.3055296. [DOI] [PubMed] [Google Scholar]
- Schulman L. H. Recognition of tRNAs by aminoacyl-tRNA synthetases. Prog Nucleic Acid Res Mol Biol. 1991;41:23–87. [PubMed] [Google Scholar]
- Scorer C. A., Carrier M. J., Rosenberger R. F. Amino acid misincorporation during high-level expression of mouse epidermal growth factor in Escherichia coli. Nucleic Acids Res. 1991 Jul 11;19(13):3511–3516. doi: 10.1093/nar/19.13.3511. [DOI] [PMC free article] [PubMed] [Google Scholar]
- Seetharam R., Heeren R. A., Wong E. Y., Braford S. R., Klein B. K., Aykent S., Kotts C. E., Mathis K. J., Bishop B. F., Jennings M. J. Mistranslation in IGF-1 during over-expression of the protein in Escherichia coli using a synthetic gene containing low frequency codons. Biochem Biophys Res Commun. 1988 Aug 30;155(1):518–523. doi: 10.1016/s0006-291x(88)81117-3. [DOI] [PubMed] [Google Scholar]
- Seibold M., Nill K., Poralla K. Homoserine and threonine pools of borrelidin resistant Saccharomyces cerevisiae mutants with an altered aspartokinase. Arch Microbiol. 1981 Jul;129(5):368–370. doi: 10.1007/BF00406464. [DOI] [PubMed] [Google Scholar]
- Shoji J., Sakazaki R. A new peptide antibiotic complex S-520. IV. Isolation of three new amino acids from the hydrolysate. J Antibiot (Tokyo) 1970 Oct;23(10):519–521. doi: 10.7164/antibiotics.23.519. [DOI] [PubMed] [Google Scholar]
- Spindel E., McCully K. S. Conversion of methionine to homocysteine thiolactone in liver. Biochim Biophys Acta. 1974 May 24;343(3):687–691. doi: 10.1016/0304-4165(74)90290-6. [DOI] [PubMed] [Google Scholar]
- Springgate C. F., Loeb L. A. On the fidelity of transcription by Escherichia coli ribonucleic acid polymerase. J Mol Biol. 1975 Oct 5;97(4):577–591. doi: 10.1016/s0022-2836(75)80060-x. [DOI] [PubMed] [Google Scholar]
- Srere P. A. Complexes of sequential metabolic enzymes. Annu Rev Biochem. 1987;56:89–124. doi: 10.1146/annurev.bi.56.070187.000513. [DOI] [PubMed] [Google Scholar]
- Söll D. The accuracy of aminoacylation--ensuring the fidelity of the genetic code. Experientia. 1990 Dec 1;46(11-12):1089–1096. doi: 10.1007/BF01936918. [DOI] [PubMed] [Google Scholar]
- Thompson R. C. EFTu provides an internal kinetic standard for translational accuracy. Trends Biochem Sci. 1988 Mar;13(3):91–93. doi: 10.1016/0968-0004(88)90047-3. [DOI] [PubMed] [Google Scholar]
- Thompson R. C., Stone P. J. Proofreading of the codon-anticodon interaction on ribosomes. Proc Natl Acad Sci U S A. 1977 Jan;74(1):198–202. doi: 10.1073/pnas.74.1.198. [DOI] [PMC free article] [PubMed] [Google Scholar]
- Trupin J., Dickerman H., Nirenberg M., Weissbach H. Formylation of amino acid analogues of methionine sRNA. Biochem Biophys Res Commun. 1966 Jul 6;24(1):50–55. doi: 10.1016/0006-291x(66)90408-6. [DOI] [PubMed] [Google Scholar]
- Tsai L. B., Lu H. S., Kenney W. C., Curless C. C., Klein M. L., Lai P. H., Fenton D. M., Altrock B. W., Mann M. B. Control of misincorporation of de novo synthesized norleucine into recombinant interleukin-2 in E. coli. Biochem Biophys Res Commun. 1988 Oct 31;156(2):733–739. doi: 10.1016/s0006-291x(88)80904-5. [DOI] [PubMed] [Google Scholar]
- Tsui W. C., Fersht A. R. Probing the principles of amino acid selection using the alanyl-tRNA synthetase from Escherichia coli. Nucleic Acids Res. 1981 Sep 25;9(18):4627–4637. doi: 10.1093/nar/9.18.4627. [DOI] [PMC free article] [PubMed] [Google Scholar]
- Urbanowski M. L., Stauffer G. V. Role of homocysteine in metR-mediated activation of the metE and metH genes in Salmonella typhimurium and Escherichia coli. J Bacteriol. 1989 Jun;171(6):3277–3281. doi: 10.1128/jb.171.6.3277-3281.1989. [DOI] [PMC free article] [PubMed] [Google Scholar]
- Whitfield C. D., Steers E. J., Jr, Weissbach H. Purification and properties of 5-methyltetrahydropteroyltriglutamate-homocysteine transmethylase. J Biol Chem. 1970 Jan 25;245(2):390–401. [PubMed] [Google Scholar]
- Yamane T., Hopfield J. J. Experimental evidence for kinetic proofreading in the aminoacylation of tRNA by synthetase. Proc Natl Acad Sci U S A. 1977 Jun;74(6):2246–2250. doi: 10.1073/pnas.74.6.2246. [DOI] [PMC free article] [PubMed] [Google Scholar]
- Yarus M. Phenylalanyl-tRNA synthetase and isoleucyl-tRNA Phe : a possible verification mechanism for aminoacyl-tRNA. Proc Natl Acad Sci U S A. 1972 Jul;69(7):1915–1919. doi: 10.1073/pnas.69.7.1915. [DOI] [PMC free article] [PubMed] [Google Scholar]
- Yarus M. tRNA identity: a hair of the dogma that bit us. Cell. 1988 Dec 2;55(5):739–741. doi: 10.1016/0092-8674(88)90127-4. [DOI] [PubMed] [Google Scholar]
- von der Haar F., Cramer F. Hydrolytic action of aminoacyl-tRNA synthetases from baker's yeast: "chemical proofreading" preventing acylation of tRNA(I1e) with misactivated valine. Biochemistry. 1976 Sep 7;15(18):4131–4138. doi: 10.1021/bi00663a034. [DOI] [PubMed] [Google Scholar]