Abstract
Residue 225 in serine proteases is typically Pro or Tyr and specifies an important and unanticipated functional aspect of this class of enzymes. Proteases with Y225, like thrombin, are involved in highly specialized functions like blood coagulation and complement that are exclusively found in vertebrates. In these proteases, the catalytic activity is enhanced allosterically by Na+ binding. Proteases with P225, like trypsin, are typically involved in digestive functions and are also found in organisms as primitive as eubacteria. These proteases have no requirement for Na+ or other monovalent cations. The molecular origin of this physiologically important difference is remarkably simple and is revealed by a comparison of the Na+ binding loop of thrombin with the homologous region of trypsin. The carbonyl O atom of residue 224 makes a key contribution to the coordination shell of the bound Na+ in thrombin, but is oriented in a manner incompatible with Na+ binding in trypsin because of constraints imposed by P225 on the protein backbone. Pro at position 225 is therefore incompatible with Na+ binding and is a direct predictor of the lack of allosteric regulation in serine proteases. To directly test this hypothesis, we have engineered the thrombin mutant Y225P. This mutant has lost the ability to bind Na+ and behaves like the allosteric slow (Na(+)-free) form. The Na(+)-induced allosteric regulation also bears on the molecular evolution of serine proteases. A strong correlation exists between residue 225 and the codon used for the active site S195. Proteases with P225 typically use a TCN codon for S195, whereas proteases with Y225 use an AGY codon. It is proposed that serine proteases evolved from two main lineages: (i) TCN/P225 with a trypsin-like ancestor and (ii) AGY/Y225 with a thrombin-like ancestor. We predict that the Na(+)-induced allosteric regulation of catalytic activity can be introduced in the TCN/P225 lineage using the P225Y replacement.
Full text
PDF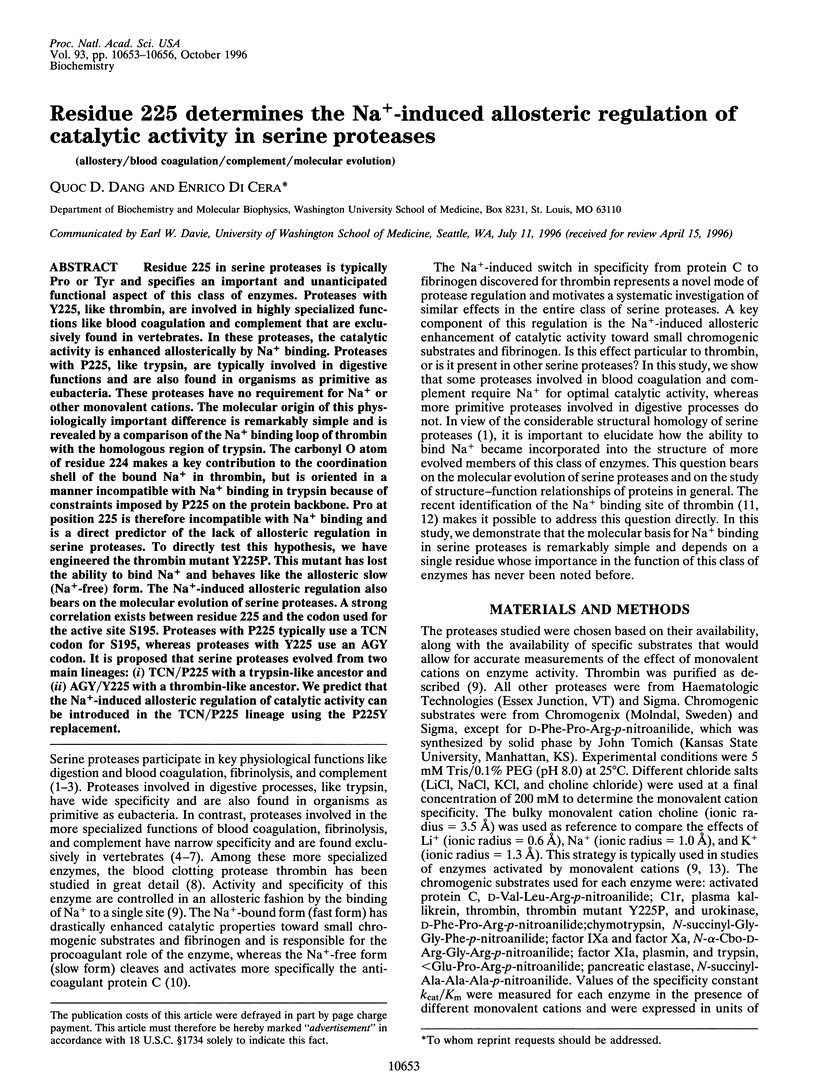
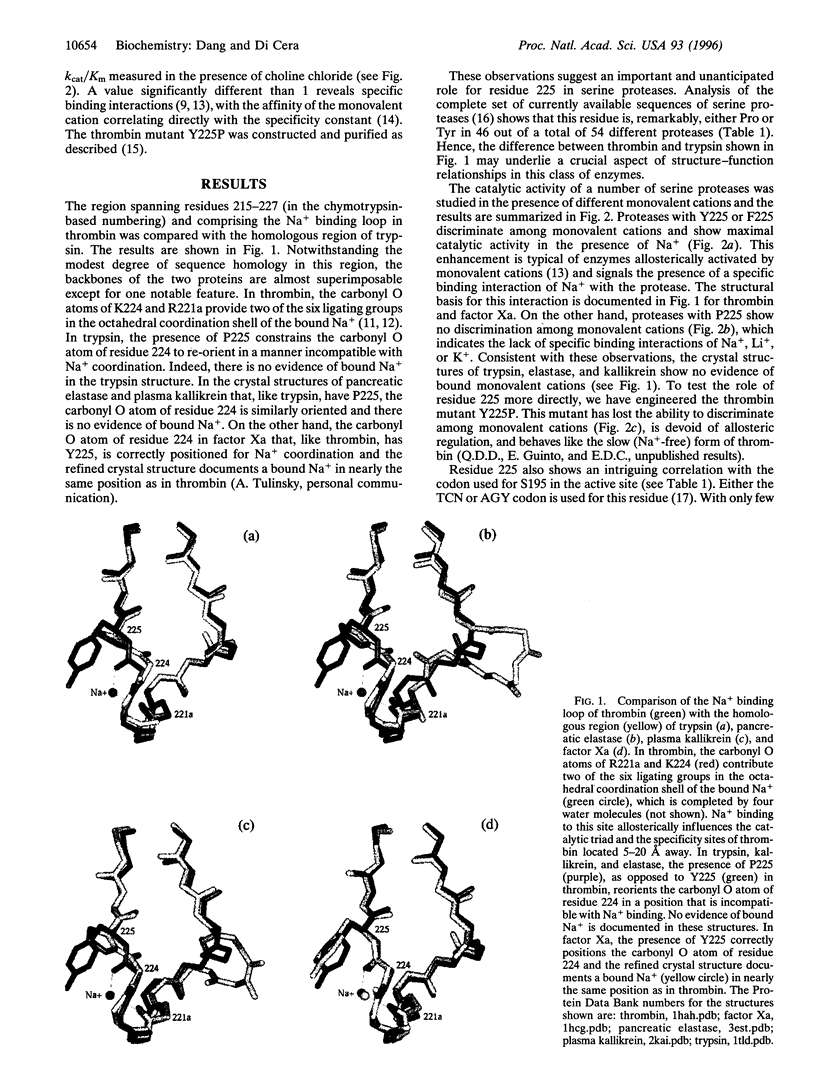
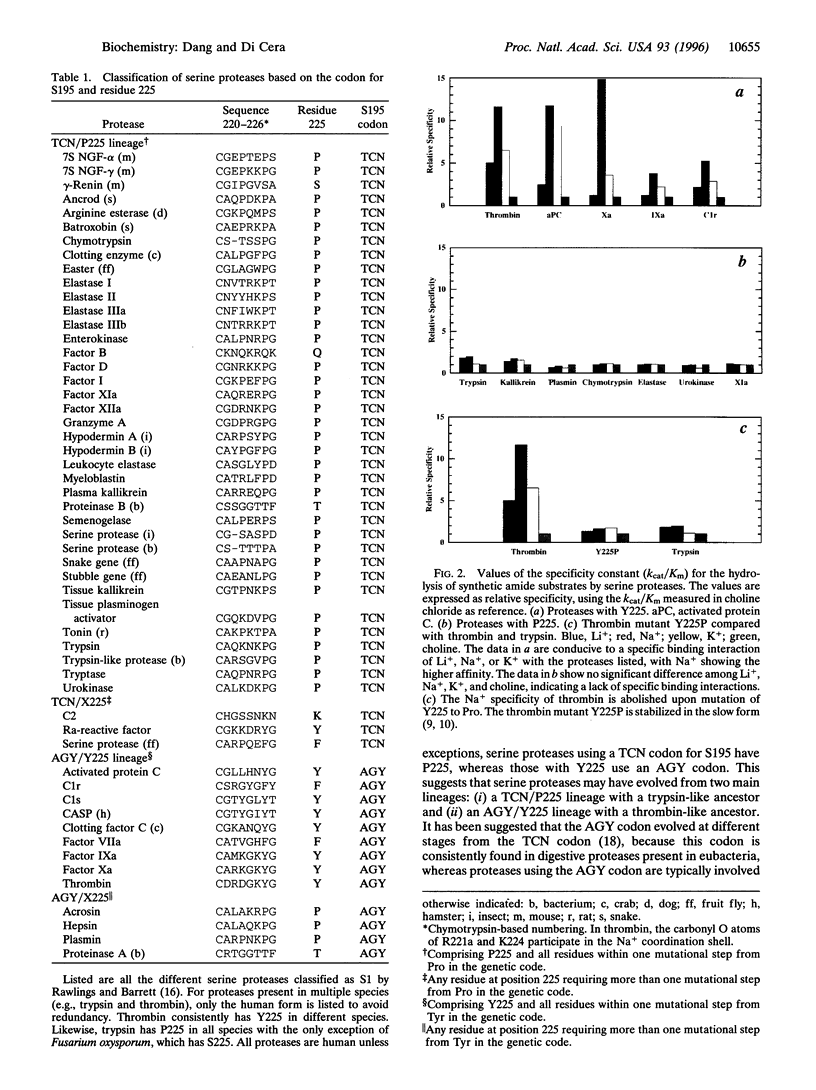
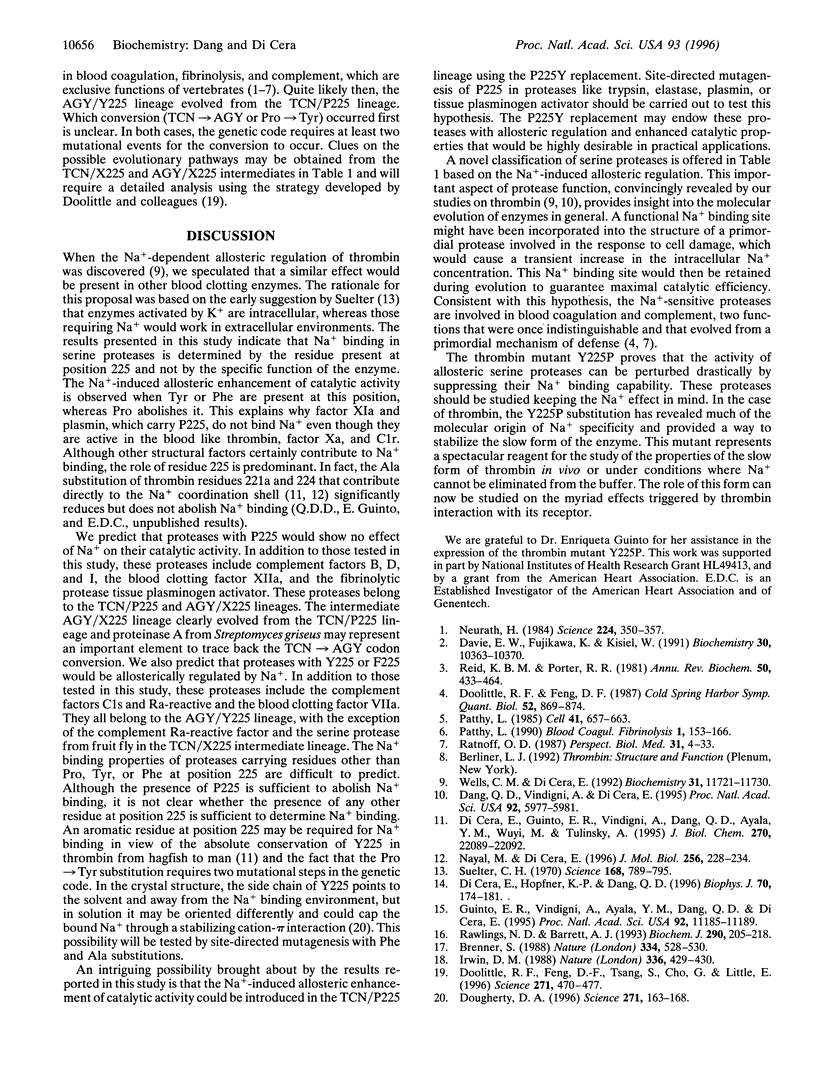
Images in this article
Selected References
These references are in PubMed. This may not be the complete list of references from this article.
- Brenner S. The molecular evolution of genes and proteins: a tale of two serines. Nature. 1988 Aug 11;334(6182):528–530. doi: 10.1038/334528a0. [DOI] [PubMed] [Google Scholar]
- Dang O. D., Vindigni A., Di Cera E. An allosteric switch controls the procoagulant and anticoagulant activities of thrombin. Proc Natl Acad Sci U S A. 1995 Jun 20;92(13):5977–5981. doi: 10.1073/pnas.92.13.5977. [DOI] [PMC free article] [PubMed] [Google Scholar]
- Davie E. W., Fujikawa K., Kisiel W. The coagulation cascade: initiation, maintenance, and regulation. Biochemistry. 1991 Oct 29;30(43):10363–10370. doi: 10.1021/bi00107a001. [DOI] [PubMed] [Google Scholar]
- Di Cera E., Guinto E. R., Vindigni A., Dang Q. D., Ayala Y. M., Wuyi M., Tulinsky A. The Na+ binding site of thrombin. J Biol Chem. 1995 Sep 22;270(38):22089–22092. doi: 10.1074/jbc.270.38.22089. [DOI] [PubMed] [Google Scholar]
- Di Cera E., Hopfner K. P., Dang Q. D. Theory of allosteric effects in serine proteases. Biophys J. 1996 Jan;70(1):174–181. doi: 10.1016/S0006-3495(96)79558-9. [DOI] [PMC free article] [PubMed] [Google Scholar]
- Doolittle R. F., Feng D. F. Reconstructing the evolution of vertebrate blood coagulation from a consideration of the amino acid sequences of clotting proteins. Cold Spring Harb Symp Quant Biol. 1987;52:869–874. doi: 10.1101/sqb.1987.052.01.095. [DOI] [PubMed] [Google Scholar]
- Doolittle R. F., Feng D. F., Tsang S., Cho G., Little E. Determining divergence times of the major kingdoms of living organisms with a protein clock. Science. 1996 Jan 26;271(5248):470–477. doi: 10.1126/science.271.5248.470. [DOI] [PubMed] [Google Scholar]
- Dougherty D. A. Cation-pi interactions in chemistry and biology: a new view of benzene, Phe, Tyr, and Trp. Science. 1996 Jan 12;271(5246):163–168. doi: 10.1126/science.271.5246.163. [DOI] [PubMed] [Google Scholar]
- Guinto E. R., Vindigni A., Ayala Y. M., Dang Q. D., Di Cera E. Identification of residues linked to the slow-->fast transition of thrombin. Proc Natl Acad Sci U S A. 1995 Nov 21;92(24):11185–11189. doi: 10.1073/pnas.92.24.11185. [DOI] [PMC free article] [PubMed] [Google Scholar]
- Irwin D. M. Evolution of an active-site codon in serine proteases. Nature. 1988 Dec 1;336(6198):429–430. doi: 10.1038/336429b0. [DOI] [PubMed] [Google Scholar]
- Nayal M., Di Cera E. Valence screening of water in protein crystals reveals potential Na+ binding sites. J Mol Biol. 1996 Feb 23;256(2):228–234. doi: 10.1006/jmbi.1996.0081. [DOI] [PubMed] [Google Scholar]
- Neurath H. Evolution of proteolytic enzymes. Science. 1984 Apr 27;224(4647):350–357. doi: 10.1126/science.6369538. [DOI] [PubMed] [Google Scholar]
- Patthy L. Evolution of blood coagulation and fibrinolysis. Blood Coagul Fibrinolysis. 1990 Jun;1(2):153–166. [PubMed] [Google Scholar]
- Patthy L. Evolution of the proteases of blood coagulation and fibrinolysis by assembly from modules. Cell. 1985 Jul;41(3):657–663. doi: 10.1016/s0092-8674(85)80046-5. [DOI] [PubMed] [Google Scholar]
- Ratnoff O. D. The evolution of hemostatic mechanisms. Perspect Biol Med. 1987 Autumn;31(1):4–33. doi: 10.1353/pbm.1987.0003. [DOI] [PubMed] [Google Scholar]
- Rawlings N. D., Barrett A. J. Evolutionary families of peptidases. Biochem J. 1993 Feb 15;290(Pt 1):205–218. doi: 10.1042/bj2900205. [DOI] [PMC free article] [PubMed] [Google Scholar]
- Reid K. B., Porter R. R. The proteolytic activation systems of complement. Annu Rev Biochem. 1981;50:433–464. doi: 10.1146/annurev.bi.50.070181.002245. [DOI] [PubMed] [Google Scholar]
- Suelter C. H. Enzymes activated by monovalent cations. Science. 1970 May 15;168(3933):789–795. doi: 10.1126/science.168.3933.789. [DOI] [PubMed] [Google Scholar]
- Wells C. M., Di Cera E. Thrombin is a Na(+)-activated enzyme. Biochemistry. 1992 Dec 1;31(47):11721–11730. doi: 10.1021/bi00162a008. [DOI] [PubMed] [Google Scholar]