Abstract
We develop a unifying theory of hypoxia tolerance based on information from two cell level models (brain cortical cells and isolated hepatocytes) from the highly anoxia tolerant aquatic turtle and from other more hypoxia sensitive systems. We propose that the response of hypoxia tolerant systems to oxygen lack occurs in two phases (defense and rescue). The first lines of defense against hypoxia include a balanced suppression of ATP-demand and ATP-supply pathways; this regulation stabilizes (adenylates) at new steady-state levels even while ATP turnover rates greatly decline. The ATP demands of ion pumping are down-regulated by generalized "channel" arrest in hepatocytes and by "spike" arrest in neurons. Hypoxic ATP demands of protein synthesis are down-regulated probably by translational arrest. In hypoxia sensitive cells this translational arrest seems irreversible, but hypoxia-tolerant systems activate "rescue" mechanisms if the period of oxygen lack is extended by preferentially regulating the expression of several proteins. In these cells, a cascade of processes underpinning hypoxia rescue and defense begins with an oxygen sensor (a heme protein) and a signal-transduction pathway, which leads to significant gene-based metabolic reprogramming-the rescue process-with maintained down-regulation of energy-demand and energy-supply pathways in metabolism throughout the hypoxic period. This recent work begins to clarify how normoxic maintenance ATP turnover rates can be drastically (10-fold) down-regulated to a new hypometabolic steady state, which is prerequisite for surviving prolonged hypoxia or anoxia. The implications of these developments are extensive in biology and medicine.
Full text
PDF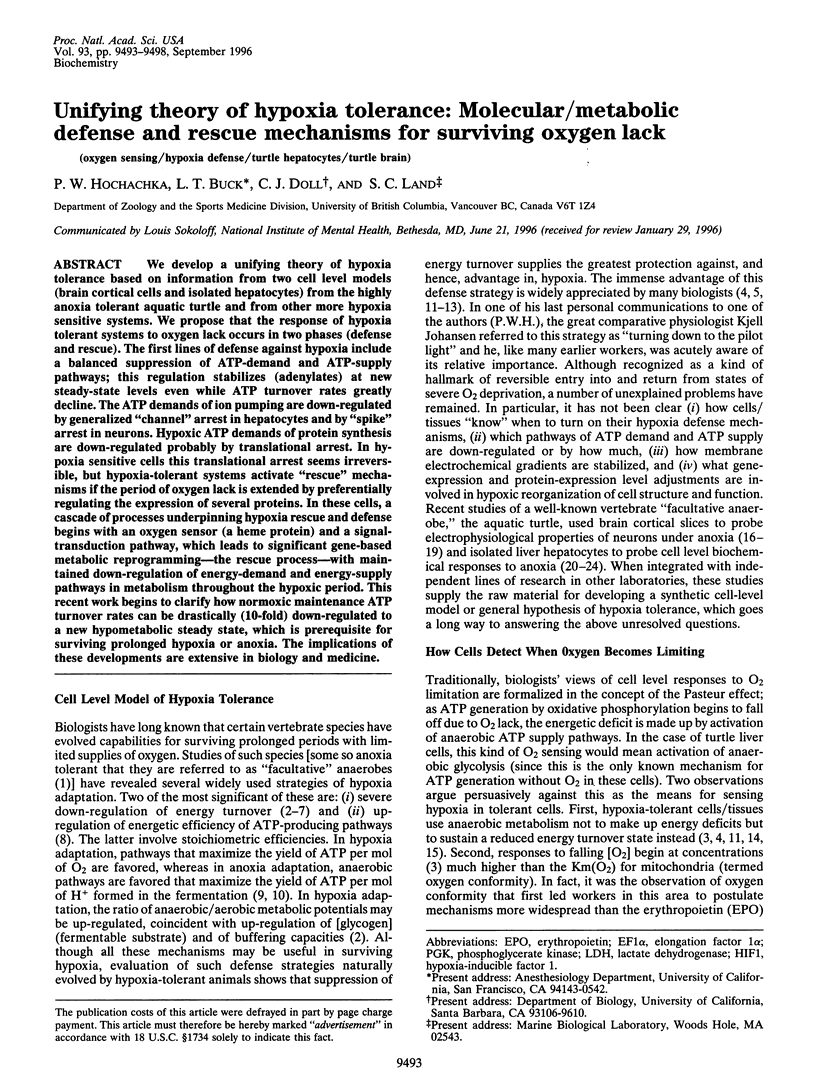
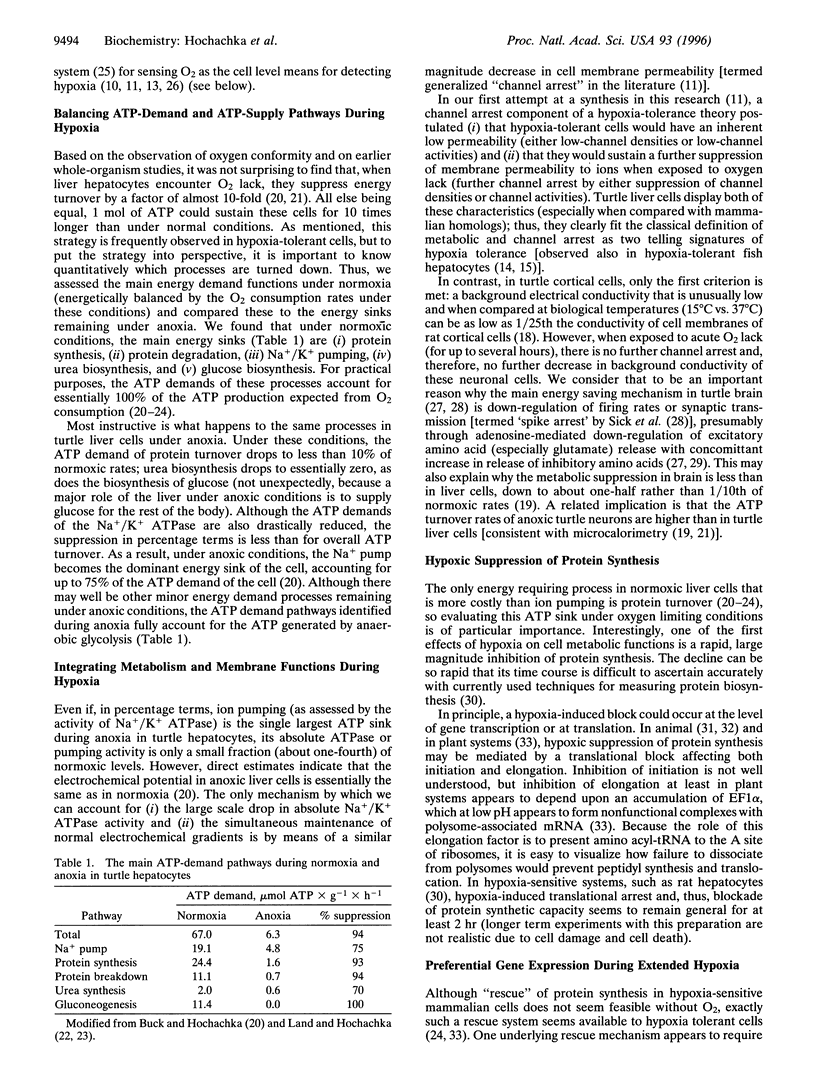
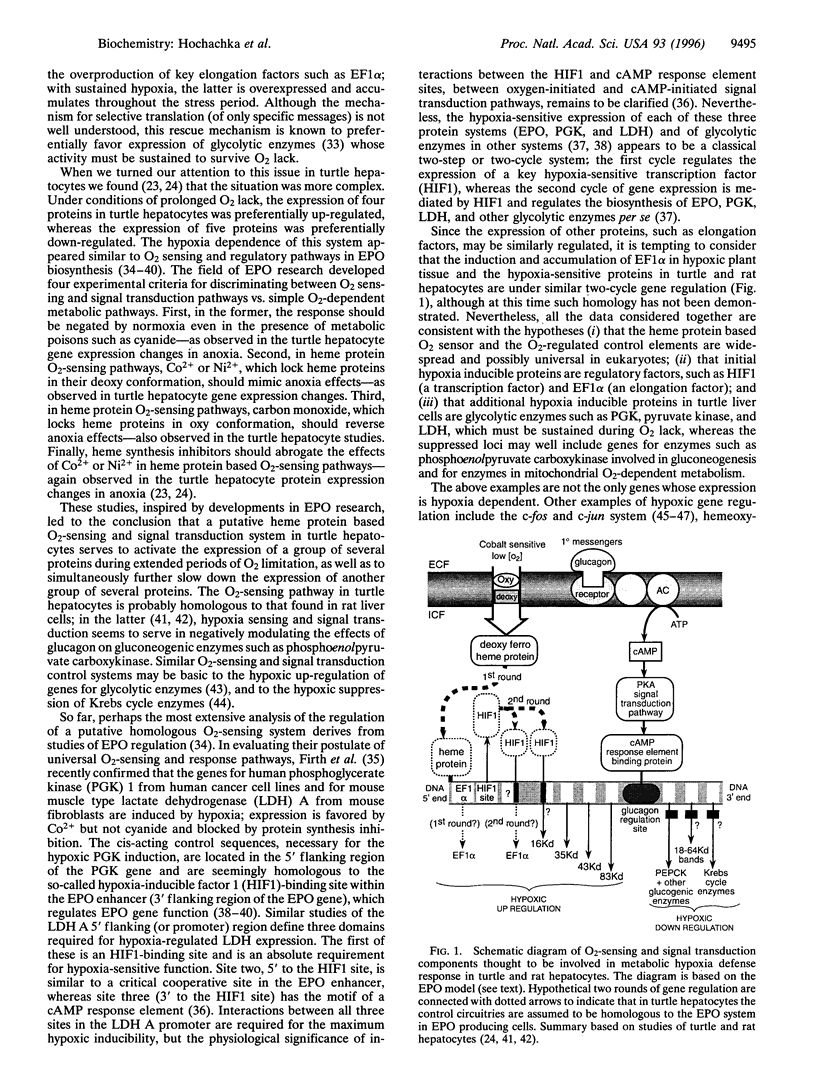
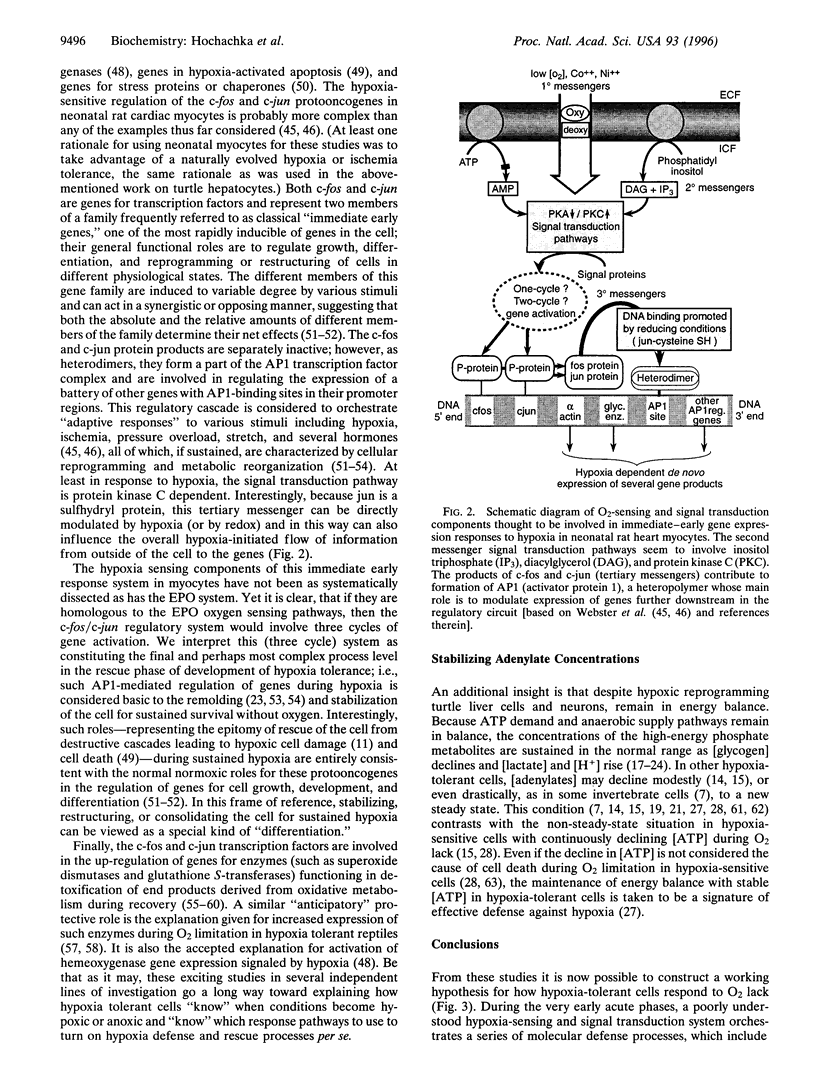
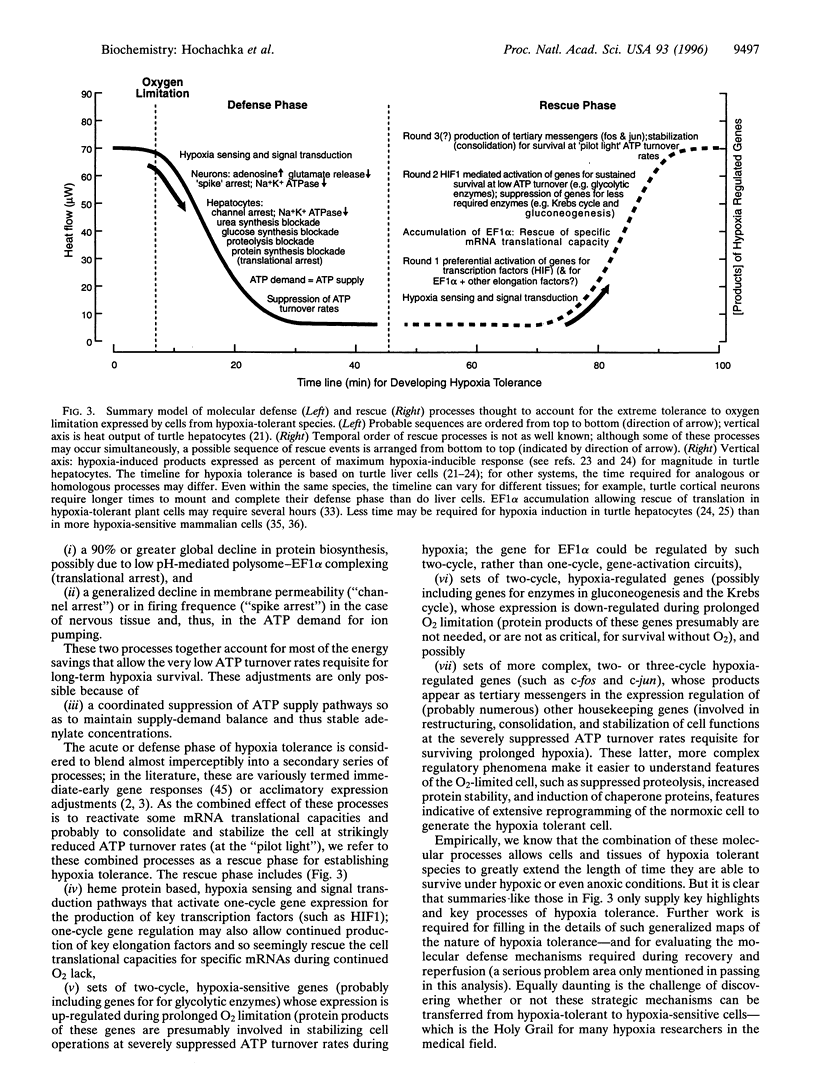
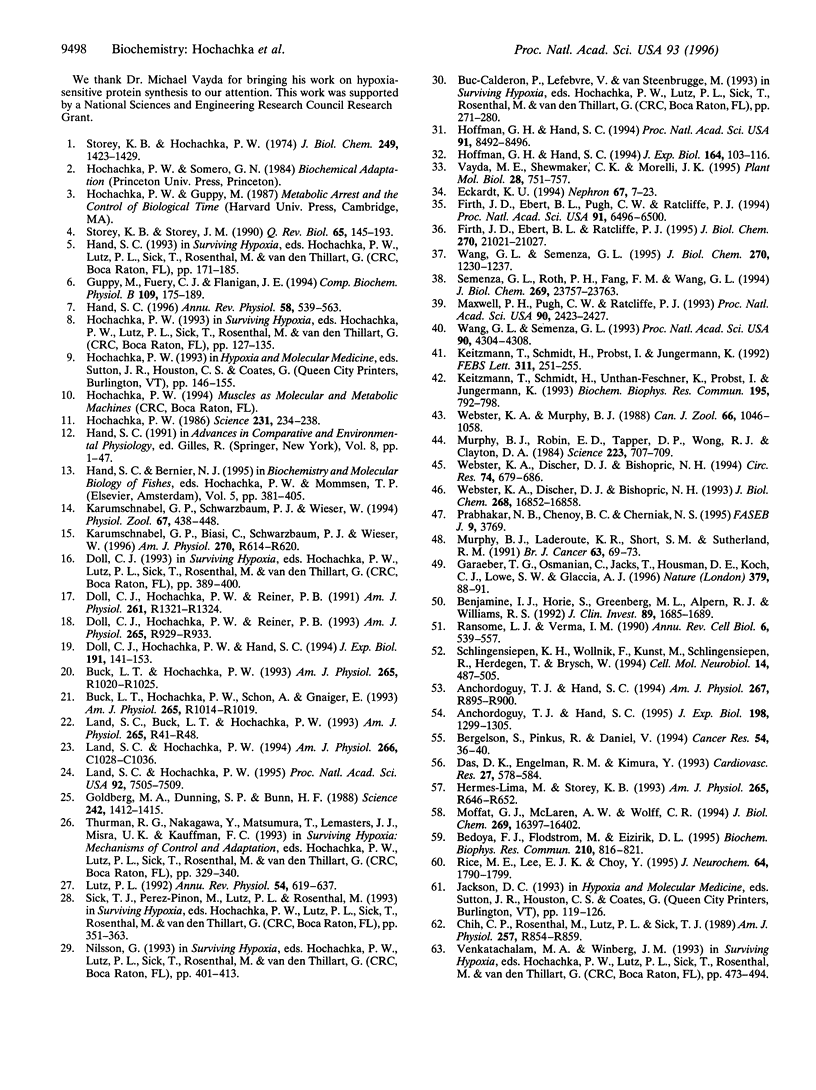
Images in this article
Selected References
These references are in PubMed. This may not be the complete list of references from this article.
- Anchordoguy T. J., Hand S. C. Acute blockage of the ubiquitin-mediated proteolytic pathway during invertebrate quiescence. Am J Physiol. 1994 Oct;267(4 Pt 2):R895–R900. doi: 10.1152/ajpregu.1994.267.4.R895. [DOI] [PubMed] [Google Scholar]
- Anchordoguy T, Hand S. Reactivation of ubiquitination in Artemia franciscana embryos during recovery from anoxia-induced quiescence. J Exp Biol. 1995;198(Pt 6):1299–1305. doi: 10.1242/jeb.198.6.1299. [DOI] [PubMed] [Google Scholar]
- Bedoya F. J., Flodström M., Eizirik D. L. Pyrrolidine dithiocarbamate prevents IL-1-induced nitric oxide synthase mRNA, but not superoxide dismutase mRNA, in insulin producing cells. Biochem Biophys Res Commun. 1995 May 25;210(3):816–822. doi: 10.1006/bbrc.1995.1731. [DOI] [PubMed] [Google Scholar]
- Benjamin I. J., Horie S., Greenberg M. L., Alpern R. J., Williams R. S. Induction of stress proteins in cultured myogenic cells. Molecular signals for the activation of heat shock transcription factor during ischemia. J Clin Invest. 1992 May;89(5):1685–1689. doi: 10.1172/JCI115768. [DOI] [PMC free article] [PubMed] [Google Scholar]
- Bergelson S., Pinkus R., Daniel V. Intracellular glutathione levels regulate Fos/Jun induction and activation of glutathione S-transferase gene expression. Cancer Res. 1994 Jan 1;54(1):36–40. [PubMed] [Google Scholar]
- Buck L. T., Hochachka P. W. Anoxic suppression of Na(+)-K(+)-ATPase and constant membrane potential in hepatocytes: support for channel arrest. Am J Physiol. 1993 Nov;265(5 Pt 2):R1020–R1025. doi: 10.1152/ajpregu.1993.265.5.R1020. [DOI] [PubMed] [Google Scholar]
- Buck L. T., Hochachka P. W., Schön A., Gnaiger E. Microcalorimetric measurement of reversible metabolic suppression induced by anoxia in isolated hepatocytes. Am J Physiol. 1993 Nov;265(5 Pt 2):R1014–R1019. doi: 10.1152/ajpregu.1993.265.5.R1014. [DOI] [PubMed] [Google Scholar]
- Chih C. P., Feng Z. C., Rosenthal M., Lutz P. L., Sick T. J. Energy metabolism, ion homeostasis, and evoked potentials in anoxic turtle brain. Am J Physiol. 1989 Oct;257(4 Pt 2):R854–R860. doi: 10.1152/ajpregu.1989.257.4.R854. [DOI] [PubMed] [Google Scholar]
- Das D. K., Engelman R. M., Kimura Y. Molecular adaptation of cellular defences following preconditioning of the heart by repeated ischaemia. Cardiovasc Res. 1993 Apr;27(4):578–584. doi: 10.1093/cvr/27.4.578. [DOI] [PubMed] [Google Scholar]
- Doll C. J., Hochachka P. W., Reiner P. B. Channel arrest: implications from membrane resistance in turtle neurons. Am J Physiol. 1991 Nov;261(5 Pt 2):R1321–R1324. doi: 10.1152/ajpregu.1991.261.5.R1321. [DOI] [PubMed] [Google Scholar]
- Doll C. J., Hochachka P. W., Reiner P. B. Reduced ionic conductance in turtle brain. Am J Physiol. 1993 Oct;265(4 Pt 2):R929–R933. doi: 10.1152/ajpregu.1993.265.4.R929. [DOI] [PubMed] [Google Scholar]
- Doll C, Hochachka P, Hand S. A MICROCALORIMETRIC STUDY OF TURTLE CORTICAL SLICES: INSIGHTS INTO BRAIN METABOLIC DEPRESSION. J Exp Biol. 1994 Jun;191(1):141–153. doi: 10.1242/jeb.191.1.141. [DOI] [PubMed] [Google Scholar]
- Eckardt K. U. Erythropoietin: oxygen-dependent control of erythropoiesis and its failure in renal disease. Nephron. 1994;67(1):7–23. doi: 10.1159/000187882. [DOI] [PubMed] [Google Scholar]
- Firth J. D., Ebert B. L., Pugh C. W., Ratcliffe P. J. Oxygen-regulated control elements in the phosphoglycerate kinase 1 and lactate dehydrogenase A genes: similarities with the erythropoietin 3' enhancer. Proc Natl Acad Sci U S A. 1994 Jul 5;91(14):6496–6500. doi: 10.1073/pnas.91.14.6496. [DOI] [PMC free article] [PubMed] [Google Scholar]
- Firth J. D., Ebert B. L., Ratcliffe P. J. Hypoxic regulation of lactate dehydrogenase A. Interaction between hypoxia-inducible factor 1 and cAMP response elements. J Biol Chem. 1995 Sep 8;270(36):21021–21027. doi: 10.1074/jbc.270.36.21021. [DOI] [PubMed] [Google Scholar]
- Goldberg M. A., Dunning S. P., Bunn H. F. Regulation of the erythropoietin gene: evidence that the oxygen sensor is a heme protein. Science. 1988 Dec 9;242(4884):1412–1415. doi: 10.1126/science.2849206. [DOI] [PubMed] [Google Scholar]
- Graeber T. G., Osmanian C., Jacks T., Housman D. E., Koch C. J., Lowe S. W., Giaccia A. J. Hypoxia-mediated selection of cells with diminished apoptotic potential in solid tumours. Nature. 1996 Jan 4;379(6560):88–91. doi: 10.1038/379088a0. [DOI] [PubMed] [Google Scholar]
- Guppy M., Fuery C. J., Flanigan J. E. Biochemical principles of metabolic depression. Comp Biochem Physiol B Biochem Mol Biol. 1994 Oct-Nov;109(2-3):175–189. doi: 10.1016/0305-0491(94)90001-9. [DOI] [PubMed] [Google Scholar]
- Hand S. C., Hardewig I. Downregulation of cellular metabolism during environmental stress: mechanisms and implications. Annu Rev Physiol. 1996;58:539–563. doi: 10.1146/annurev.ph.58.030196.002543. [DOI] [PubMed] [Google Scholar]
- Hermes-Lima M., Storey K. B. Antioxidant defenses in the tolerance of freezing and anoxia by garter snakes. Am J Physiol. 1993 Sep;265(3 Pt 2):R646–R652. doi: 10.1152/ajpregu.1993.265.3.R646. [DOI] [PubMed] [Google Scholar]
- Hochachka P. W. Defense strategies against hypoxia and hypothermia. Science. 1986 Jan 17;231(4735):234–241. doi: 10.1126/science.2417316. [DOI] [PubMed] [Google Scholar]
- Hofmann G. E., Hand S. C. Comparison of messenger RNA pools in active and dormant Artemia franciscana embryos: evidence for translational control. J Exp Biol. 1992 Mar;164:103–116. doi: 10.1242/jeb.164.1.103. [DOI] [PubMed] [Google Scholar]
- Hofmann G. E., Hand S. C. Global arrest of translation during invertebrate quiescence. Proc Natl Acad Sci U S A. 1994 Aug 30;91(18):8492–8496. doi: 10.1073/pnas.91.18.8492. [DOI] [PMC free article] [PubMed] [Google Scholar]
- Kietzmann T., Schmidt H., Probst I., Jungermann K. Modulation of the glucagon-dependent activation of the phosphoenolpyruvate carboxykinase gene by oxygen in rat hepatocyte cultures. Evidence for a heme protein as oxygen sensor. FEBS Lett. 1992 Oct 26;311(3):251–255. doi: 10.1016/0014-5793(92)81113-z. [DOI] [PubMed] [Google Scholar]
- Kietzmann T., Schmidt H., Unthan-Fechner K., Probst I., Jungermann K. A ferro-heme protein senses oxygen levels, which modulate the glucagon-dependent activation of the phosphoenolpyruvate carboxykinase gene in rat hepatocyte cultures. Biochem Biophys Res Commun. 1993 Sep 15;195(2):792–798. doi: 10.1006/bbrc.1993.2115. [DOI] [PubMed] [Google Scholar]
- Krumschnabel G., Biasi C., Schwarzbaum P. J., Wieser W. Membrane-metabolic coupling and ion homeostasis in anoxia-tolerant and anoxia-intolerant hepatocytes. Am J Physiol. 1996 Mar;270(3 Pt 2):R614–R620. doi: 10.1152/ajpregu.1996.270.3.R614. [DOI] [PubMed] [Google Scholar]
- Land S. C., Buck L. T., Hochachka P. W. Response of protein synthesis to anoxia and recovery in anoxia-tolerant hepatocytes. Am J Physiol. 1993 Jul;265(1 Pt 2):R41–R48. doi: 10.1152/ajpregu.1993.265.1.R41. [DOI] [PubMed] [Google Scholar]
- Land S. C., Hochachka P. W. A heme-protein-based oxygen-sensing mechanism controls the expression and suppression of multiple proteins in anoxia-tolerant turtle hepatocytes. Proc Natl Acad Sci U S A. 1995 Aug 1;92(16):7505–7509. doi: 10.1073/pnas.92.16.7505. [DOI] [PMC free article] [PubMed] [Google Scholar]
- Land S. C., Hochachka P. W. Protein turnover during metabolic arrest in turtle hepatocytes: role and energy dependence of proteolysis. Am J Physiol. 1994 Apr;266(4 Pt 1):C1028–C1036. doi: 10.1152/ajpcell.1994.266.4.C1028. [DOI] [PubMed] [Google Scholar]
- Maxwell P. H., Pugh C. W., Ratcliffe P. J. Inducible operation of the erythropoietin 3' enhancer in multiple cell lines: evidence for a widespread oxygen-sensing mechanism. Proc Natl Acad Sci U S A. 1993 Mar 15;90(6):2423–2427. doi: 10.1073/pnas.90.6.2423. [DOI] [PMC free article] [PubMed] [Google Scholar]
- Moffat G. J., McLaren A. W., Wolf C. R. Involvement of Jun and Fos proteins in regulating transcriptional activation of the human pi class glutathione S-transferase gene in multidrug-resistant MCF7 breast cancer cells. J Biol Chem. 1994 Jun 10;269(23):16397–16402. [PubMed] [Google Scholar]
- Murphy B. J., Laderoute K. R., Short S. M., Sutherland R. M. The identification of heme oxygenase as a major hypoxic stress protein in Chinese hamster ovary cells. Br J Cancer. 1991 Jul;64(1):69–73. doi: 10.1038/bjc.1991.241. [DOI] [PMC free article] [PubMed] [Google Scholar]
- Murphy B. J., Robin E. D., Tapper D. P., Wong R. J., Clayton D. A. Hypoxic coordinate regulation of mitochondrial enzymes in mammalian cells. Science. 1984 Feb 17;223(4637):707–709. doi: 10.1126/science.6320368. [DOI] [PubMed] [Google Scholar]
- Ransone L. J., Verma I. M. Nuclear proto-oncogenes fos and jun. Annu Rev Cell Biol. 1990;6:539–557. doi: 10.1146/annurev.cb.06.110190.002543. [DOI] [PubMed] [Google Scholar]
- Rice M. E., Lee E. J., Choy Y. High levels of ascorbic acid, not glutathione, in the CNS of anoxia-tolerant reptiles contrasted with levels in anoxia-intolerant species. J Neurochem. 1995 Apr;64(4):1790–1799. doi: 10.1046/j.1471-4159.1995.64041790.x. [DOI] [PubMed] [Google Scholar]
- Schlingensiepen K. H., Wollnik F., Kunst M., Schlingensiepen R., Herdegen T., Brysch W. The role of Jun transcription factor expression and phosphorylation in neuronal differentiation, neuronal cell death, and plastic adaptations in vivo. Cell Mol Neurobiol. 1994 Oct;14(5):487–505. doi: 10.1007/BF02088833. [DOI] [PMC free article] [PubMed] [Google Scholar]
- Semenza G. L., Roth P. H., Fang H. M., Wang G. L. Transcriptional regulation of genes encoding glycolytic enzymes by hypoxia-inducible factor 1. J Biol Chem. 1994 Sep 23;269(38):23757–23763. [PubMed] [Google Scholar]
- Storey K. B., Hochachka P. W. Enzymes of energy metabolism in a vertebrate facultative anaerobe, Pseudemys scripta. Turtle heart pyruvate kinase. J Biol Chem. 1974 Mar 10;249(5):1423–1427. [PubMed] [Google Scholar]
- Storey K. B., Storey J. M. Metabolic rate depression and biochemical adaptation in anaerobiosis, hibernation and estivation. Q Rev Biol. 1990 Jun;65(2):145–174. doi: 10.1086/416717. [DOI] [PubMed] [Google Scholar]
- Storey K. B., Storey J. M. Natural freeze tolerance in ectothermic vertebrates. Annu Rev Physiol. 1992;54:619–637. doi: 10.1146/annurev.ph.54.030192.003155. [DOI] [PubMed] [Google Scholar]
- Vayda M. E., Shewmaker C. K., Morelli J. K. Translational arrest in hypoxic potato tubers is correlated with the aberrant association of elongation factor EF-1 alpha with polysomes. Plant Mol Biol. 1995 Jul;28(4):751–757. doi: 10.1007/BF00021198. [DOI] [PubMed] [Google Scholar]
- Wang G. L., Semenza G. L. General involvement of hypoxia-inducible factor 1 in transcriptional response to hypoxia. Proc Natl Acad Sci U S A. 1993 May 1;90(9):4304–4308. doi: 10.1073/pnas.90.9.4304. [DOI] [PMC free article] [PubMed] [Google Scholar]
- Wang G. L., Semenza G. L. Purification and characterization of hypoxia-inducible factor 1. J Biol Chem. 1995 Jan 20;270(3):1230–1237. doi: 10.1074/jbc.270.3.1230. [DOI] [PubMed] [Google Scholar]
- Webster K. A., Discher D. J., Bishopric N. H. Induction and nuclear accumulation of fos and jun proto-oncogenes in hypoxic cardiac myocytes. J Biol Chem. 1993 Aug 5;268(22):16852–16858. [PubMed] [Google Scholar]
- Webster K. A., Discher D. J., Bishopric N. H. Regulation of fos and jun immediate-early genes by redox or metabolic stress in cardiac myocytes. Circ Res. 1994 Apr;74(4):679–686. doi: 10.1161/01.res.74.4.679. [DOI] [PubMed] [Google Scholar]