Abstract
The interactions between myelinated axons and surrounding glia cells, in rat optic nerve, were investigated by optical recording with voltage-sensitive dyes. Electrical stimulation of the nerve evoked an optical signal revealing two clearly distinct components: a fast propagating component, corresponding to the compound action potential, and a prominent slow component. Several lines of evidence suggest that part of the slow component originated from depolarization of the oligodendrocytes by potassium accumulation in the paranodal or internodal region. In addition, the experiments suggest that in this preparation axons also have voltage-dependent Ca2+ channels, and a Ca2+-dependent K+ conductance involved in the depolarization of oligodendrocytes. Thus, axons and oligodendrocytes communicate in an intimate, ionically-mediated fashion, and oligodendrocytes may play an important functional role beyond that of providing the myelin sheath.
Full text
PDF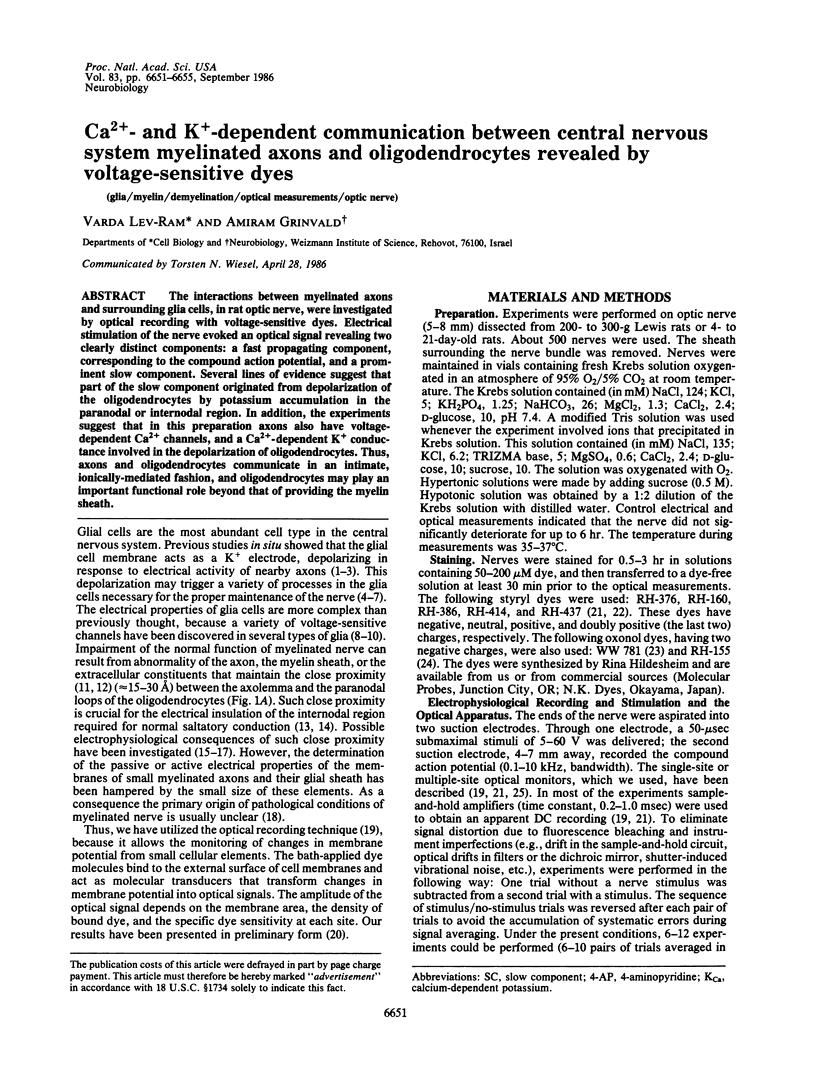
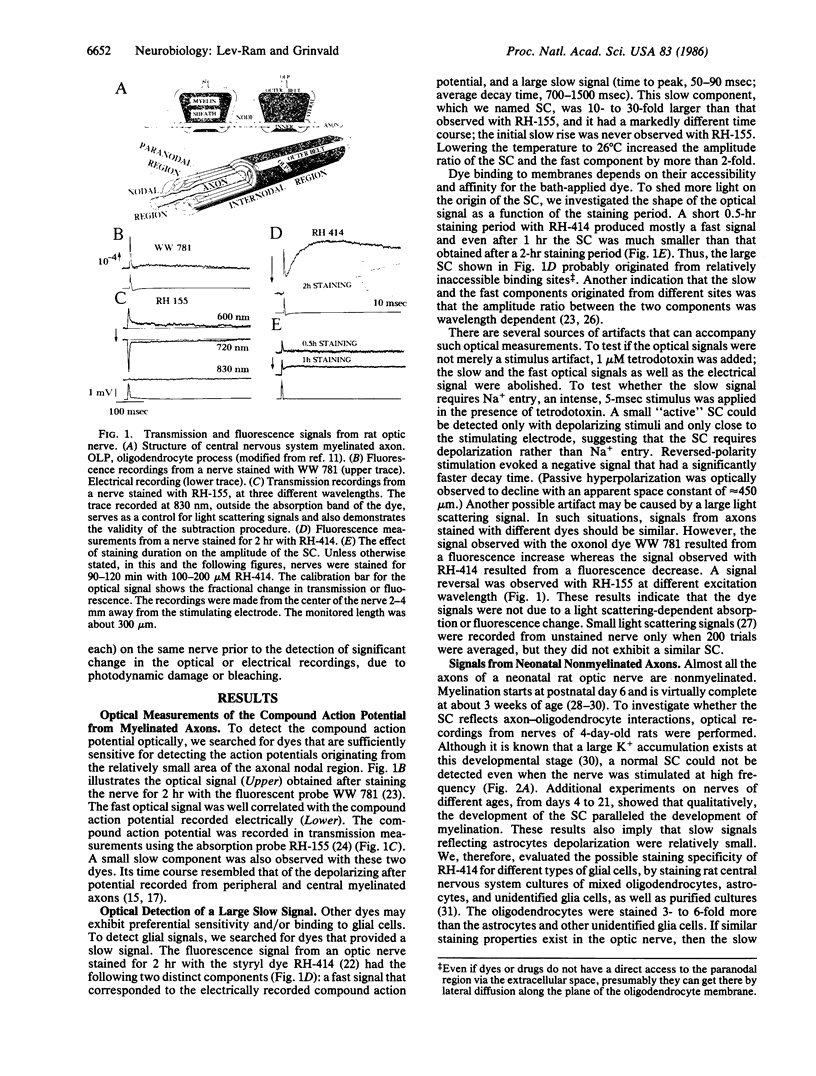
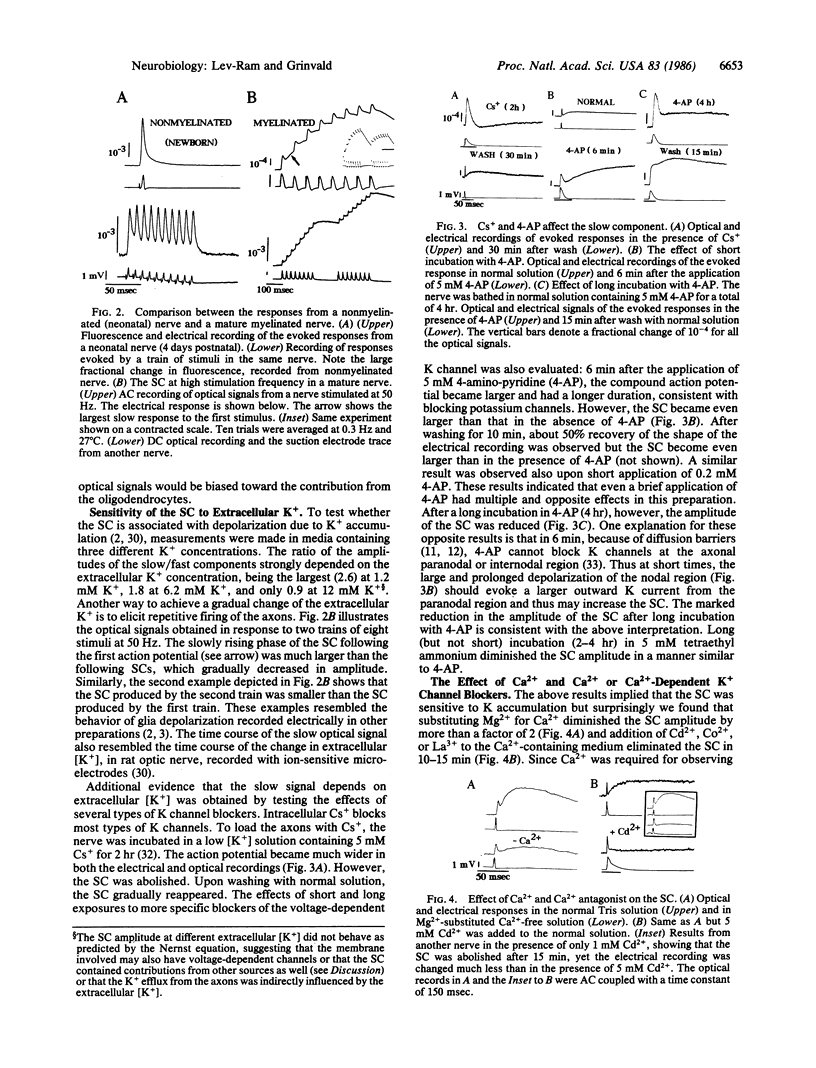
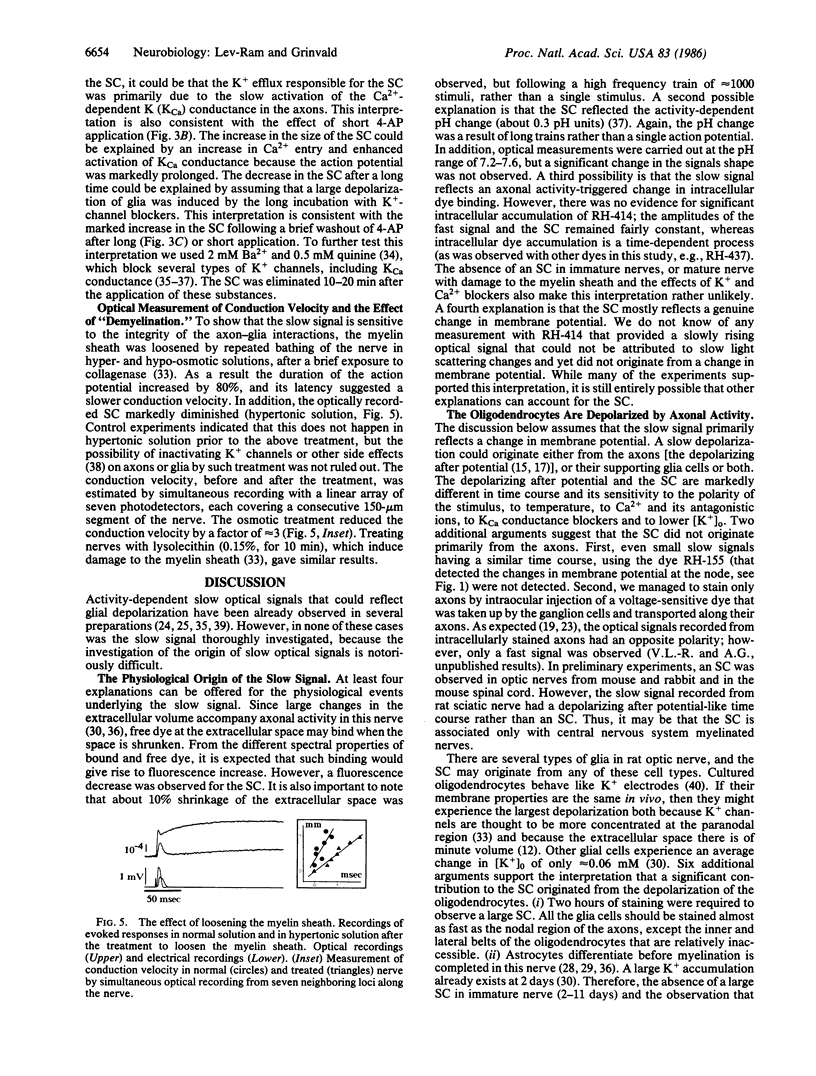
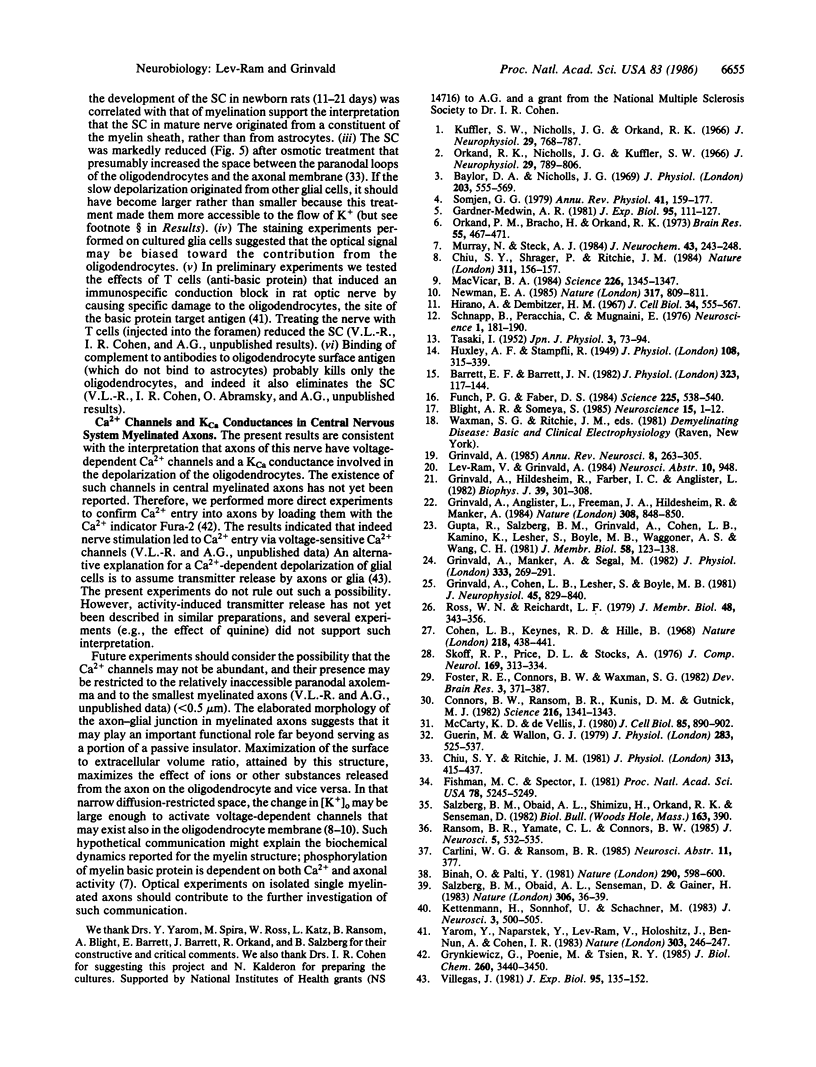
Images in this article
Selected References
These references are in PubMed. This may not be the complete list of references from this article.
- Barrett E. F., Barrett J. N. Intracellular recording from vertebrate myelinated axons: mechanism of the depolarizing afterpotential. J Physiol. 1982 Feb;323:117–144. doi: 10.1113/jphysiol.1982.sp014064. [DOI] [PMC free article] [PubMed] [Google Scholar]
- Baylor D. A., Nicholls J. G. Changes in extracellular potassium concentration produced by neuronal activity in the central nervous system of the leech. J Physiol. 1969 Aug;203(3):555–569. doi: 10.1113/jphysiol.1969.sp008879. [DOI] [PMC free article] [PubMed] [Google Scholar]
- Binah O., Palti Y. Potassium channels in the nodal membrane of rat myelinated fibres. Nature. 1981 Apr 16;290(5807):598–600. doi: 10.1038/290598a0. [DOI] [PubMed] [Google Scholar]
- Blight A. R., Someya S. Depolarizing afterpotentials in myelinated axons of mammalian spinal cord. Neuroscience. 1985 May;15(1):1–12. doi: 10.1016/0306-4522(85)90118-6. [DOI] [PubMed] [Google Scholar]
- Chiu S. Y., Ritchie J. M. Evidence for the presence of potassium channels in the paranodal region of acutely demyelinated mammalian single nerve fibres. J Physiol. 1981;313:415–437. doi: 10.1113/jphysiol.1981.sp013674. [DOI] [PMC free article] [PubMed] [Google Scholar]
- Chiu S. Y., Schrager P., Ritchie J. M. Neuronal-type Na+ and K+ channels in rabbit cultured Schwann cells. Nature. 1984 Sep 13;311(5982):156–157. doi: 10.1038/311156a0. [DOI] [PubMed] [Google Scholar]
- Cohen L. B., Keynes R. D., Hille B. Light scattering and birefringence changes during nerve activity. Nature. 1968 May 4;218(5140):438–441. doi: 10.1038/218438a0. [DOI] [PubMed] [Google Scholar]
- Connors B. W., Ransom B. R., Kunis D. M., Gutnick M. J. Activity-dependent K+ accumulation in the developing rat optic nerve. Science. 1982 Jun 18;216(4552):1341–1343. doi: 10.1126/science.7079771. [DOI] [PubMed] [Google Scholar]
- Fishman M. C., Spector I. Potassium current suppression by quinidine reveals additional calcium currents in neuroblastoma cells. Proc Natl Acad Sci U S A. 1981 Aug;78(8):5245–5249. doi: 10.1073/pnas.78.8.5245. [DOI] [PMC free article] [PubMed] [Google Scholar]
- Foster R. E., Connors B. W., Waxman S. G. Rat optic nerve: electrophysiological, pharmacological and anatomical studies during development. Brain Res. 1982 Mar;255(3):371–386. doi: 10.1016/0165-3806(82)90005-0. [DOI] [PubMed] [Google Scholar]
- Funch P. G., Faber D. S. Measurement of myelin sheath resistances: implications for axonal conduction and pathophysiology. Science. 1984 Aug 3;225(4661):538–540. doi: 10.1126/science.6204382. [DOI] [PubMed] [Google Scholar]
- Gardner-Medwin A. R. Possible roles of vertebrate neuroglia in potassium dynamics, spreading depression and migraine. J Exp Biol. 1981 Dec;95:111–127. doi: 10.1242/jeb.95.1.111. [DOI] [PubMed] [Google Scholar]
- Grinvald A., Anglister L., Freeman J. A., Hildesheim R., Manker A. Real-time optical imaging of naturally evoked electrical activity in intact frog brain. 1984 Apr 26-May 2Nature. 308(5962):848–850. doi: 10.1038/308848a0. [DOI] [PubMed] [Google Scholar]
- Grinvald A., Cohen L. B., Lesher S., Boyle M. B. Simultaneous optical monitoring of activity of many neurons in invertebrate ganglia using a 124-element photodiode array. J Neurophysiol. 1981 May;45(5):829–840. doi: 10.1152/jn.1981.45.5.829. [DOI] [PubMed] [Google Scholar]
- Grinvald A., Hildesheim R., Farber I. C., Anglister L. Improved fluorescent probes for the measurement of rapid changes in membrane potential. Biophys J. 1982 Sep;39(3):301–308. doi: 10.1016/S0006-3495(82)84520-7. [DOI] [PMC free article] [PubMed] [Google Scholar]
- Grinvald A., Manker A., Segal M. Visualization of the spread of electrical activity in rat hippocampal slices by voltage-sensitive optical probes. J Physiol. 1982 Dec;333:269–291. doi: 10.1113/jphysiol.1982.sp014453. [DOI] [PMC free article] [PubMed] [Google Scholar]
- Grinvald A. Real-time optical mapping of neuronal activity: from single growth cones to the intact mammalian brain. Annu Rev Neurosci. 1985;8:263–305. doi: 10.1146/annurev.ne.08.030185.001403. [DOI] [PubMed] [Google Scholar]
- Grynkiewicz G., Poenie M., Tsien R. Y. A new generation of Ca2+ indicators with greatly improved fluorescence properties. J Biol Chem. 1985 Mar 25;260(6):3440–3450. [PubMed] [Google Scholar]
- Guerin M., Wallon G. The reversible replacement of internal potassium by caesium in isolated turtle heart. J Physiol. 1979 Aug;293:525–537. doi: 10.1113/jphysiol.1979.sp012905. [DOI] [PMC free article] [PubMed] [Google Scholar]
- Gupta R. K., Salzberg B. M., Grinvald A., Cohen L. B., Kamino K., Lesher S., Boyle M. B., Waggoner A. S., Wang C. H. Improvements in optical methods for measuring rapid changes in membrane potential. J Membr Biol. 1981 Feb 15;58(2):123–137. doi: 10.1007/BF01870975. [DOI] [PubMed] [Google Scholar]
- Hirano A., Dembitzer H. M. A structural analysis of the myelin sheath in the central nervous system. J Cell Biol. 1967 Aug;34(2):555–567. doi: 10.1083/jcb.34.2.555. [DOI] [PMC free article] [PubMed] [Google Scholar]
- Huxley A. F., Stämpfli R. Evidence for saltatory conduction in peripheral myelinated nerve fibres. J Physiol. 1949 May 15;108(3):315–339. [PMC free article] [PubMed] [Google Scholar]
- Kettenmann H., Sonnhof U., Schachner M. Exclusive potassium dependence of the membrane potential in cultured mouse oligodendrocytes. J Neurosci. 1983 Mar;3(3):500–505. doi: 10.1523/JNEUROSCI.03-03-00500.1983. [DOI] [PMC free article] [PubMed] [Google Scholar]
- Kuffler S. W., Nicholls J. G., Orkand R. K. Physiological properties of glial cells in the central nervous system of amphibia. J Neurophysiol. 1966 Jul;29(4):768–787. doi: 10.1152/jn.1966.29.4.768. [DOI] [PubMed] [Google Scholar]
- MacVicar B. A. Voltage-dependent calcium channels in glial cells. Science. 1984 Dec 14;226(4680):1345–1347. doi: 10.1126/science.6095454. [DOI] [PubMed] [Google Scholar]
- McCarthy K. D., de Vellis J. Preparation of separate astroglial and oligodendroglial cell cultures from rat cerebral tissue. J Cell Biol. 1980 Jun;85(3):890–902. doi: 10.1083/jcb.85.3.890. [DOI] [PMC free article] [PubMed] [Google Scholar]
- Murray N., Steck A. J. Impulse conduction regulates myelin basic protein phosphorylation in rat optic nerve. J Neurochem. 1984 Jul;43(1):243–248. doi: 10.1111/j.1471-4159.1984.tb06702.x. [DOI] [PubMed] [Google Scholar]
- Newman E. A. Voltage-dependent calcium and potassium channels in retinal glial cells. 1985 Oct 31-Nov 6Nature. 317(6040):809–811. doi: 10.1038/317809a0. [DOI] [PMC free article] [PubMed] [Google Scholar]
- Orkand P. M., Bracho H., Orkand R. K. Glial metabolism: alteration by potassium levels comparable to those during neural activity. Brain Res. 1973 Jun 15;55(2):467–471. doi: 10.1016/0006-8993(73)90315-6. [DOI] [PubMed] [Google Scholar]
- Orkand R. K., Nicholls J. G., Kuffler S. W. Effect of nerve impulses on the membrane potential of glial cells in the central nervous system of amphibia. J Neurophysiol. 1966 Jul;29(4):788–806. doi: 10.1152/jn.1966.29.4.788. [DOI] [PubMed] [Google Scholar]
- Ransom B. R., Yamate C. L., Connors B. W. Activity-dependent shrinkage of extracellular space in rat optic nerve: a developmental study. J Neurosci. 1985 Feb;5(2):532–535. doi: 10.1523/JNEUROSCI.05-02-00532.1985. [DOI] [PMC free article] [PubMed] [Google Scholar]
- Ross W. N., Reichardt L. F. Species-specific effects on the optical signals of voltage-sensitive dyes. J Membr Biol. 1979 Aug;48(4):343–356. doi: 10.1007/BF01869445. [DOI] [PubMed] [Google Scholar]
- Salzberg B. M., Obaid A. L., Senseman D. M., Gainer H. Optical recording of action potentials from vertebrate nerve terminals using potentiometric probes provides evidence for sodium and calcium components. Nature. 1983 Nov 3;306(5938):36–40. doi: 10.1038/306036a0. [DOI] [PubMed] [Google Scholar]
- Schnapp B., Peracchia C., Mugnaini E. The paranodal axo-glial junction in the central nervous system studied with thin sections and freeze-fracture. Neuroscience. 1976 Jun;1(3):181–190. doi: 10.1016/0306-4522(76)90075-0. [DOI] [PubMed] [Google Scholar]
- Skoff R. P., Price D. L., Stocks A. Electron microscopic autoradiographic studies of gliogenesis in rat optic nerve. II. Time of origin. J Comp Neurol. 1976 Oct 1;169(3):313–334. doi: 10.1002/cne.901690304. [DOI] [PubMed] [Google Scholar]
- Somjen G. G. Extracellular potassium in the mammalian central nervous system. Annu Rev Physiol. 1979;41:159–177. doi: 10.1146/annurev.ph.41.030179.001111. [DOI] [PubMed] [Google Scholar]
- TASAKI I. Properties of myelinated fibers in frog sciatic nerve and in spinal cord as examined with micro-electrodes. Jpn J Physiol. 1952 Nov;3(1):73–94. doi: 10.2170/jjphysiol.3.73. [DOI] [PubMed] [Google Scholar]
- Villegas J. Axon/Schwann-cell relationships in the giant nerve fibre of the squid. J Exp Biol. 1981 Dec;95:135–151. doi: 10.1242/jeb.95.1.135. [DOI] [PubMed] [Google Scholar]
- Yarom Y., Naparstek Y., Lev-Ram V., Holoshitz J., Ben-Nun A., Cohen I. R. Immunospecific inhibition of nerve conduction by T lymphocytes reactive to basic protein of myelin. Nature. 1983 May 19;303(5914):246–247. doi: 10.1038/303246a0. [DOI] [PubMed] [Google Scholar]