Abstract
Covalent fluorescent probes were used to label the rabbit intestinal brush border Na+-glucose cotransporter at the putative glucose and Na+ binding sites, and a steady-state fluorescence energy transfer technique was used to measure the distance between the two binding sites. In both intact brush border membrane vesicles and partially purified soluble protein, the distance (R2/3) between the Na+ and glucose sites was approximately equal to 35 A. This distance was the same with four different donor/acceptor pairs with different transfer efficiencies, by donor quantum yield measurements, or sensitized acceptor fluorescence. The fact that the Na+ site and glucose site probes bind to a 75-kDa polypeptide, copurify with the same isoelectric point (pI 5.3) and retain function, and exhibit energy transfer indicates that the sites are on the same 75-kDa polypeptide. The large distance between the Na+ and glucose site probes raises questions about simple models of frictional interactions between the two substrates during the transport cycle.
Full text
PDF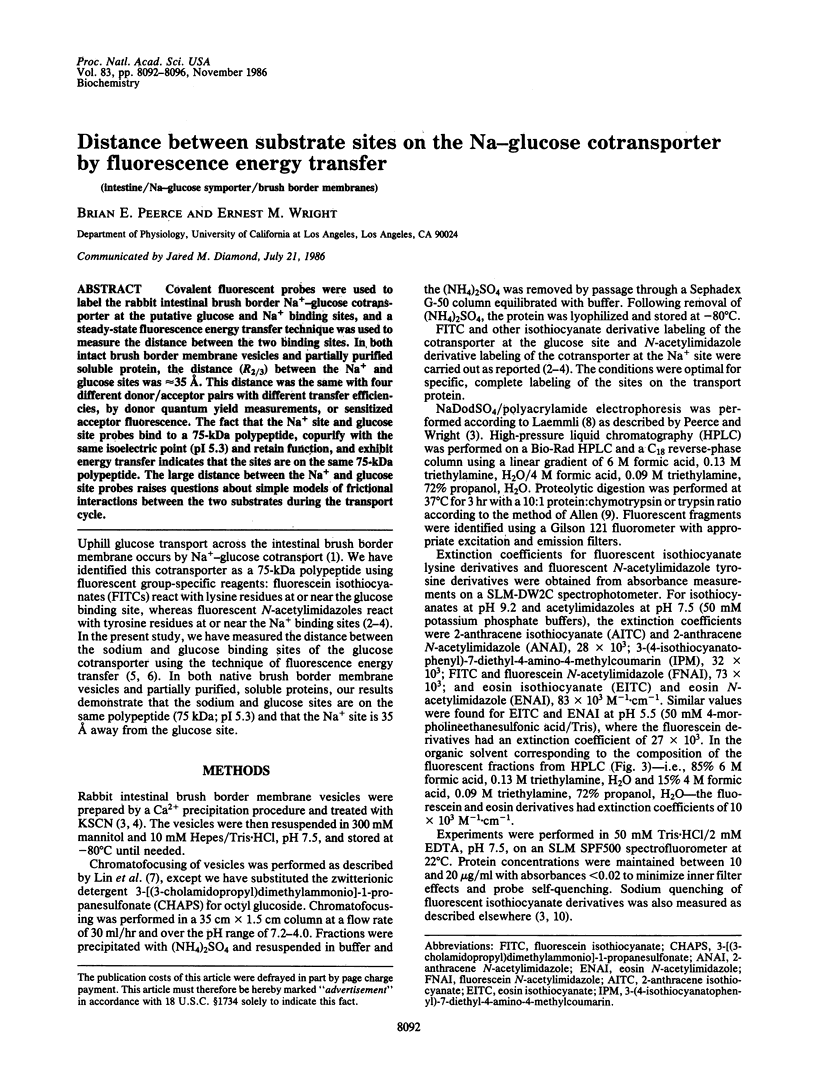
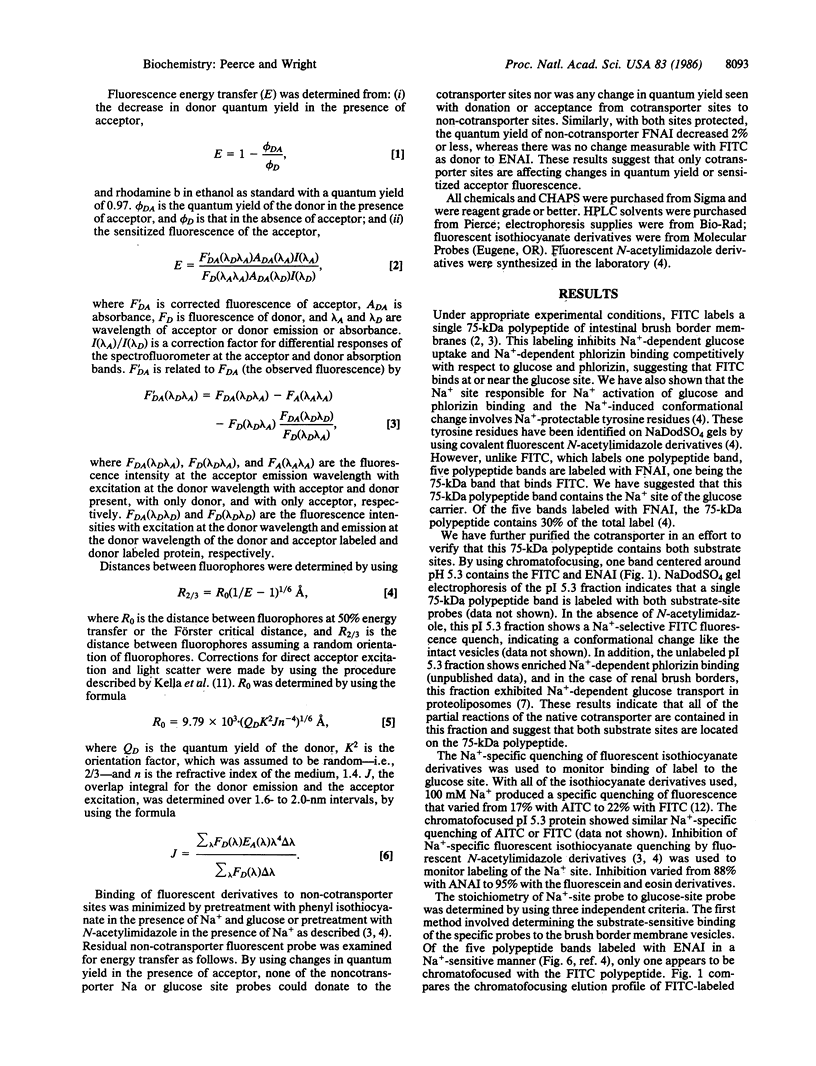
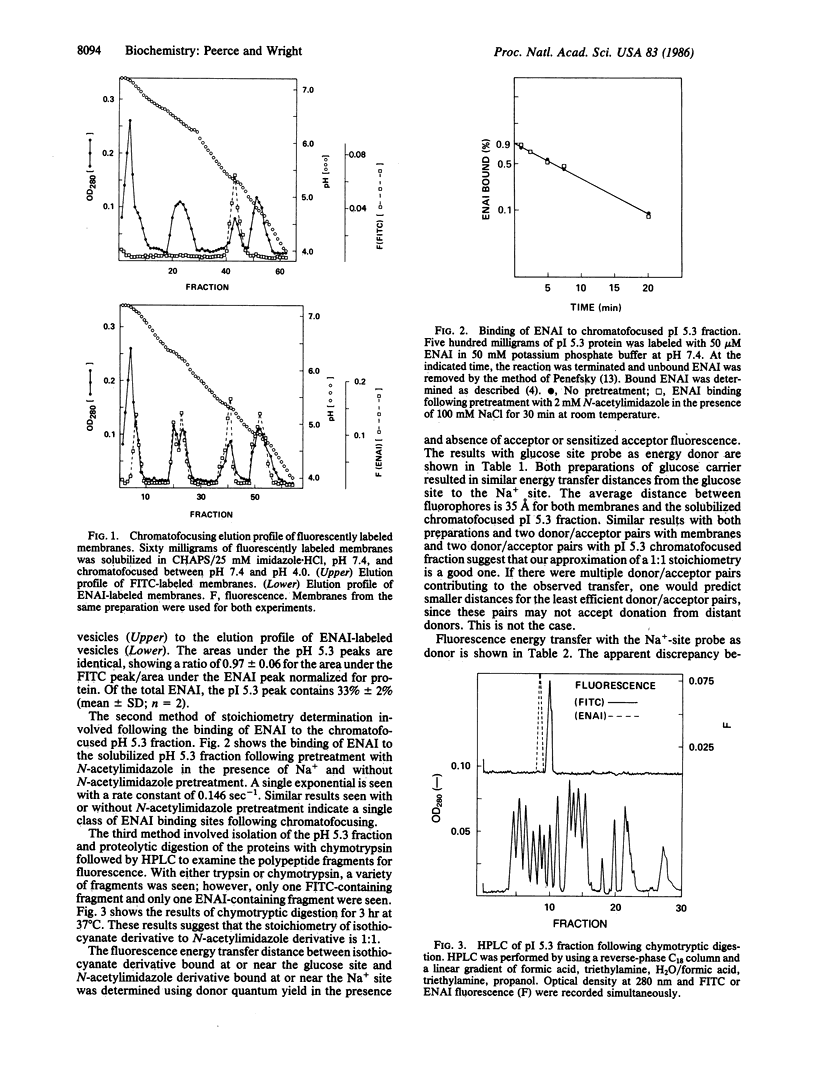
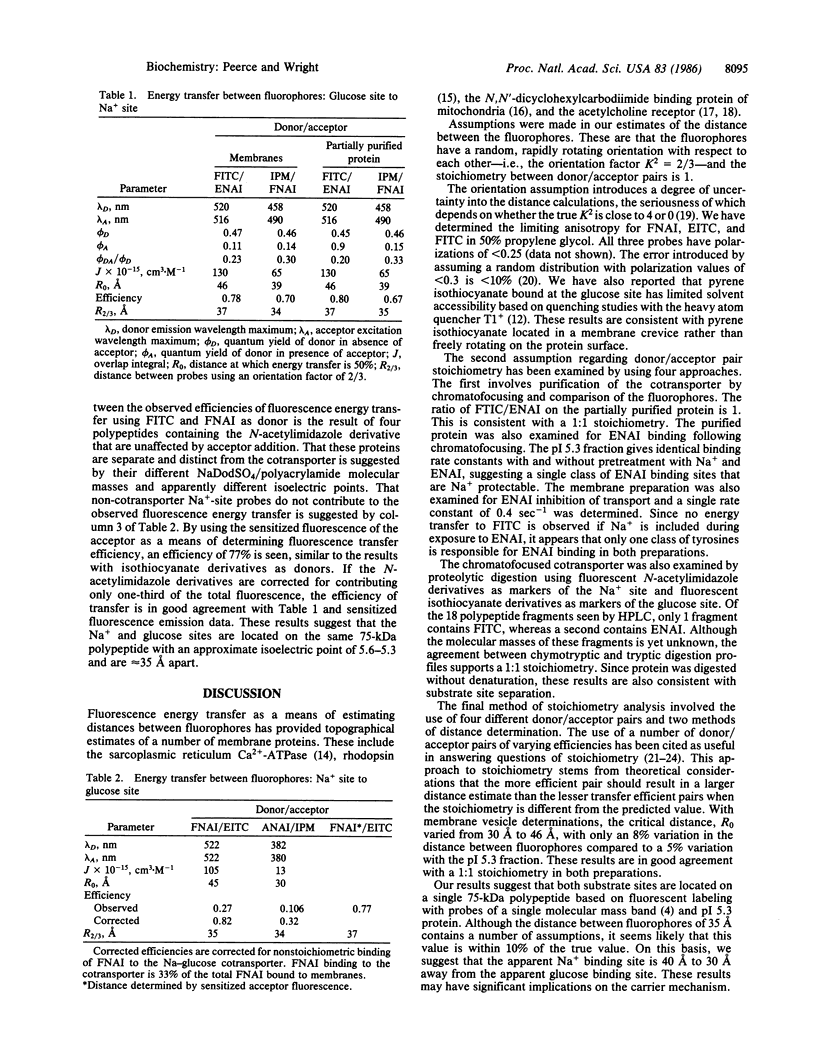
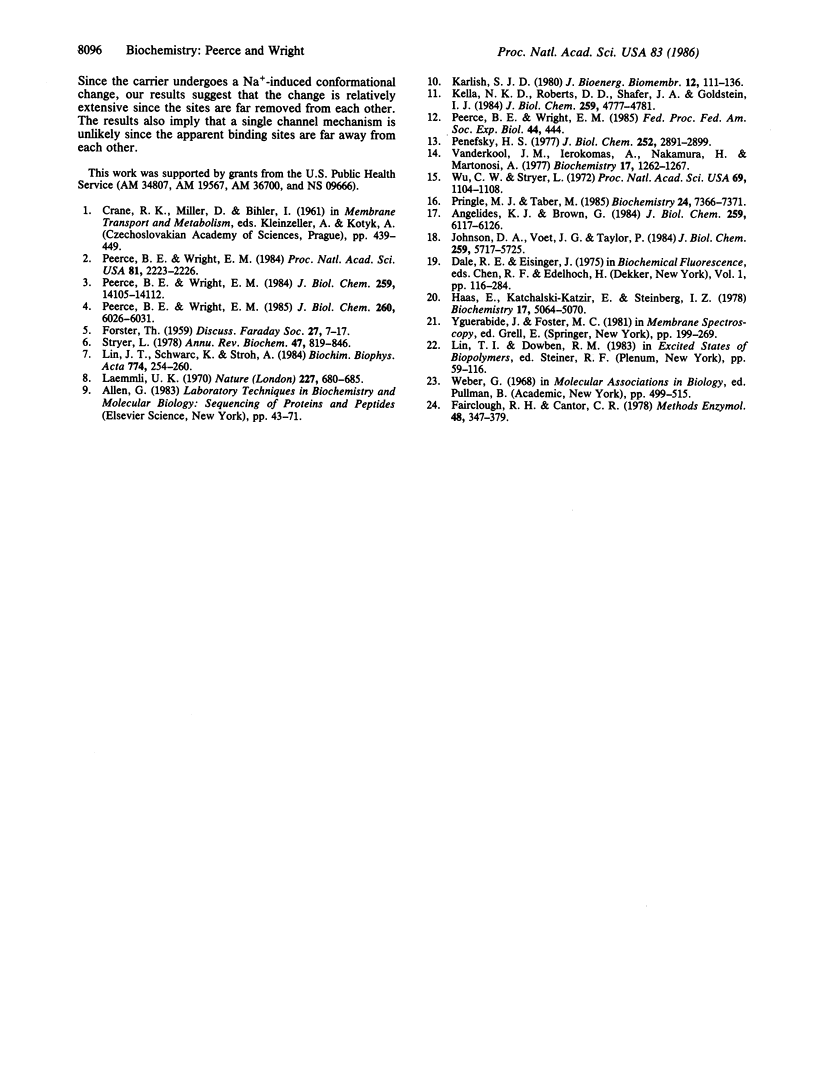
Selected References
These references are in PubMed. This may not be the complete list of references from this article.
- Angelides K. J., Brown G. B. Fluorescence resonance energy transfer on the voltage-dependent sodium channel. Spatial relationship and site coupling between the batrachotoxin and Leiurus quinquestriatus quinquestriatus alpha-scorpion toxin receptors. J Biol Chem. 1984 May 25;259(10):6117–6126. [PubMed] [Google Scholar]
- Fairclough R. H., Cantor C. R. The use of singlet-singlet energy transfer to study macromolecular assemblies. Methods Enzymol. 1978;48:347–379. doi: 10.1016/s0076-6879(78)48019-x. [DOI] [PubMed] [Google Scholar]
- Haas E., Katchalski-Katzir E., Steinberg I. Z. Effect of the orientation of donor and acceptor on the probability of energy transfer involving electronic transitions of mixed polarization. Biochemistry. 1978 Nov 14;17(23):5064–5070. doi: 10.1021/bi00616a032. [DOI] [PubMed] [Google Scholar]
- Johnson D. A., Voet J. G., Taylor P. Fluorescence energy transfer between cobra alpha-toxin molecules bound to the acetylcholine receptor. J Biol Chem. 1984 May 10;259(9):5717–5725. [PubMed] [Google Scholar]
- Karlish S. J. Characterization of conformational changes in (Na,K) ATPase labeled with fluorescein at the active site. J Bioenerg Biomembr. 1980 Aug;12(3-4):111–136. doi: 10.1007/BF00744678. [DOI] [PubMed] [Google Scholar]
- Kella N. K., Roberts D. D., Shafer J. A., Goldstein I. J. Fluorescence energy transfer studies on lima bean lectin. Distance between the subunit hydrophobic binding site and the thiol group essential for carbohydrate binding. J Biol Chem. 1984 Apr 25;259(8):4777–4781. [PubMed] [Google Scholar]
- Laemmli U. K. Cleavage of structural proteins during the assembly of the head of bacteriophage T4. Nature. 1970 Aug 15;227(5259):680–685. doi: 10.1038/227680a0. [DOI] [PubMed] [Google Scholar]
- Lin J. T., Schwarc K., Stroh A. Chromatofocussing and centrifugal reconstitution as tools for the separation and characterization of the Na+-cotransport systems of the brush-border membrane. Biochim Biophys Acta. 1984 Jul 25;774(2):254–260. doi: 10.1016/0005-2736(84)90299-2. [DOI] [PubMed] [Google Scholar]
- Peerce B. E., Wright E. M. Conformational changes in the intestinal brush border sodium-glucose cotransporter labeled with fluorescein isothiocyanate. Proc Natl Acad Sci U S A. 1984 Apr;81(7):2223–2226. doi: 10.1073/pnas.81.7.2223. [DOI] [PMC free article] [PubMed] [Google Scholar]
- Peerce B. E., Wright E. M. Evidence for tyrosyl residues at the Na+ site on the intestinal Na+/glucose cotransporter. J Biol Chem. 1985 May 25;260(10):6026–6031. [PubMed] [Google Scholar]
- Peerce B. E., Wright E. M. Sodium-induced conformational changes in the glucose transporter of intestinal brush borders. J Biol Chem. 1984 Nov 25;259(22):14105–14112. [PubMed] [Google Scholar]
- Penefsky H. S. Reversible binding of Pi by beef heart mitochondrial adenosine triphosphatase. J Biol Chem. 1977 May 10;252(9):2891–2899. [PubMed] [Google Scholar]
- Pringle M. J., Taber M. Fluorescent analogues of N,N'-dicyclohexylcarbodiimide as structural probes of the bovine mitochondrial proton channel. Biochemistry. 1985 Dec 3;24(25):7366–7371. doi: 10.1021/bi00346a051. [DOI] [PubMed] [Google Scholar]
- Stryer L. Fluorescence energy transfer as a spectroscopic ruler. Annu Rev Biochem. 1978;47:819–846. doi: 10.1146/annurev.bi.47.070178.004131. [DOI] [PubMed] [Google Scholar]
- Vanderkooi J. M., Ierokomas A., Nakamura H., Martonosi A. Fluorescence energy transfer between Ca2+ transport ATPase molecules in artificial membranes. Biochemistry. 1977 Apr 5;16(7):1262–1267. doi: 10.1021/bi00626a003. [DOI] [PubMed] [Google Scholar]
- Wu C. W., Stryer L. Proximity relationships in rhodopsin. Proc Natl Acad Sci U S A. 1972 May;69(5):1104–1108. doi: 10.1073/pnas.69.5.1104. [DOI] [PMC free article] [PubMed] [Google Scholar]
- Yguerabide J., Foster M. C. Fluorescence spectroscopy of biological membranes. Mol Biol Biochem Biophys. 1981;31:199–269. doi: 10.1007/978-3-642-81537-9_5. [DOI] [PubMed] [Google Scholar]