Abstract
It has been suggested that recombination and shuffling between exons has been a key feature in the evolution of proteins. We propose that this strategy could also be used for the artificial evolution of proteins in bacteria. As a first step, we illustrate the use of a self-splicing group I intron with inserted lox-Cre recombination site to assemble a very large combinatorial repertoire (> 10(11) members) of peptides from two different exons. Each exon comprised a repertoire of 10 random amino acids residues; after splicing, the repertoires were joined together through a central five-residue spacer to give a combinatorial repertoire of 25-residue peptides. The repertoire was displayed on filamentous bacteriophage by fusion to the pIII phage coat protein and selected by binding to several proteins, including beta-glucuronidase. One of the peptides selected against beta-glucuronidase was chemically synthesized and shown to inhibit the enzymatic activity (inhibition constant: 17 nM); by further exon shuffling, an improved inhibitor was isolated (inhibition constant: 7 nM). Not only does this approach provide the means for making very large peptide repertoires, but we anticipate that by introducing constraints in the sequences of the peptides and of the linker, it may be possible to evolve small folded peptides and proteins.
Full text
PDF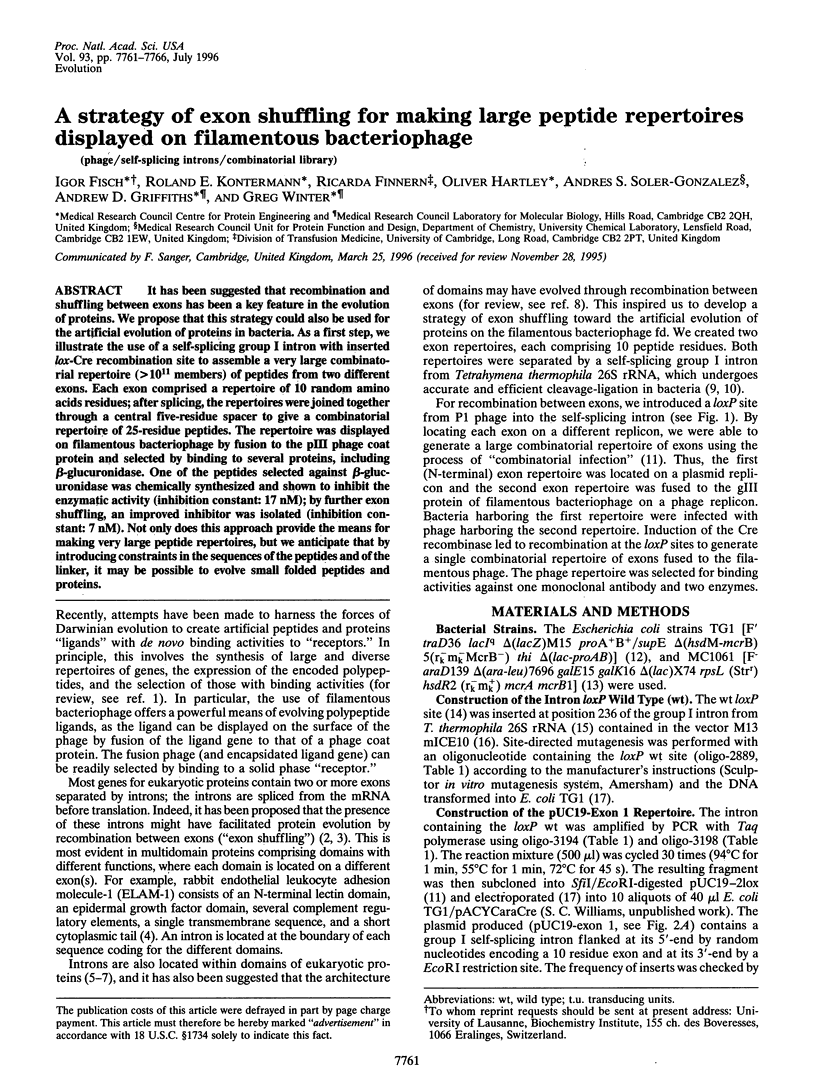
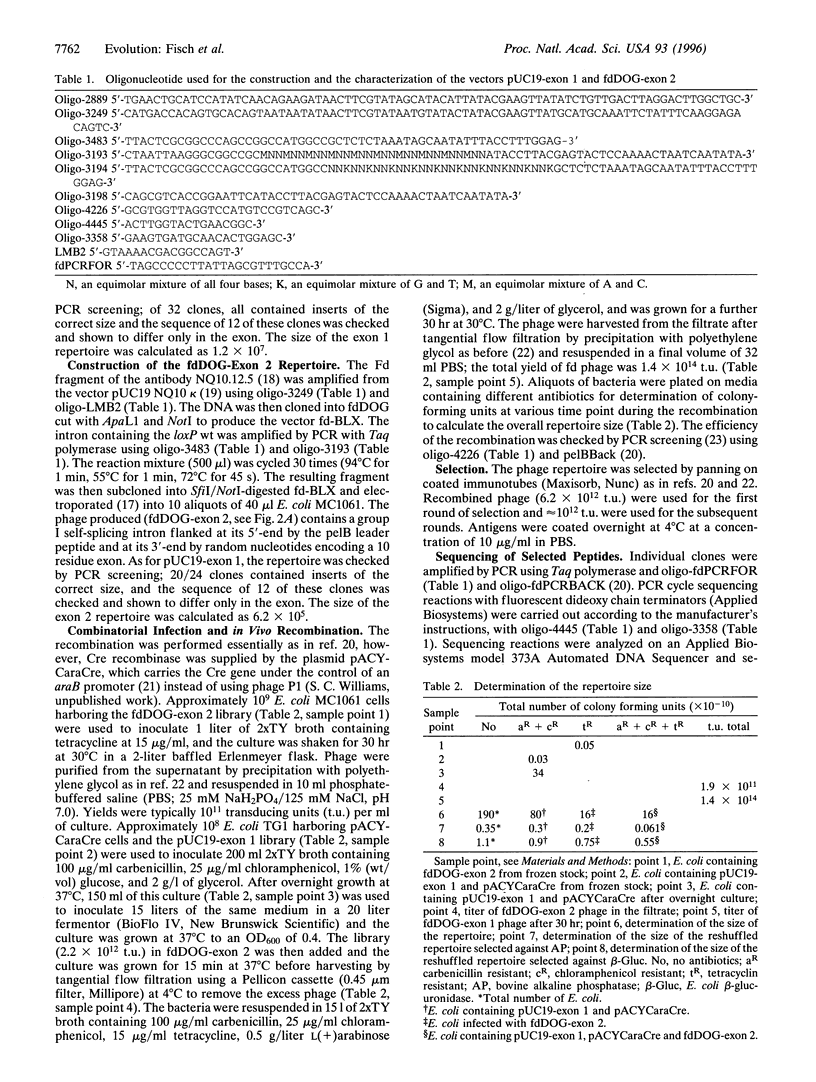
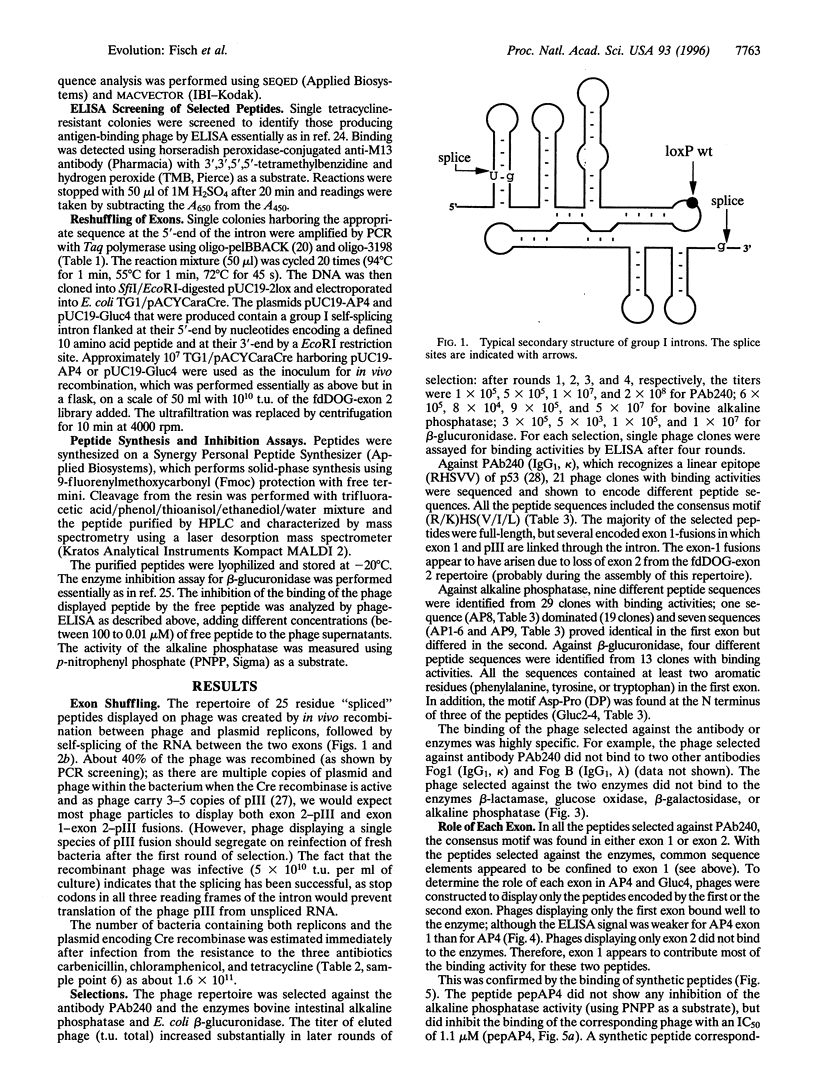
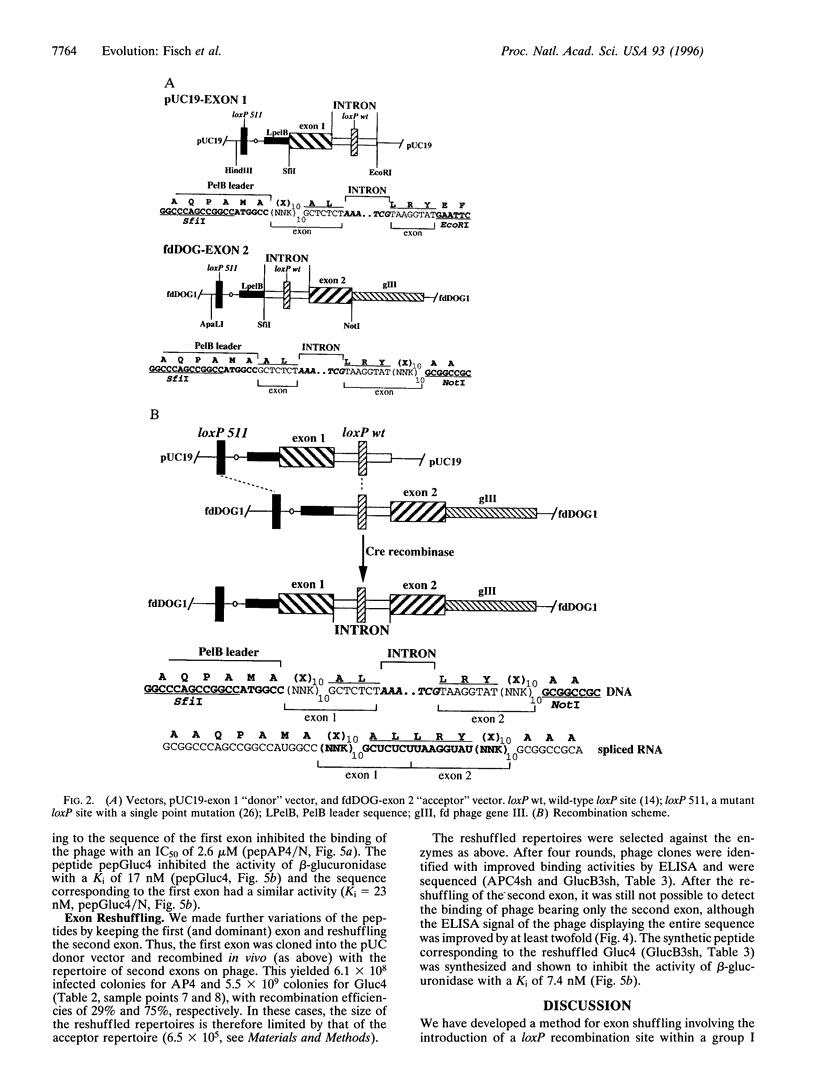
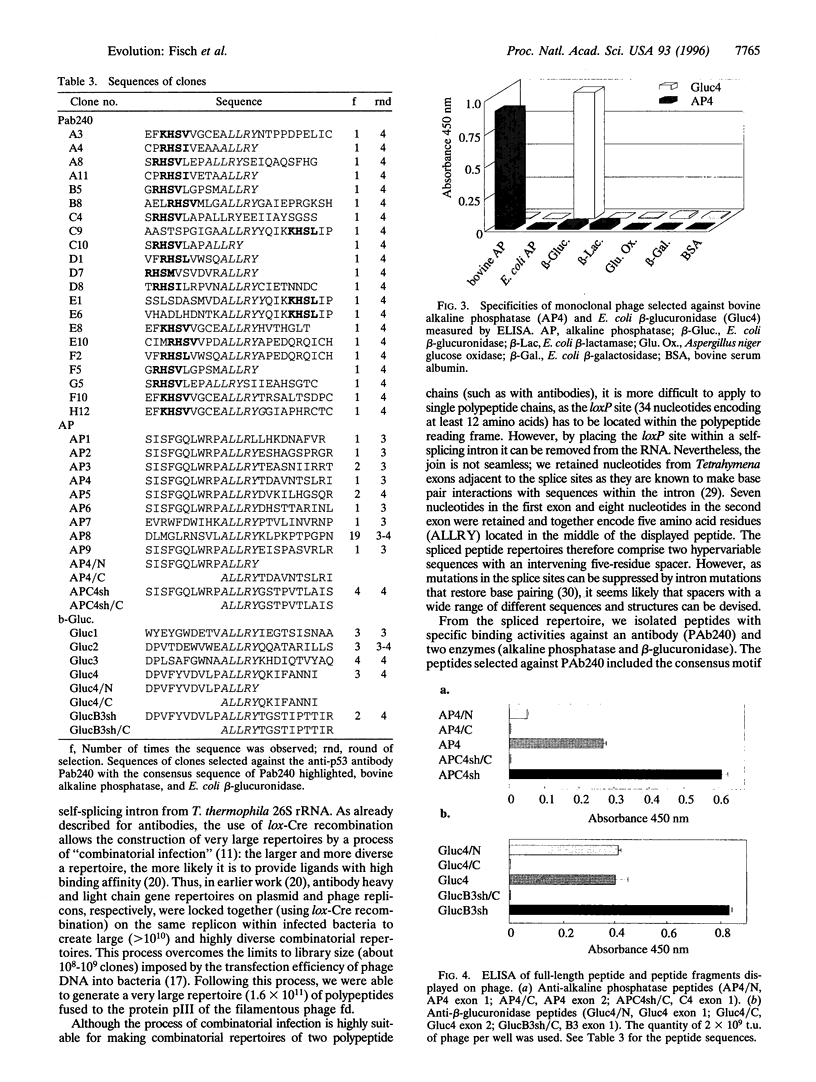
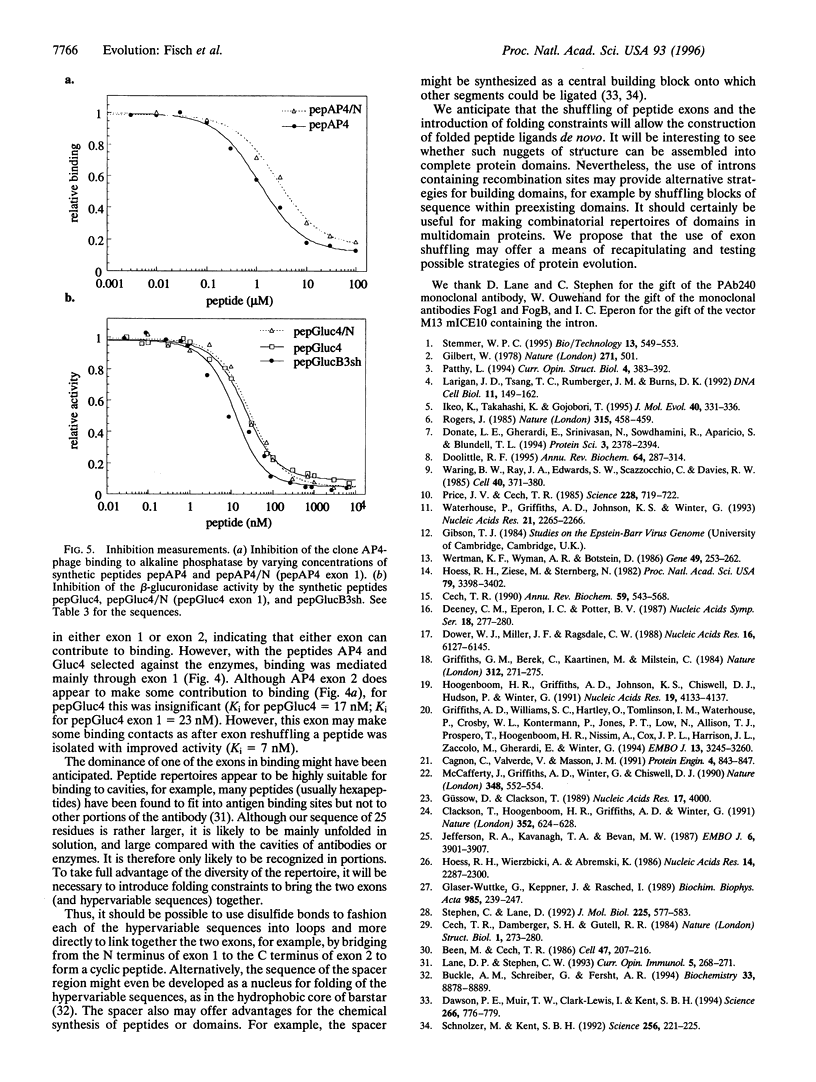
Images in this article
Selected References
These references are in PubMed. This may not be the complete list of references from this article.
- Been M. D., Cech T. R. One binding site determines sequence specificity of Tetrahymena pre-rRNA self-splicing, trans-splicing, and RNA enzyme activity. Cell. 1986 Oct 24;47(2):207–216. doi: 10.1016/0092-8674(86)90443-5. [DOI] [PubMed] [Google Scholar]
- Buckle A. M., Schreiber G., Fersht A. R. Protein-protein recognition: crystal structural analysis of a barnase-barstar complex at 2.0-A resolution. Biochemistry. 1994 Aug 2;33(30):8878–8889. doi: 10.1021/bi00196a004. [DOI] [PubMed] [Google Scholar]
- Cagnon C., Valverde V., Masson J. M. A new family of sugar-inducible expression vectors for Escherichia coli. Protein Eng. 1991 Oct;4(7):843–847. doi: 10.1093/protein/4.7.843. [DOI] [PubMed] [Google Scholar]
- Cech T. R., Damberger S. H., Gutell R. R. Representation of the secondary and tertiary structure of group I introns. Nat Struct Biol. 1994 May;1(5):273–280. doi: 10.1038/nsb0594-273. [DOI] [PubMed] [Google Scholar]
- Cech T. R. Self-splicing of group I introns. Annu Rev Biochem. 1990;59:543–568. doi: 10.1146/annurev.bi.59.070190.002551. [DOI] [PubMed] [Google Scholar]
- Clackson T., Hoogenboom H. R., Griffiths A. D., Winter G. Making antibody fragments using phage display libraries. Nature. 1991 Aug 15;352(6336):624–628. doi: 10.1038/352624a0. [DOI] [PubMed] [Google Scholar]
- Dawson P. E., Muir T. W., Clark-Lewis I., Kent S. B. Synthesis of proteins by native chemical ligation. Science. 1994 Nov 4;266(5186):776–779. doi: 10.1126/science.7973629. [DOI] [PubMed] [Google Scholar]
- Deeney C. M., Eperon I. C., Potter B. V. Self-splicing of Tetrahymena rRNA can proceed with phosphorothioate substitution at the splice sites. Nucleic Acids Symp Ser. 1987;(18):277–280. [PubMed] [Google Scholar]
- Donate L. E., Gherardi E., Srinivasan N., Sowdhamini R., Aparicio S., Blundell T. L. Molecular evolution and domain structure of plasminogen-related growth factors (HGF/SF and HGF1/MSP). Protein Sci. 1994 Dec;3(12):2378–2394. doi: 10.1002/pro.5560031222. [DOI] [PMC free article] [PubMed] [Google Scholar]
- Doolittle R. F. The multiplicity of domains in proteins. Annu Rev Biochem. 1995;64:287–314. doi: 10.1146/annurev.bi.64.070195.001443. [DOI] [PubMed] [Google Scholar]
- Dower W. J., Miller J. F., Ragsdale C. W. High efficiency transformation of E. coli by high voltage electroporation. Nucleic Acids Res. 1988 Jul 11;16(13):6127–6145. doi: 10.1093/nar/16.13.6127. [DOI] [PMC free article] [PubMed] [Google Scholar]
- Gilbert W. Why genes in pieces? Nature. 1978 Feb 9;271(5645):501–501. doi: 10.1038/271501a0. [DOI] [PubMed] [Google Scholar]
- Glaser-Wuttke G., Keppner J., Rasched I. Pore-forming properties of the adsorption protein of filamentous phage fd. Biochim Biophys Acta. 1989 Nov 3;985(3):239–247. doi: 10.1016/0005-2736(89)90408-2. [DOI] [PubMed] [Google Scholar]
- Griffiths A. D., Williams S. C., Hartley O., Tomlinson I. M., Waterhouse P., Crosby W. L., Kontermann R. E., Jones P. T., Low N. M., Allison T. J. Isolation of high affinity human antibodies directly from large synthetic repertoires. EMBO J. 1994 Jul 15;13(14):3245–3260. doi: 10.1002/j.1460-2075.1994.tb06626.x. [DOI] [PMC free article] [PubMed] [Google Scholar]
- Griffiths G. M., Berek C., Kaartinen M., Milstein C. Somatic mutation and the maturation of immune response to 2-phenyl oxazolone. Nature. 1984 Nov 15;312(5991):271–275. doi: 10.1038/312271a0. [DOI] [PubMed] [Google Scholar]
- Güssow D., Clackson T. Direct clone characterization from plaques and colonies by the polymerase chain reaction. Nucleic Acids Res. 1989 May 25;17(10):4000–4000. doi: 10.1093/nar/17.10.4000. [DOI] [PMC free article] [PubMed] [Google Scholar]
- Hoess R. H., Wierzbicki A., Abremski K. The role of the loxP spacer region in P1 site-specific recombination. Nucleic Acids Res. 1986 Mar 11;14(5):2287–2300. doi: 10.1093/nar/14.5.2287. [DOI] [PMC free article] [PubMed] [Google Scholar]
- Hoess R. H., Ziese M., Sternberg N. P1 site-specific recombination: nucleotide sequence of the recombining sites. Proc Natl Acad Sci U S A. 1982 Jun;79(11):3398–3402. doi: 10.1073/pnas.79.11.3398. [DOI] [PMC free article] [PubMed] [Google Scholar]
- Hoogenboom H. R., Griffiths A. D., Johnson K. S., Chiswell D. J., Hudson P., Winter G. Multi-subunit proteins on the surface of filamentous phage: methodologies for displaying antibody (Fab) heavy and light chains. Nucleic Acids Res. 1991 Aug 11;19(15):4133–4137. doi: 10.1093/nar/19.15.4133. [DOI] [PMC free article] [PubMed] [Google Scholar]
- Ikeo K., Takahashi K., Gojobori T. Different evolutionary histories of kringle and protease domains in serine proteases: a typical example of domain evolution. J Mol Evol. 1995 Mar;40(3):331–336. doi: 10.1007/BF00163238. [DOI] [PubMed] [Google Scholar]
- Jefferson R. A., Kavanagh T. A., Bevan M. W. GUS fusions: beta-glucuronidase as a sensitive and versatile gene fusion marker in higher plants. EMBO J. 1987 Dec 20;6(13):3901–3907. doi: 10.1002/j.1460-2075.1987.tb02730.x. [DOI] [PMC free article] [PubMed] [Google Scholar]
- Lane D. P., Stephen C. W. Epitope mapping using bacteriophage peptide libraries. Curr Opin Immunol. 1993 Apr;5(2):268–271. doi: 10.1016/0952-7915(93)90016-l. [DOI] [PubMed] [Google Scholar]
- Larigan J. D., Tsang T. C., Rumberger J. M., Burns D. K. Characterization of cDNA and genomic sequences encoding rabbit ELAM-1: conservation of structure and functional interactions with leukocytes. DNA Cell Biol. 1992 Mar;11(2):149–162. doi: 10.1089/dna.1992.11.149. [DOI] [PubMed] [Google Scholar]
- McCafferty J., Griffiths A. D., Winter G., Chiswell D. J. Phage antibodies: filamentous phage displaying antibody variable domains. Nature. 1990 Dec 6;348(6301):552–554. doi: 10.1038/348552a0. [DOI] [PubMed] [Google Scholar]
- Price J. V., Cech T. R. Coupling of Tetrahymena ribosomal RNA splicing to beta-galactosidase expression in Escherichia coli. Science. 1985 May 10;228(4700):719–722. doi: 10.1126/science.2986286. [DOI] [PubMed] [Google Scholar]
- Rogers J. Exon shuffling and intron insertion in serine protease genes. Nature. 1985 Jun 6;315(6019):458–459. doi: 10.1038/315458a0. [DOI] [PubMed] [Google Scholar]
- Schnölzer M., Kent S. B. Constructing proteins by dovetailing unprotected synthetic peptides: backbone-engineered HIV protease. Science. 1992 Apr 10;256(5054):221–225. doi: 10.1126/science.1566069. [DOI] [PubMed] [Google Scholar]
- Stephen C. W., Lane D. P. Mutant conformation of p53. Precise epitope mapping using a filamentous phage epitope library. J Mol Biol. 1992 Jun 5;225(3):577–583. doi: 10.1016/0022-2836(92)90386-x. [DOI] [PubMed] [Google Scholar]
- Waring R. B., Ray J. A., Edwards S. W., Scazzocchio C., Davies R. W. The Tetrahymena rRNA intron self-splices in E. coli: in vivo evidence for the importance of key base-paired regions of RNA for RNA enzyme function. Cell. 1985 Feb;40(2):371–380. doi: 10.1016/0092-8674(85)90151-5. [DOI] [PubMed] [Google Scholar]
- Waterhouse P., Griffiths A. D., Johnson K. S., Winter G. Combinatorial infection and in vivo recombination: a strategy for making large phage antibody repertoires. Nucleic Acids Res. 1993 May 11;21(9):2265–2266. doi: 10.1093/nar/21.9.2265. [DOI] [PMC free article] [PubMed] [Google Scholar]
- Wertman K. F., Wyman A. R., Botstein D. Host/vector interactions which affect the viability of recombinant phage lambda clones. Gene. 1986;49(2):253–262. doi: 10.1016/0378-1119(86)90286-6. [DOI] [PubMed] [Google Scholar]