Abstract
The effect of histone H1 binding on the cleavage of superhelical plasmids by single-strand-specific nucleases was investigated. Mapping of P1 cleavage sites in pBR322, achieved by EcoRI digestion after the original P1 attack, showed an intriguing phenomenon: preexisting susceptible sites became "protected," whereas some new sites appeared at high levels of H1. Similar results were obtained with another single-strand-specific nuclease, S1. Disappearance of cutting at preexisting sites and appearance of new sites was also observed in a derivative plasmid that contains a 36-bp stretch of alternating d(AT) sequence that is known to adopt an altered P1-sensitive conformation. On the other hand, H1 titration of a dimerized version of the d(AT)18-containing plasmid led to protection of all preexisting sites except the d(AT)18 inserts, which were still cut even at high H1 levels; in this plasmid no new sites appeared. The protection of preexisting sites is best explained by long-range effects of histone H1 binding on the superhelical torsion of the plasmid. The appearance of new sites, on the other hand, probably also involves a local effect of stabilization of specific sequences in Pl-sensitive conformation, due to direct H1 binding to such sequences. That such binding involves linker histone N- and/or C-terminal tails is indicated by the fact that titration with the globular domain of H5, while causing disappearance of preexisting sites, does not lead to the appearance of any new sites.
Full text
PDF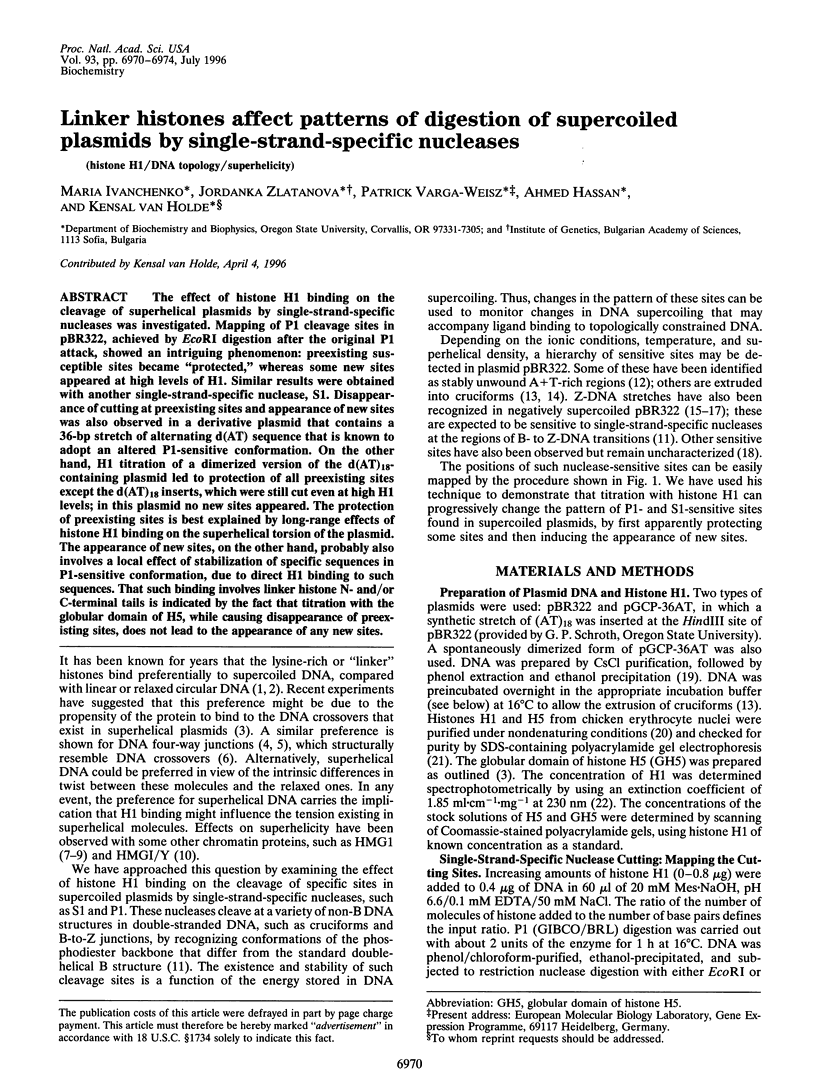
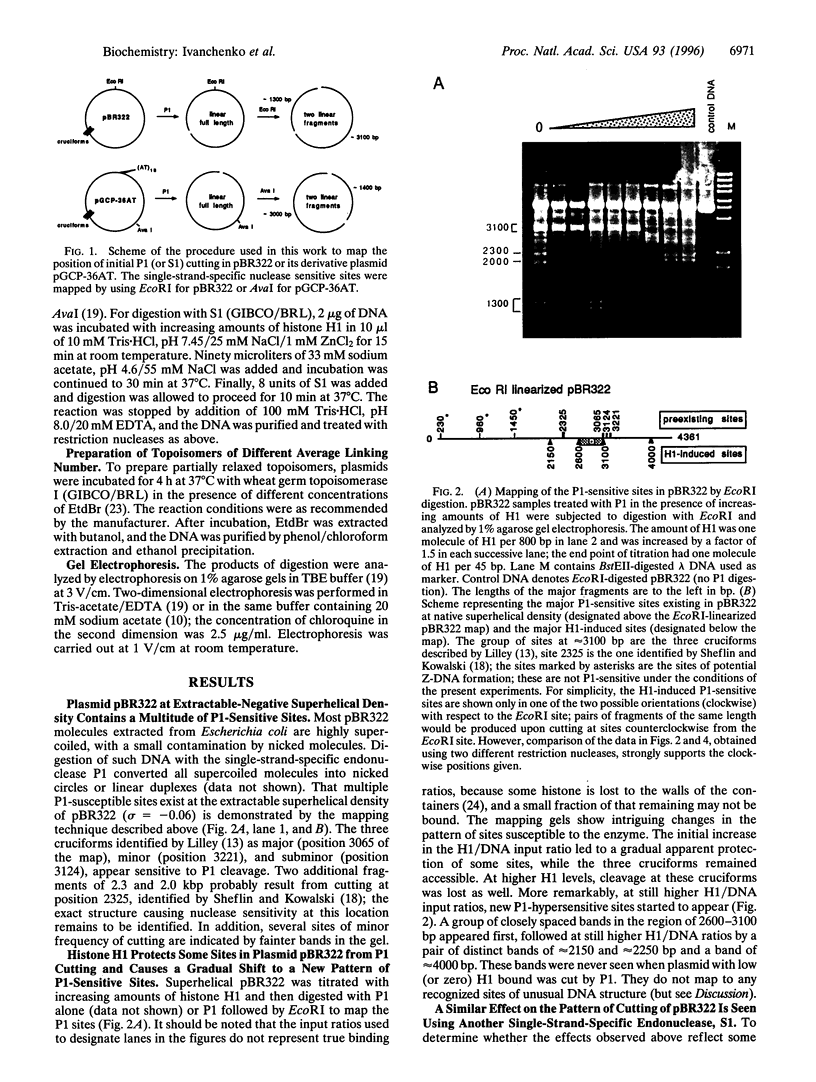
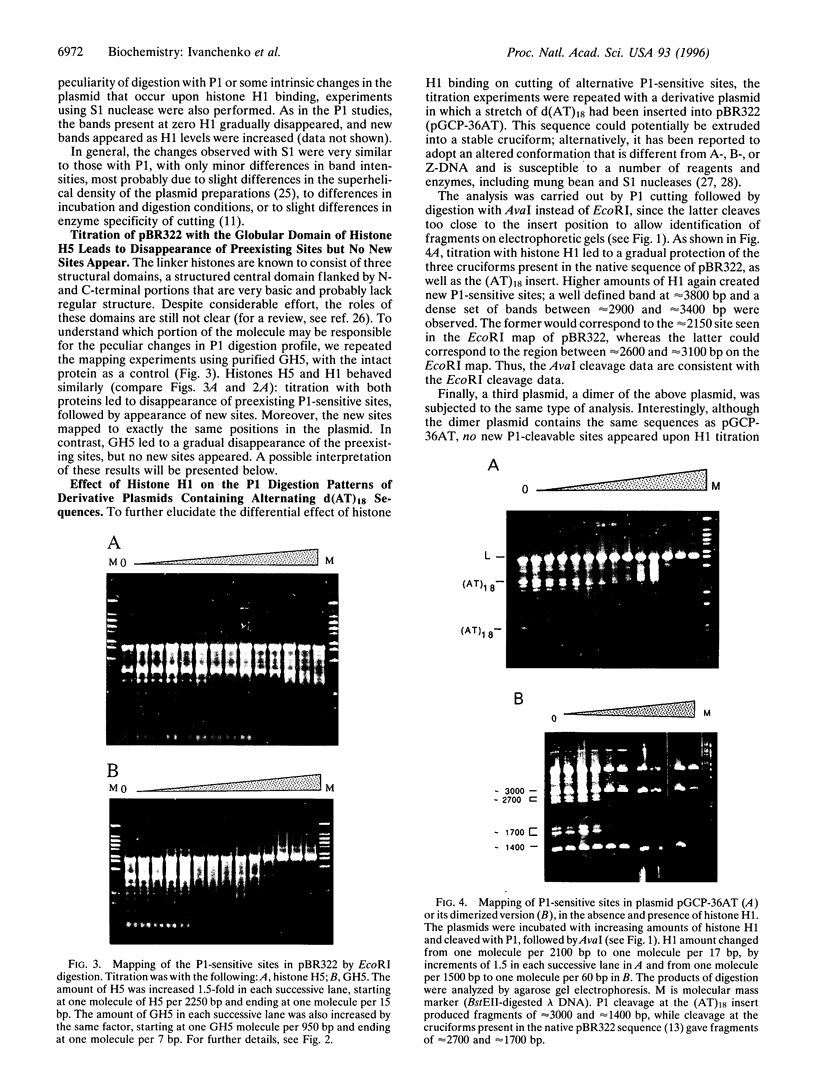
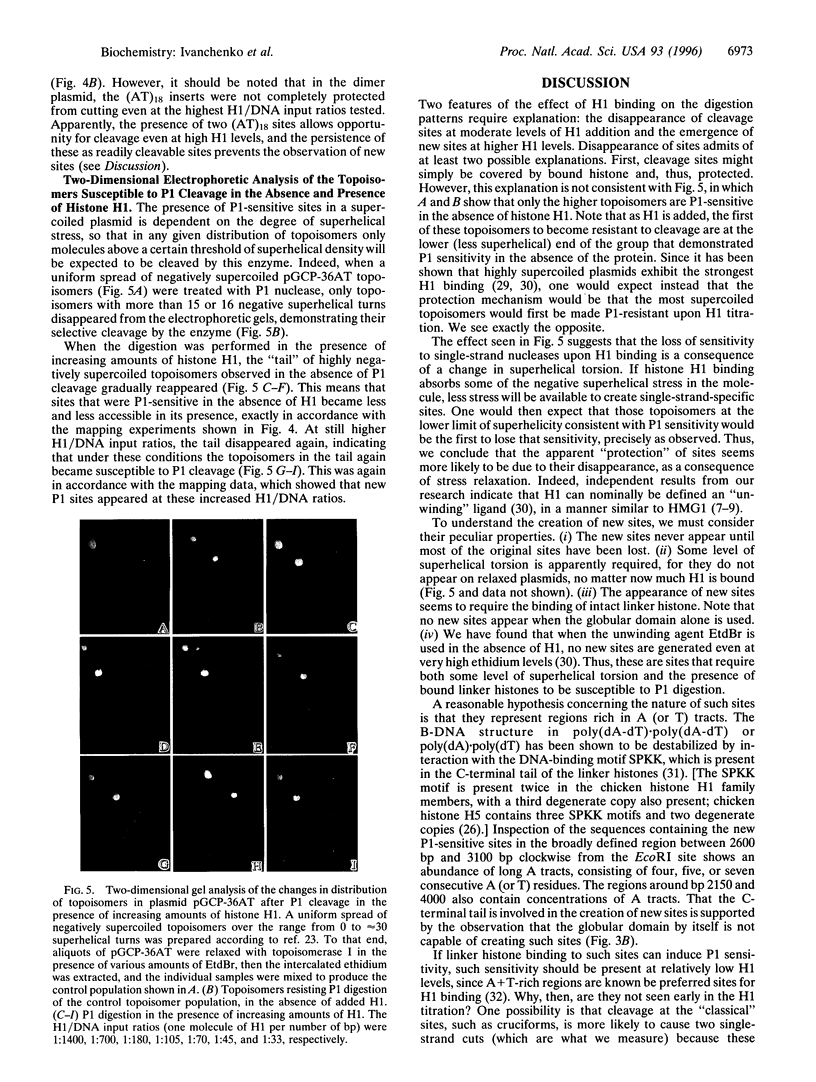
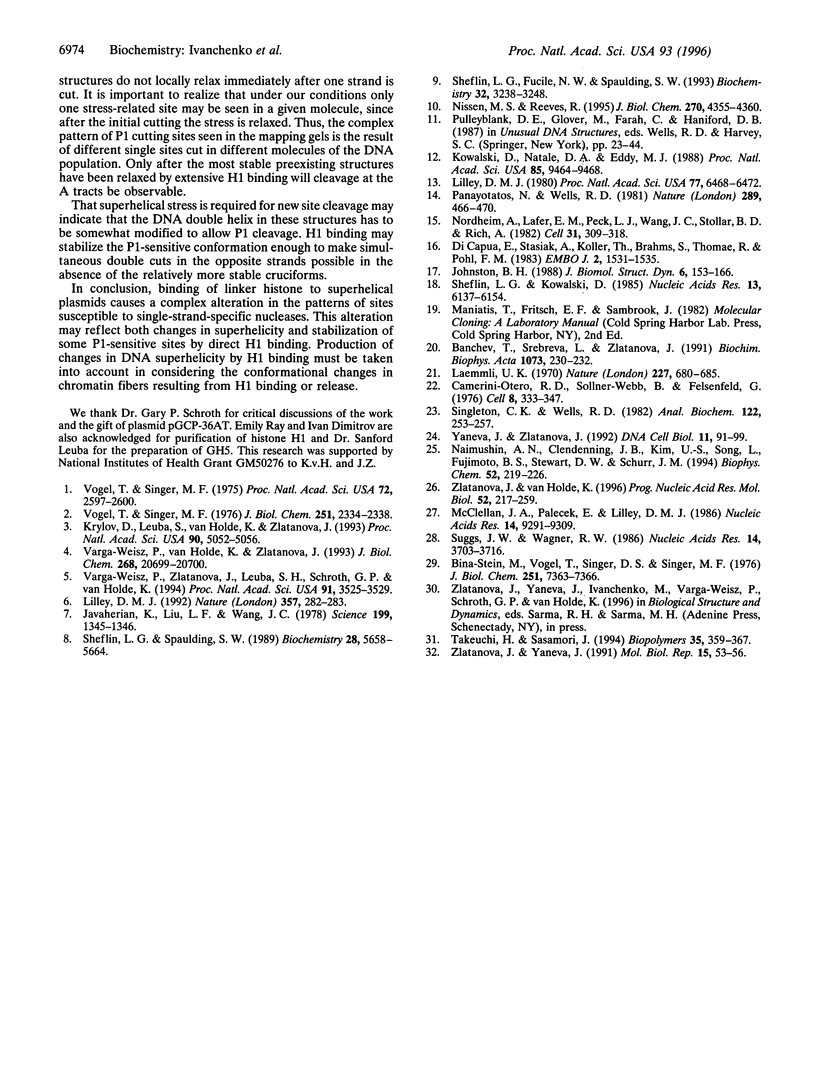
Images in this article
Selected References
These references are in PubMed. This may not be the complete list of references from this article.
- Banchev T., Srebreva L., Zlatanova J. Purification of histone H10 and its subfractions under non-denaturing conditions. Biochim Biophys Acta. 1991 Jan 23;1073(1):230–232. doi: 10.1016/0304-4165(91)90208-x. [DOI] [PubMed] [Google Scholar]
- Bina-Stein M., Vogel T., Singer D. S., Singer M. F. H5 Histone and DNA-relaxing enzyme of chicken erythrocytes. Interaction with superhelical DNA. J Biol Chem. 1976 Dec 10;251(23):7363–7366. [PubMed] [Google Scholar]
- Camerini-Otero R. D., Sollner-Webb B., Felsenfeld G. The organization of histones and DNA in chromatin: evidence for an arginine-rich histone kernel. Cell. 1976 Jul;8(3):333–347. doi: 10.1016/0092-8674(76)90145-8. [DOI] [PubMed] [Google Scholar]
- Di Capua E., Stasiak A., Koller T., Brahms S., Thomae R., Pohl F. M. Torsional stress induces left-handed helical stretches in DNA of natural base sequence: circular dichroism and antibody binding. EMBO J. 1983;2(9):1531–1535. doi: 10.1002/j.1460-2075.1983.tb01619.x. [DOI] [PMC free article] [PubMed] [Google Scholar]
- Javaherian K., Liu J. F., Wang J. C. Nonhistone proteins HMG1 and HMG2 change the DNA helical structure. Science. 1978 Mar 24;199(4335):1345–1346. doi: 10.1126/science.628842. [DOI] [PubMed] [Google Scholar]
- Johnston B. H. Chemical probing of the B-Z transition in negatively supercoiled DNA. J Biomol Struct Dyn. 1988 Aug;6(1):153–166. doi: 10.1080/07391102.1988.10506488. [DOI] [PubMed] [Google Scholar]
- Kowalski D., Natale D. A., Eddy M. J. Stable DNA unwinding, not "breathing," accounts for single-strand-specific nuclease hypersensitivity of specific A+T-rich sequences. Proc Natl Acad Sci U S A. 1988 Dec;85(24):9464–9468. doi: 10.1073/pnas.85.24.9464. [DOI] [PMC free article] [PubMed] [Google Scholar]
- Krylov D., Leuba S., van Holde K., Zlatanova J. Histones H1 and H5 interact preferentially with crossovers of double-helical DNA. Proc Natl Acad Sci U S A. 1993 Jun 1;90(11):5052–5056. doi: 10.1073/pnas.90.11.5052. [DOI] [PMC free article] [PubMed] [Google Scholar]
- Laemmli U. K. Cleavage of structural proteins during the assembly of the head of bacteriophage T4. Nature. 1970 Aug 15;227(5259):680–685. doi: 10.1038/227680a0. [DOI] [PubMed] [Google Scholar]
- Lilley D. M. DNA--protein interactions. HMG has DNA wrapped up. Nature. 1992 May 28;357(6376):282–283. doi: 10.1038/357282a0. [DOI] [PubMed] [Google Scholar]
- Lilley D. M. The inverted repeat as a recognizable structural feature in supercoiled DNA molecules. Proc Natl Acad Sci U S A. 1980 Nov;77(11):6468–6472. doi: 10.1073/pnas.77.11.6468. [DOI] [PMC free article] [PubMed] [Google Scholar]
- McClellan J. A., Palecek E., Lilley D. M. (A-T)n tracts embedded in random sequence DNA--formation of a structure which is chemically reactive and torsionally deformable. Nucleic Acids Res. 1986 Dec 9;14(23):9291–9309. doi: 10.1093/nar/14.23.9291. [DOI] [PMC free article] [PubMed] [Google Scholar]
- Naimushin A. N., Clendenning J. B., Kim U. S., Song L., Fujimoto B. S., Stewart D. W., Schurr J. M. Effect of ethidium binding and superhelix density on the apparent supercoiling free energy and torsion constant of pBR322 DNA. Biophys Chem. 1994 Nov;52(3):219–226. doi: 10.1016/0301-4622(94)00037-k. [DOI] [PubMed] [Google Scholar]
- Nissen M. S., Reeves R. Changes in superhelicity are introduced into closed circular DNA by binding of high mobility group protein I/Y. J Biol Chem. 1995 Mar 3;270(9):4355–4360. doi: 10.1074/jbc.270.9.4355. [DOI] [PubMed] [Google Scholar]
- Nordheim A., Lafer E. M., Peck L. J., Wang J. C., Stollar B. D., Rich A. Negatively supercoiled plasmids contain left-handed Z-DNA segments as detected by specific antibody binding. Cell. 1982 Dec;31(2 Pt 1):309–318. doi: 10.1016/0092-8674(82)90124-6. [DOI] [PubMed] [Google Scholar]
- Panayotatos N., Wells R. D. Cruciform structures in supercoiled DNA. Nature. 1981 Feb 5;289(5797):466–470. doi: 10.1038/289466a0. [DOI] [PubMed] [Google Scholar]
- Sheflin L. G., Fucile N. W., Spaulding S. W. The specific interactions of HMG 1 and 2 with negatively supercoiled DNA are modulated by their acidic C-terminal domains and involve cysteine residues in their HMG 1/2 boxes. Biochemistry. 1993 Apr 6;32(13):3238–3248. doi: 10.1021/bi00064a005. [DOI] [PubMed] [Google Scholar]
- Sheflin L. G., Kowalski D. Altered DNA conformations detected by mung bean nuclease occur in promoter and terminator regions of supercoiled pBR322 DNA. Nucleic Acids Res. 1985 Sep 11;13(17):6137–6154. doi: 10.1093/nar/13.17.6137. [DOI] [PMC free article] [PubMed] [Google Scholar]
- Sheflin L. G., Spaulding S. W. High mobility group protein 1 preferentially conserves torsion in negatively supercoiled DNA. Biochemistry. 1989 Jun 27;28(13):5658–5664. doi: 10.1021/bi00439a048. [DOI] [PubMed] [Google Scholar]
- Singleton C. K., Wells R. D. The facile generation of covalently closed, circular DNAs with defined negative superhelical densities. Anal Biochem. 1982 May 15;122(2):253–257. doi: 10.1016/0003-2697(82)90277-9. [DOI] [PubMed] [Google Scholar]
- Suggs J. W., Wagner R. W. Nuclease recognition of an alternating structure in a d(AT)14 plasmid insert. Nucleic Acids Res. 1986 May 12;14(9):3703–3716. doi: 10.1093/nar/14.9.3703. [DOI] [PMC free article] [PubMed] [Google Scholar]
- Takeuchi H., Sasamori J. Structural modification of DNA by a DNA-binding motif SPKK: detection of changes in base-pair hydrogen bonding and base stacking by UV resonance Raman spectroscopy. Biopolymers. 1995 Apr;35(4):359–367. doi: 10.1002/bip.360350403. [DOI] [PubMed] [Google Scholar]
- Varga-Weisz P., Zlatanova J., Leuba S. H., Schroth G. P., van Holde K. Binding of histones H1 and H5 and their globular domains to four-way junction DNA. Proc Natl Acad Sci U S A. 1994 Apr 26;91(9):3525–3529. doi: 10.1073/pnas.91.9.3525. [DOI] [PMC free article] [PubMed] [Google Scholar]
- Varga-Weisz P., van Holde K., Zlatanova J. Preferential binding of histone H1 to four-way helical junction DNA. J Biol Chem. 1993 Oct 5;268(28):20699–20700. [PubMed] [Google Scholar]
- Vogel T., Singer M. F. Interaction of f1 histone with superhelical DNA. Proc Natl Acad Sci U S A. 1975 Jul;72(7):2597–2600. doi: 10.1073/pnas.72.7.2597. [DOI] [PMC free article] [PubMed] [Google Scholar]
- Vogel T., Singer M. F. The effect of superhelicity on the interaction of histone f1 with closed circular duplex DNA. J Biol Chem. 1976 Apr 25;251(8):2334–2338. [PubMed] [Google Scholar]
- Yaneva J., Zlatanova J. Histone H1 interacts specifically with certain regions of the mouse alpha-globin gene. DNA Cell Biol. 1992 Mar;11(2):91–99. doi: 10.1089/dna.1992.11.91. [DOI] [PubMed] [Google Scholar]
- Zlatanova J., Yaneva J. DNA sequence specific interactions of histone H1. Mol Biol Rep. 1991 Feb;15(1):53–56. doi: 10.1007/BF00369901. [DOI] [PubMed] [Google Scholar]
- Zlatanova J., van Holde K. The linker histones and chromatin structure: new twists. Prog Nucleic Acid Res Mol Biol. 1996;52:217–259. doi: 10.1016/s0079-6603(08)60968-x. [DOI] [PubMed] [Google Scholar]