Abstract
Active ion transport systems with fixed stoichiometry are subject to a thermodynamic limit on the ion concentration gradients that they can generate and maintain, and their net rates of transport must inevitably decrease as this limit is approached. The capability to vary stoichiometry might thus be physiologically advantageous: a shift to lower stoichiometry (fewer ions pumped per reaction cycle) at increasing thermodynamic load could increase the limit on the supportable concentration gradient and could accelerate the rate of transport under high-load conditions. Here we present a theoretical and numerical analysis of this possibility, using the sarcoplasmic reticulum ATP-driven Ca pump as the example. It is easy to introduce alternate pathways into the reaction cycle for this system to shift the stoichiometry (Ca2+/ATP) from the normal value of 2:1 to 1:1, but it cannot be done without simultaneous generation of a pathway for uncoupled leak of Ca2+ across the membrane. This counteracts the advantageous effect of the change in transport stoichiometry and a physiologically useful rate acceleration cannot be obtained. This result is likely to be generally applicable to most active transport systems.
Full text
PDF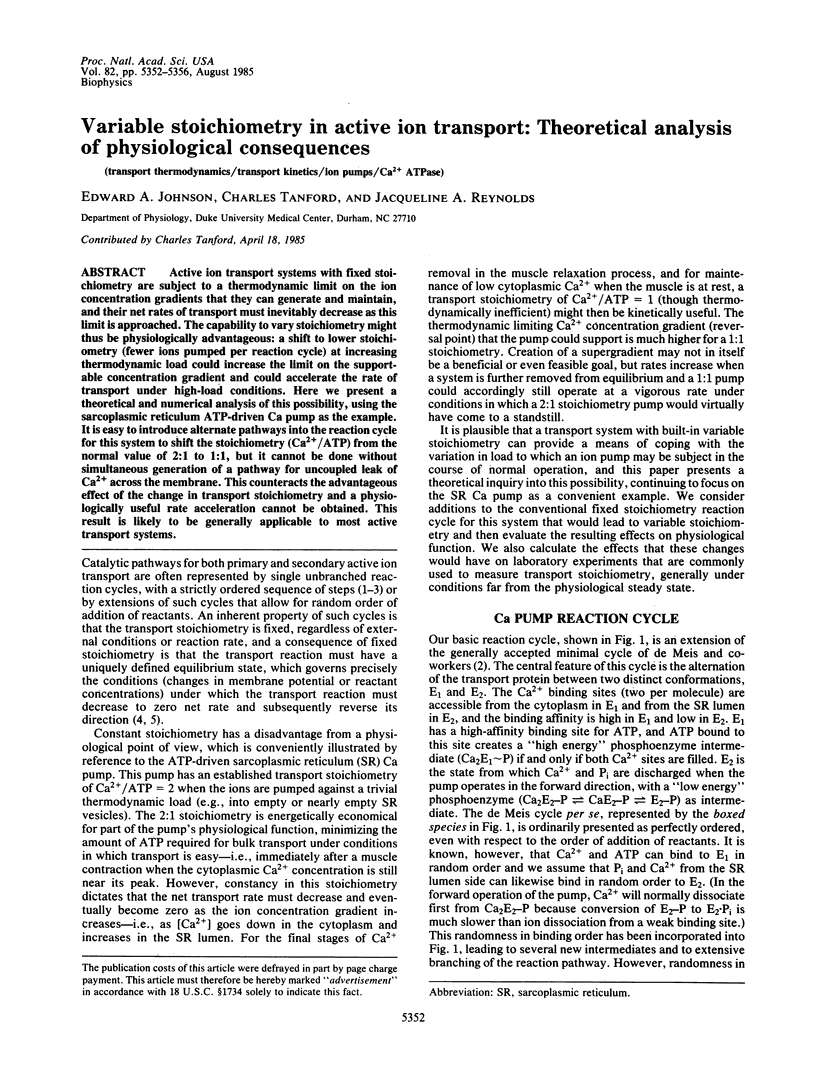
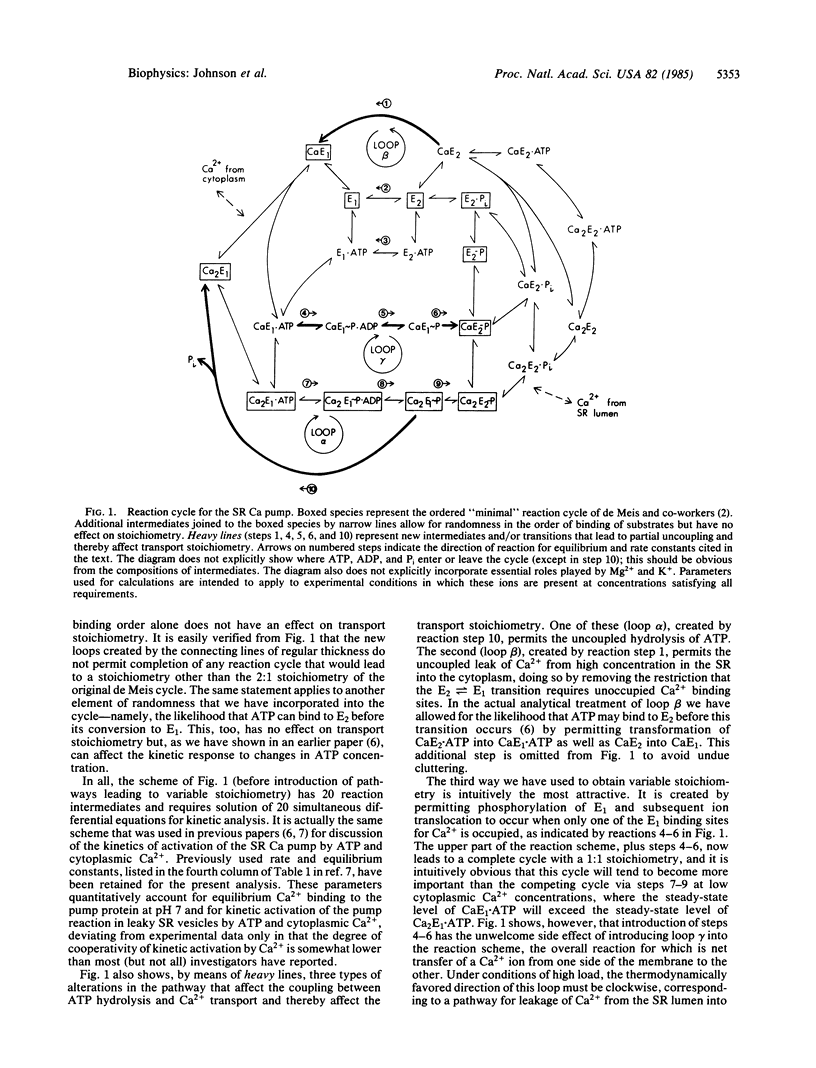
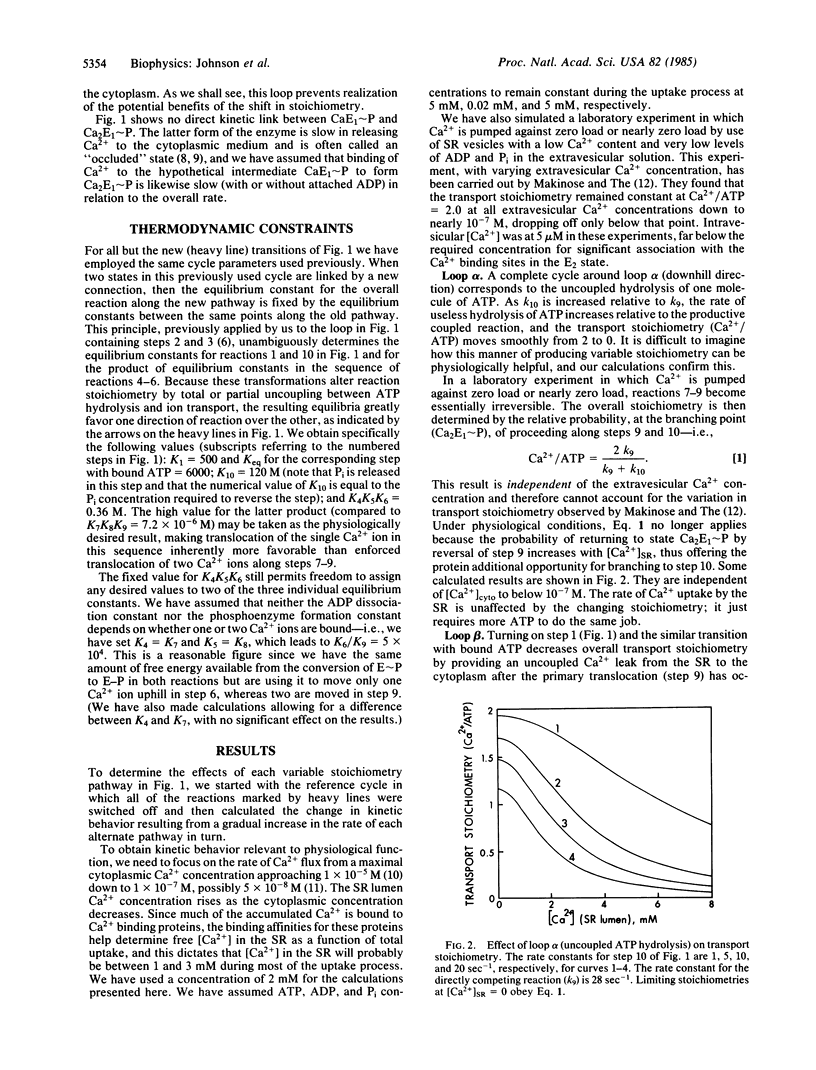
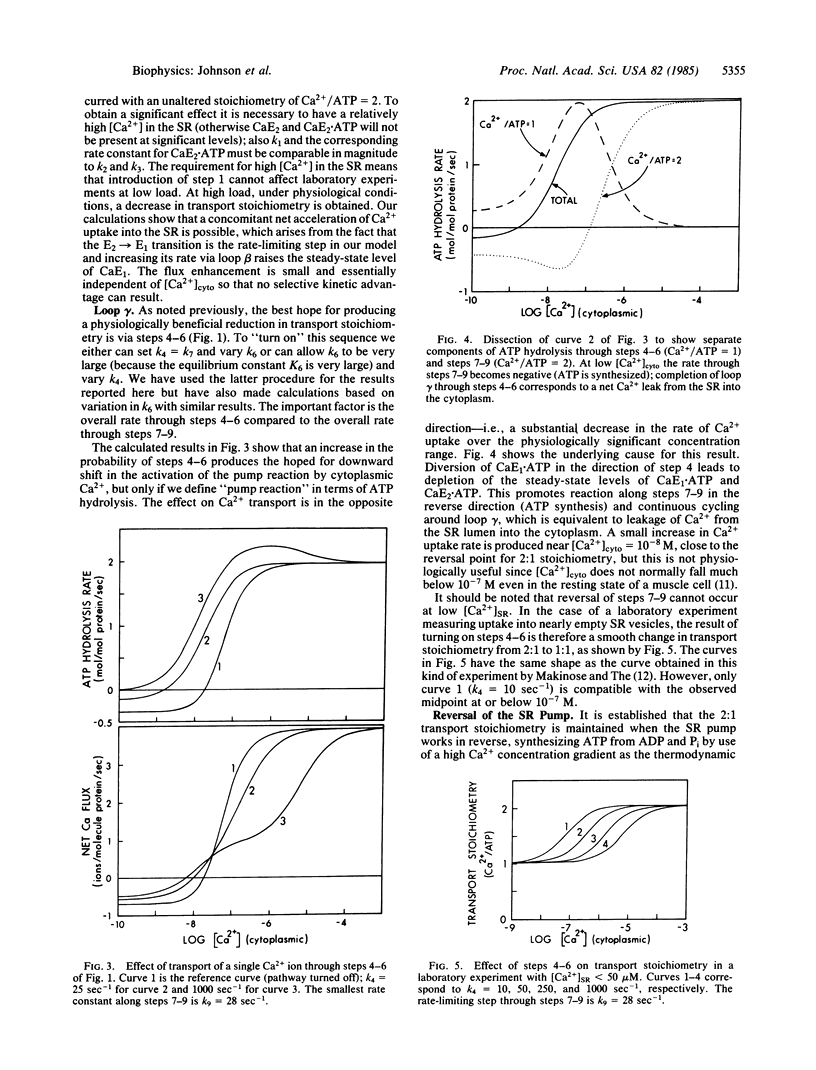
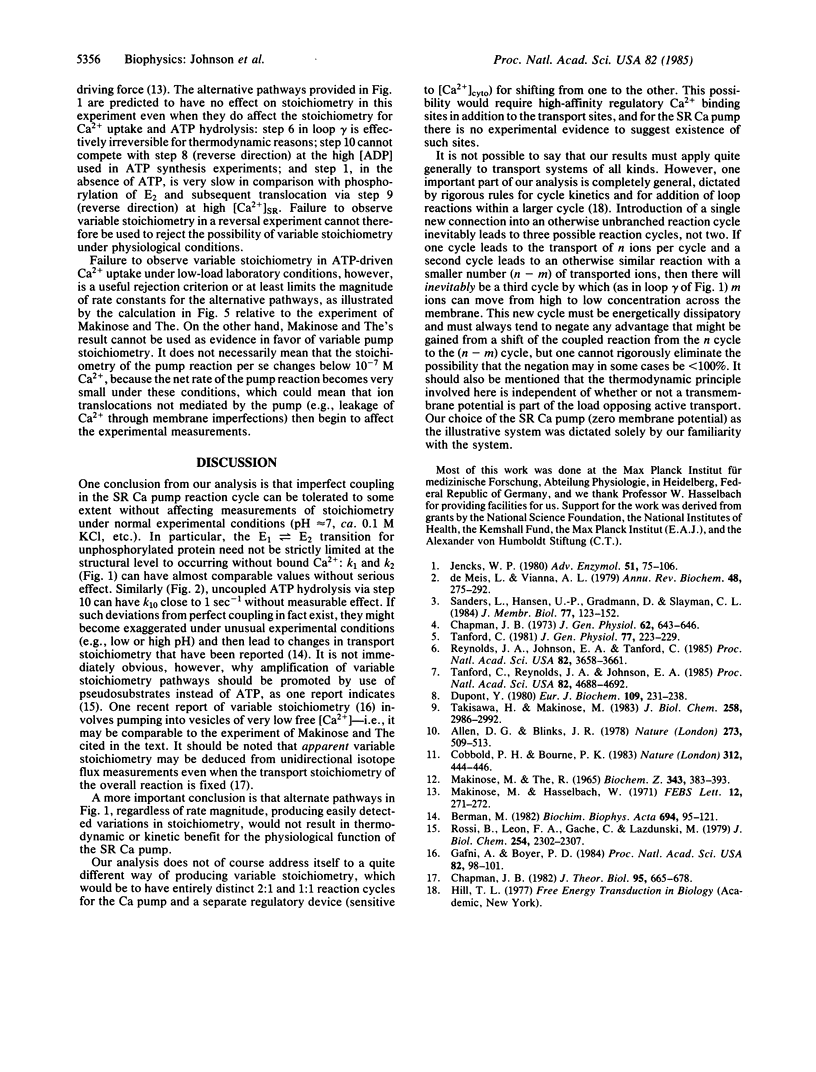
Selected References
These references are in PubMed. This may not be the complete list of references from this article.
- Allen D. G., Blinks J. R. Calcium transients in aequorin-injected frog cardiac muscle. Nature. 1978 Jun 15;273(5663):509–513. doi: 10.1038/273509a0. [DOI] [PubMed] [Google Scholar]
- Berman M. C. Energy coupling and uncoupling of active calcium transport by sarcoplasmic reticulum membranes. Biochim Biophys Acta. 1982 Aug 11;694(1):95–121. doi: 10.1016/0304-4157(82)90015-6. [DOI] [PubMed] [Google Scholar]
- Chapman J. B. A kinetic interpretation of "variable" stoichiometry for an electrogenic sodium pump obeying chemiosmotic principles. J Theor Biol. 1982 Apr 21;95(4):665–678. doi: 10.1016/0022-5193(82)90347-2. [DOI] [PubMed] [Google Scholar]
- Chapman J. B. Letter: On the reversibility of the sodium pump in dialyzed squid axons. A method for determining the free energy of ATP breakdown? J Gen Physiol. 1973 Nov;62(5):643–646. doi: 10.1085/jgp.62.5.643. [DOI] [PMC free article] [PubMed] [Google Scholar]
- Cobbold P. H., Bourne P. K. Aequorin measurements of free calcium in single heart cells. 1984 Nov 29-Dec 5Nature. 312(5993):444–446. doi: 10.1038/312444a0. [DOI] [PubMed] [Google Scholar]
- Dupont Y. Occlusion of divalent cations in the phosphorylated calcium pump of sarcoplasmic reticulum. Eur J Biochem. 1980 Aug;109(1):231–238. doi: 10.1111/j.1432-1033.1980.tb04788.x. [DOI] [PubMed] [Google Scholar]
- Gafni A., Boyer P. D. Modulation of stoichiometry of the sarcoplasmic reticulum calcium pump may enhance thermodynamic efficiency. Proc Natl Acad Sci U S A. 1985 Jan;82(1):98–101. doi: 10.1073/pnas.82.1.98. [DOI] [PMC free article] [PubMed] [Google Scholar]
- Jencks W. P. The utilization of binding energy in coupled vectorial processes. Adv Enzymol Relat Areas Mol Biol. 1980;51:75–106. doi: 10.1002/9780470122969.ch2. [DOI] [PubMed] [Google Scholar]
- Makinose M., Hasselbach W. ATP synthesis by the reverse of the sarcoplasmic calcium pump. FEBS Lett. 1971 Jan 30;12(5):271–272. doi: 10.1016/0014-5793(71)80196-5. [DOI] [PubMed] [Google Scholar]
- Makinose M., The R. Calcium-Akkumulation und Nucleosidtriphosphat-Spaltung durch die Vesikel des sarkoplasmatischen Reticulum. Biochem Z. 1965 Dec 31;343(4):383–393. [PubMed] [Google Scholar]
- Reynolds J. A., Johnson E. A., Tanford C. Application of the principle of linked functions to ATP-driven ion pumps: kinetics of activation by ATP. Proc Natl Acad Sci U S A. 1985 Jun;82(11):3658–3661. doi: 10.1073/pnas.82.11.3658. [DOI] [PMC free article] [PubMed] [Google Scholar]
- Rossi B., de Assis Leone F., Gache C., Lazdunski M. Pseudosubstrates of the sarcoplasmic Ca2+-ATPase as tools to study the coupling between substrate hydrolysis and Ca2+ transport. J Biol Chem. 1979 Apr 10;254(7):2302–2307. [PubMed] [Google Scholar]
- Sanders D., Hansen U. P., Gradmann D., Slayman C. L. Generalized kinetic analysis of ion-driven cotransport systems: a unified interpretation of selective ionic effects on Michaelis parameters. J Membr Biol. 1984;77(2):123–152. doi: 10.1007/BF01925862. [DOI] [PubMed] [Google Scholar]
- Takisawa H., Makinose M. Occlusion of calcium in the ADP-sensitive phosphoenzyme of the adenosine triphosphatase of sarcoplasmic reticulum. J Biol Chem. 1983 Mar 10;258(5):2986–2992. [PubMed] [Google Scholar]
- Tanford C. Equilibrium state of ATP-driven ion pumps in relation to physiological ion concentration gradients. J Gen Physiol. 1981 Feb;77(2):223–229. doi: 10.1085/jgp.77.2.223. [DOI] [PMC free article] [PubMed] [Google Scholar]
- Tanford C., Reynolds J. A., Johnson E. A. Thermodynamic and kinetic cooperativity in ligand binding to multiple sites on a protein: Ca2+ activation of an ATP-driven Ca pump. Proc Natl Acad Sci U S A. 1985 Jul;82(14):4688–4692. doi: 10.1073/pnas.82.14.4688. [DOI] [PMC free article] [PubMed] [Google Scholar]
- de Meis L., Vianna A. L. Energy interconversion by the Ca2+-dependent ATPase of the sarcoplasmic reticulum. Annu Rev Biochem. 1979;48:275–292. doi: 10.1146/annurev.bi.48.070179.001423. [DOI] [PubMed] [Google Scholar]