Abstract
Antifreeze glycoproteins (AFGPs), found in the blood of polar fish at concentrations as high as 35 g/liter, are known to prevent ice crystal growth and depress the freezing temperature of the blood. Previously, Rubinsky et al. [Rubinsky, B., Mattioli, M., Arav, A., Barboni, B. & Fletcher, G. L. (1992) Am. J. Physiol. 262, R542-R545] provided evidence that AFGPs block ion fluxes across membranes during cooling, an effect that they ascribed to interactions with ion channels. We investigated the effects of AFGPs on the leakage of a trapped marker from liposomes during chilling. As these liposomes are cooled through the transition temperature, they leak approximately 50% of their contents. Addition of less than 1 mg/ml of AFGP prevents up to 100% of this leakage, both during chilling and warming through the phase transition. This is a general effect that we show here applies to liposomes composed of phospholipids with transition temperatures ranging from 12 degrees C to 41 degrees C. Because these results were obtained with liposomes composed of phospholipids alone, we conclude that the stabilizing effects of AFGPs on intact cells during chilling reported by Rubinsky et al. may be due to a nonspecific effect on the lipid components of native membranes. There are other proteins that prevent leakage, but only under specialized conditions. For instance, antifreeze proteins, bovine serum albumin, and ovomucoid all either have no effect or actually induce leakage. Following precipitation with acetone, all three proteins inhibited leakage, although not to the extent seen with AFGPs. Alternatively, there are proteins such as ovotransferrin that have no effect on leakage, either before or after acetone precipitation.
Full text
PDF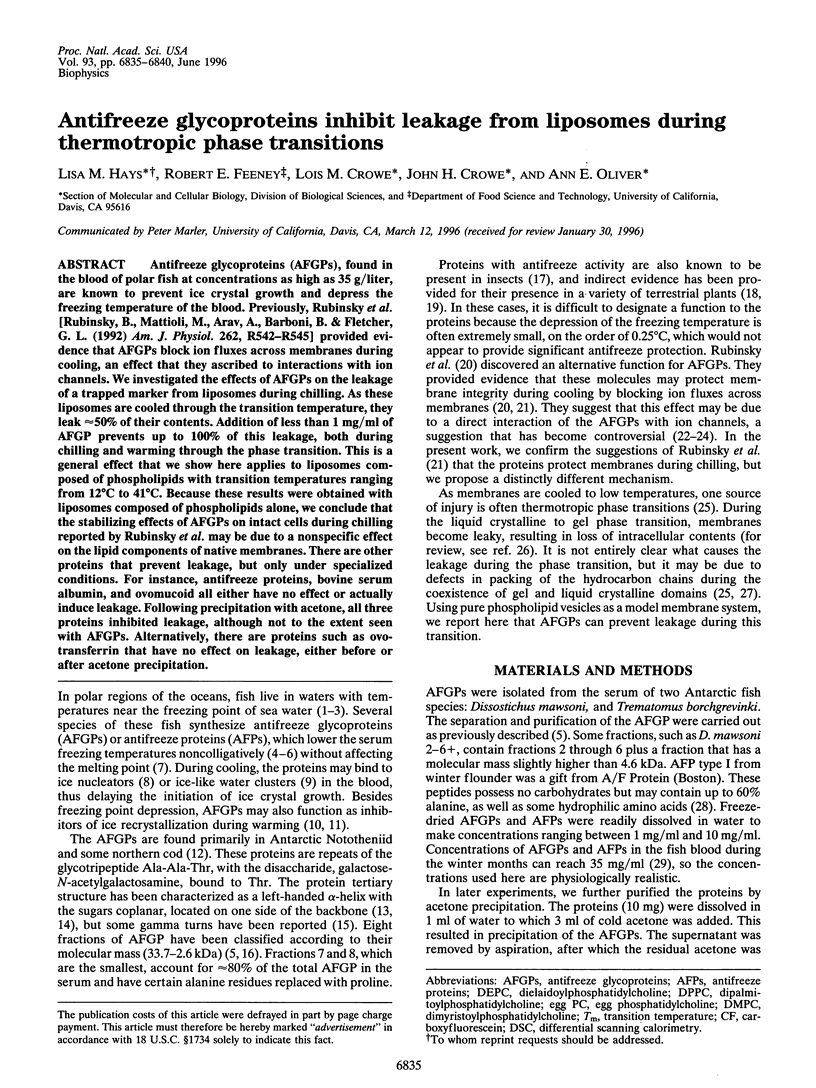
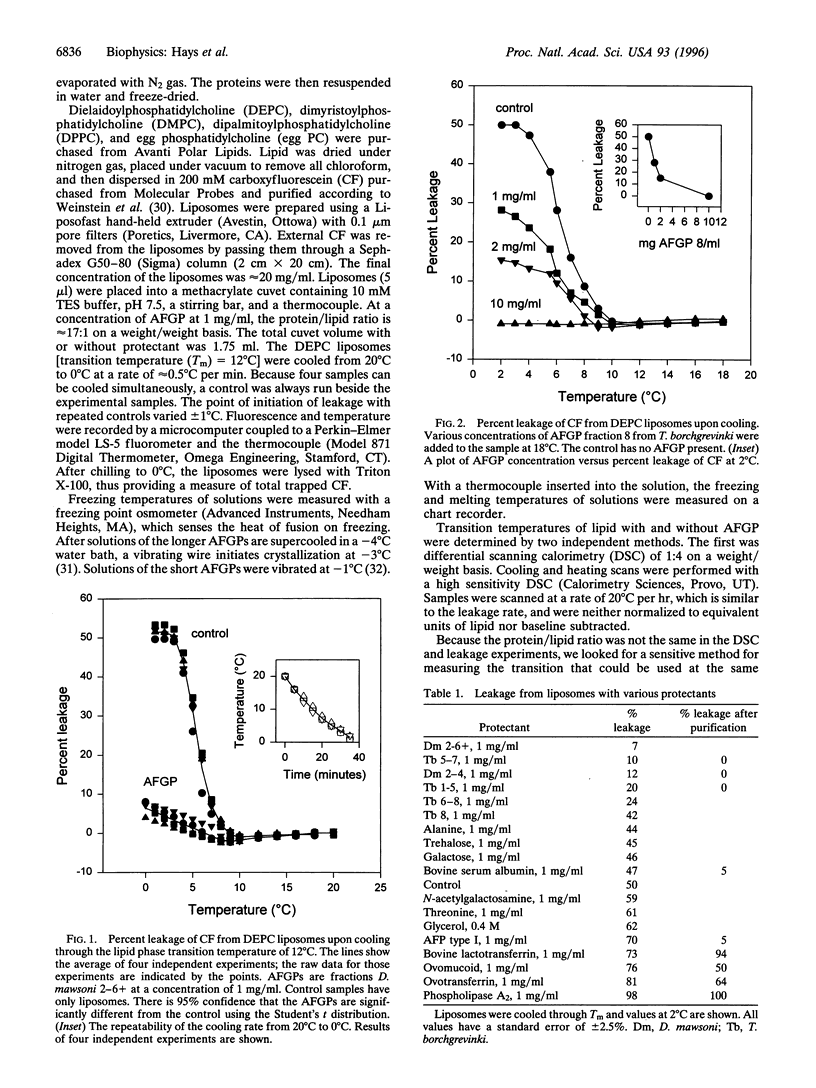
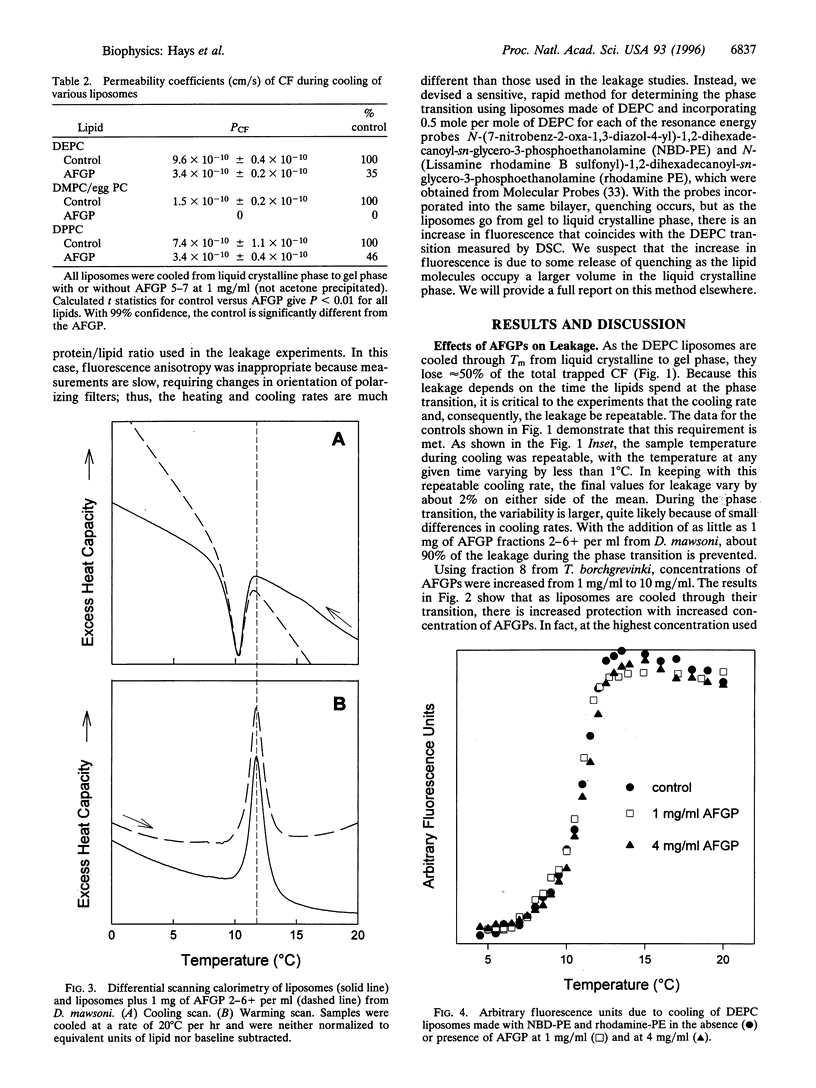
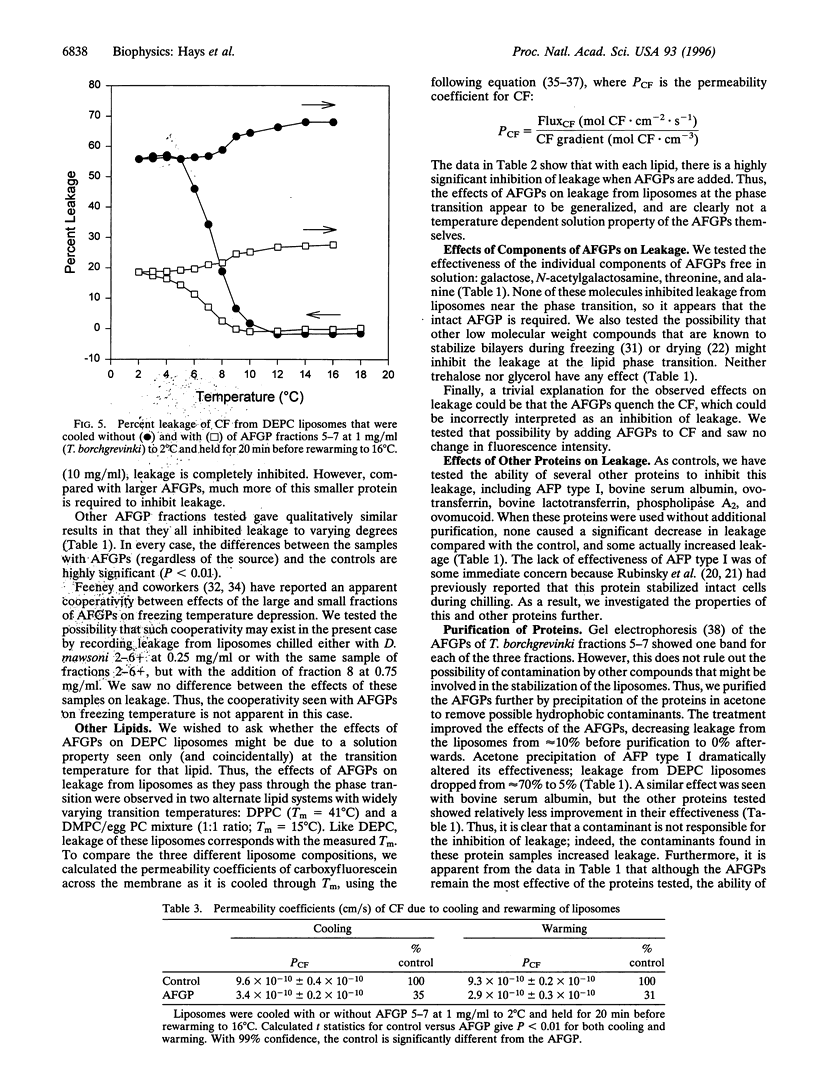
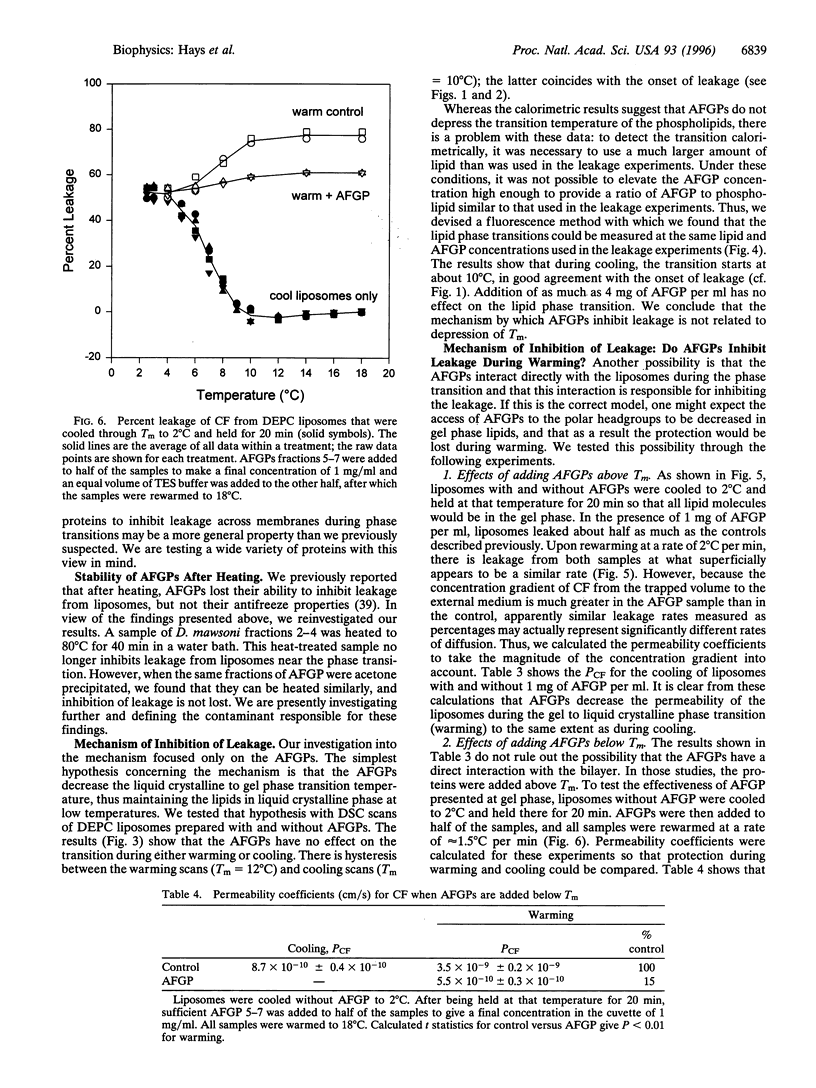
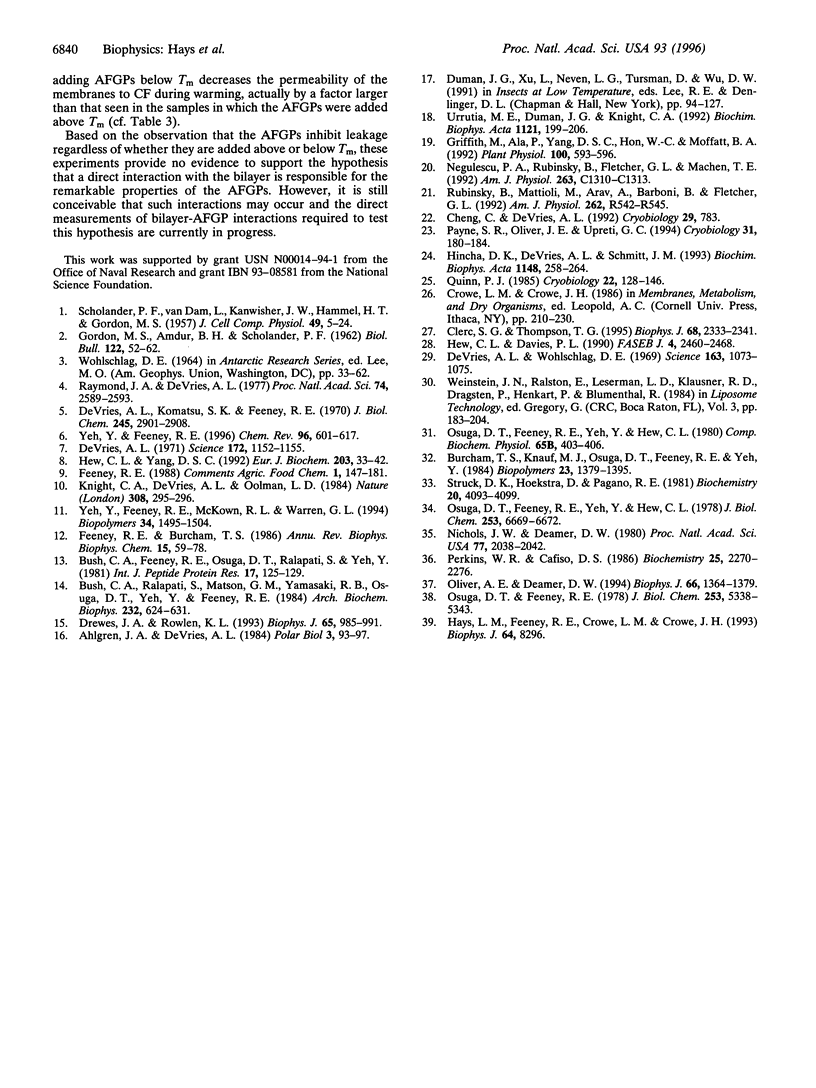
Selected References
These references are in PubMed. This may not be the complete list of references from this article.
- Burcham T. S., Knauf M. J., Osuga D. T., Feeney R. E., Yeh Y. Antifreeze glycoproteins: influence of polymer length and ice crystal habit on activity. Biopolymers. 1984 Jul;23(7):1379–1395. doi: 10.1002/bip.360230720. [DOI] [PubMed] [Google Scholar]
- Bush C. A., Feeney R. E., Osuga D. T., Ralapati S., Yeh Y. Antifreeze glycoprotein. Conformational model based on vacuum ultraviolet circular dichroism data. Int J Pept Protein Res. 1981 Jan;17(1):125–129. doi: 10.1111/j.1399-3011.1981.tb01975.x. [DOI] [PubMed] [Google Scholar]
- Bush C. A., Ralapati S., Matson G. M., Yamasaki R. B., Osuga D. T., Yeh Y., Feeney R. E. Conformation of the antifreeze glycoprotein of polar fish. Arch Biochem Biophys. 1984 Aug 1;232(2):624–631. doi: 10.1016/0003-9861(84)90582-4. [DOI] [PubMed] [Google Scholar]
- Clerc S. G., Thompson T. E. Permeability of dimyristoyl phosphatidylcholine/dipalmitoyl phosphatidylcholine bilayer membranes with coexisting gel and liquid-crystalline phases. Biophys J. 1995 Jun;68(6):2333–2341. doi: 10.1016/S0006-3495(95)80415-7. [DOI] [PMC free article] [PubMed] [Google Scholar]
- Davies P. L., Hew C. L. Biochemistry of fish antifreeze proteins. FASEB J. 1990 May;4(8):2460–2468. doi: 10.1096/fasebj.4.8.2185972. [DOI] [PubMed] [Google Scholar]
- DeVries A. L. Glycoproteins as biological antifreeze agents in antarctic fishes. Science. 1971 Jun 11;172(3988):1152–1155. doi: 10.1126/science.172.3988.1152. [DOI] [PubMed] [Google Scholar]
- DeVries A. L., Komatsu S. K., Feeney R. E. Chemical and physical properties of freezing point-depressing glycoproteins from Antarctic fishes. J Biol Chem. 1970 Jun 10;245(11):2901–2908. [PubMed] [Google Scholar]
- DeVries A. L., Wohlschlag D. E. Freezing resistance in some Antarctic fishes. Science. 1969 Mar 7;163(3871):1073–1075. doi: 10.1126/science.163.3871.1073. [DOI] [PubMed] [Google Scholar]
- Drewes J. A., Rowlen K. L. Evidence for a gamma-turn motif in antifreeze glycopeptides. Biophys J. 1993 Sep;65(3):985–991. doi: 10.1016/S0006-3495(93)81167-6. [DOI] [PMC free article] [PubMed] [Google Scholar]
- Feeney R. E., Burcham T. S., Yeh Y. Antifreeze glycoproteins from polar fish blood. Annu Rev Biophys Biophys Chem. 1986;15:59–78. doi: 10.1146/annurev.bb.15.060186.000423. [DOI] [PubMed] [Google Scholar]
- Griffith M., Ala P., Yang D. S., Hon W. C., Moffatt B. A. Antifreeze protein produced endogenously in winter rye leaves. Plant Physiol. 1992 Oct;100(2):593–596. doi: 10.1104/pp.100.2.593. [DOI] [PMC free article] [PubMed] [Google Scholar]
- Hew C. L., Yang D. S. Protein interaction with ice. Eur J Biochem. 1992 Jan 15;203(1-2):33–42. doi: 10.1111/j.1432-1033.1992.tb19824.x. [DOI] [PubMed] [Google Scholar]
- Hincha D. K., DeVries A. L., Schmitt J. M. Cryotoxicity of antifreeze proteins and glycoproteins to spinach thylakoid membranes--comparison with cryotoxic sugar acids. Biochim Biophys Acta. 1993 Mar 14;1146(2):258–264. doi: 10.1016/0005-2736(93)90364-6. [DOI] [PubMed] [Google Scholar]
- Knight C. A., DeVries A. L., Oolman L. D. Fish antifreeze protein and the freezing and recrystallization of ice. Nature. 1984 Mar 15;308(5956):295–296. doi: 10.1038/308295a0. [DOI] [PubMed] [Google Scholar]
- Negulescu P. A., Rubinsky B., Fletcher G. L., Machen T. E. Fish antifreeze proteins block Ca entry into rabbit parietal cells. Am J Physiol. 1992 Dec;263(6 Pt 1):C1310–C1313. doi: 10.1152/ajpcell.1992.263.6.C1310. [DOI] [PubMed] [Google Scholar]
- Nichols J. W., Deamer D. W. Net proton-hydroxyl permeability of large unilamellar liposomes measured by an acid-base titration technique. Proc Natl Acad Sci U S A. 1980 Apr;77(4):2038–2042. doi: 10.1073/pnas.77.4.2038. [DOI] [PMC free article] [PubMed] [Google Scholar]
- Oliver A. E., Deamer D. W. Alpha-helical hydrophobic polypeptides form proton-selective channels in lipid bilayers. Biophys J. 1994 May;66(5):1364–1379. doi: 10.1016/S0006-3495(94)80927-0. [DOI] [PMC free article] [PubMed] [Google Scholar]
- Osuga D. T., Feeney R. E. Antifreeze glycoproteins from Arctic fish. J Biol Chem. 1978 Aug 10;253(15):5338–5343. [PubMed] [Google Scholar]
- Osuga D. T., Ward F. C., Yeh Y., Feeney R. E. Cooperative functioning between antifreeze glycoproteins. J Biol Chem. 1978 Oct 10;253(19):6669–6672. [PubMed] [Google Scholar]
- Payne S. R., Oliver J. E., Upreti G. C. Effect of antifreeze proteins on the motility of ram spermatozoa. Cryobiology. 1994 Apr;31(2):180–184. doi: 10.1006/cryo.1994.1021. [DOI] [PubMed] [Google Scholar]
- Perkins W. R., Cafiso D. S. An electrical and structural characterization of H+/OH- currents in phospholipid vesicles. Biochemistry. 1986 Apr 22;25(8):2270–2276. doi: 10.1021/bi00356a063. [DOI] [PubMed] [Google Scholar]
- Quinn P. J. A lipid-phase separation model of low-temperature damage to biological membranes. Cryobiology. 1985 Apr;22(2):128–146. doi: 10.1016/0011-2240(85)90167-1. [DOI] [PubMed] [Google Scholar]
- Raymond J. A., DeVries A. L. Adsorption inhibition as a mechanism of freezing resistance in polar fishes. Proc Natl Acad Sci U S A. 1977 Jun;74(6):2589–2593. doi: 10.1073/pnas.74.6.2589. [DOI] [PMC free article] [PubMed] [Google Scholar]
- Rubinsky B., Mattioli M., Arav A., Barboni B., Fletcher G. L. Inhibition of Ca2+ and K+ currents by "antifreeze" proteins. Am J Physiol. 1992 Mar;262(3 Pt 2):R542–R545. doi: 10.1152/ajpregu.1992.262.3.R542. [DOI] [PubMed] [Google Scholar]
- Struck D. K., Hoekstra D., Pagano R. E. Use of resonance energy transfer to monitor membrane fusion. Biochemistry. 1981 Jul 7;20(14):4093–4099. doi: 10.1021/bi00517a023. [DOI] [PubMed] [Google Scholar]
- Urrutia M. E., Duman J. G., Knight C. A. Plant thermal hysteresis proteins. Biochim Biophys Acta. 1992 May 22;1121(1-2):199–206. doi: 10.1016/0167-4838(92)90355-h. [DOI] [PubMed] [Google Scholar]
- Yeh Yin, Feeney Robert E. Antifreeze Proteins: Structures and Mechanisms of Function. Chem Rev. 1996 Mar 28;96(2):601–618. doi: 10.1021/cr950260c. [DOI] [PubMed] [Google Scholar]