Abstract
The validity of macroscopic models for calculations of electrostatic energies in proteins is examined. The Tanford-Kirkwood (TK) model is extended to include the self energy of the ionized groups. It is shown that ionized groups cannot exist inside nonpolar regions of proteins and argued that the experimental finding of ions inside proteins proves that the corresponding local environment is polar. The modified TK model (MTK model), which adjusts charge-charge interactions by the corresponding solvent accessibilities, is found to be inconsistent with the TK model, on which it is based. The MTK model corresponds to a polar interior whereas the TK model assumes a nonpolar interior. It is shown that models that assume a high dielectric constant for proteins give reasonable results for interactions between charged groups at equilibrium. It is then explained why, in contradiction to common belief, protein interiors are polar around charged groups. It is argued that in focusing on charge-charge interactions one overlooks the key contribution of the protein dipoles in determining the self energy of charges in the interior of proteins.
Full text
PDF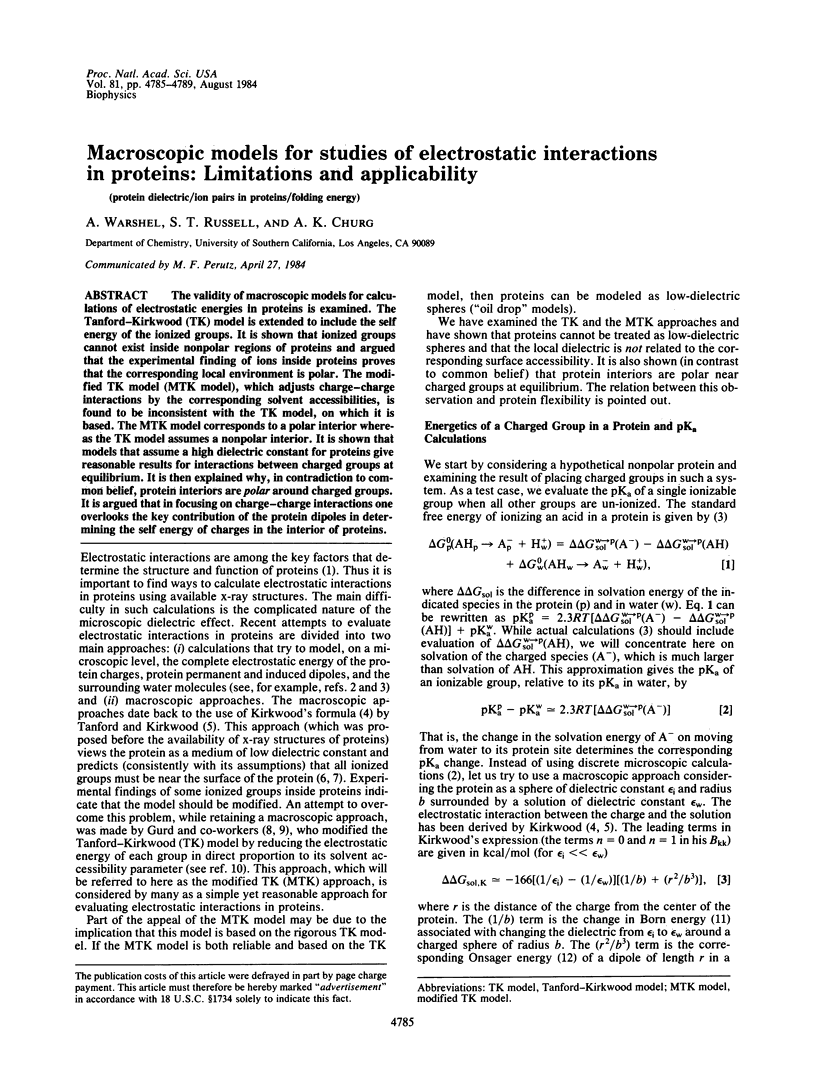
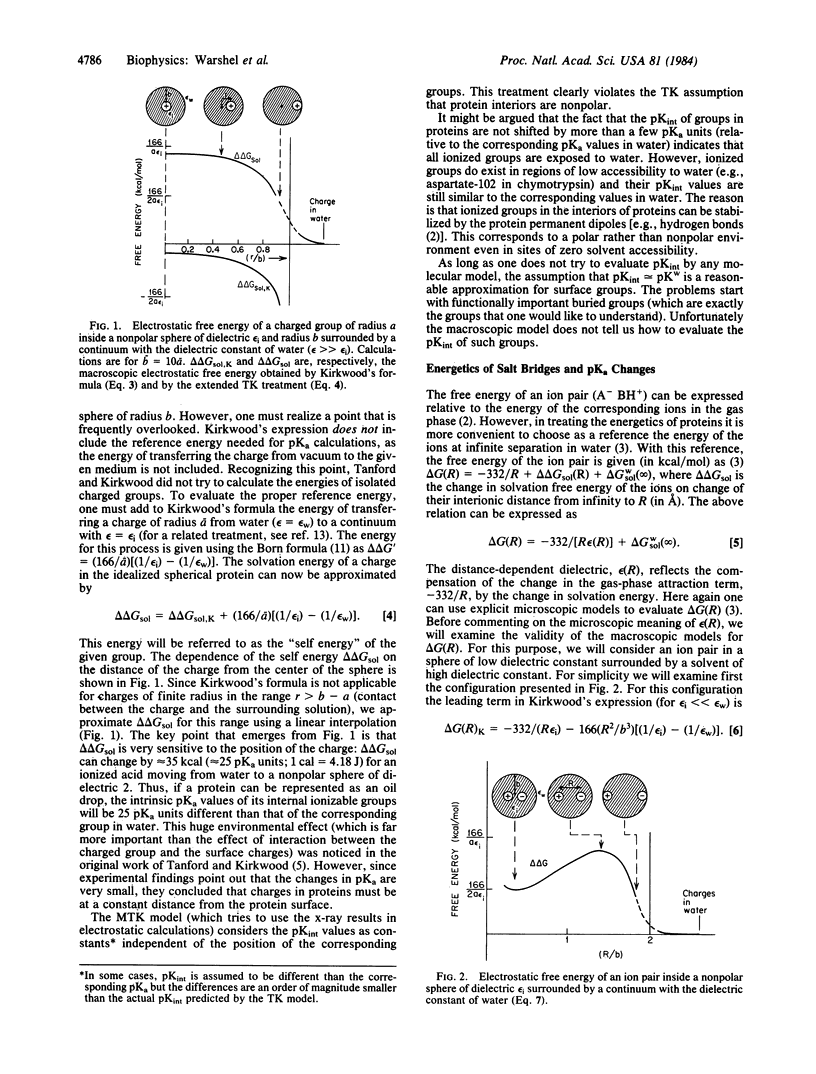
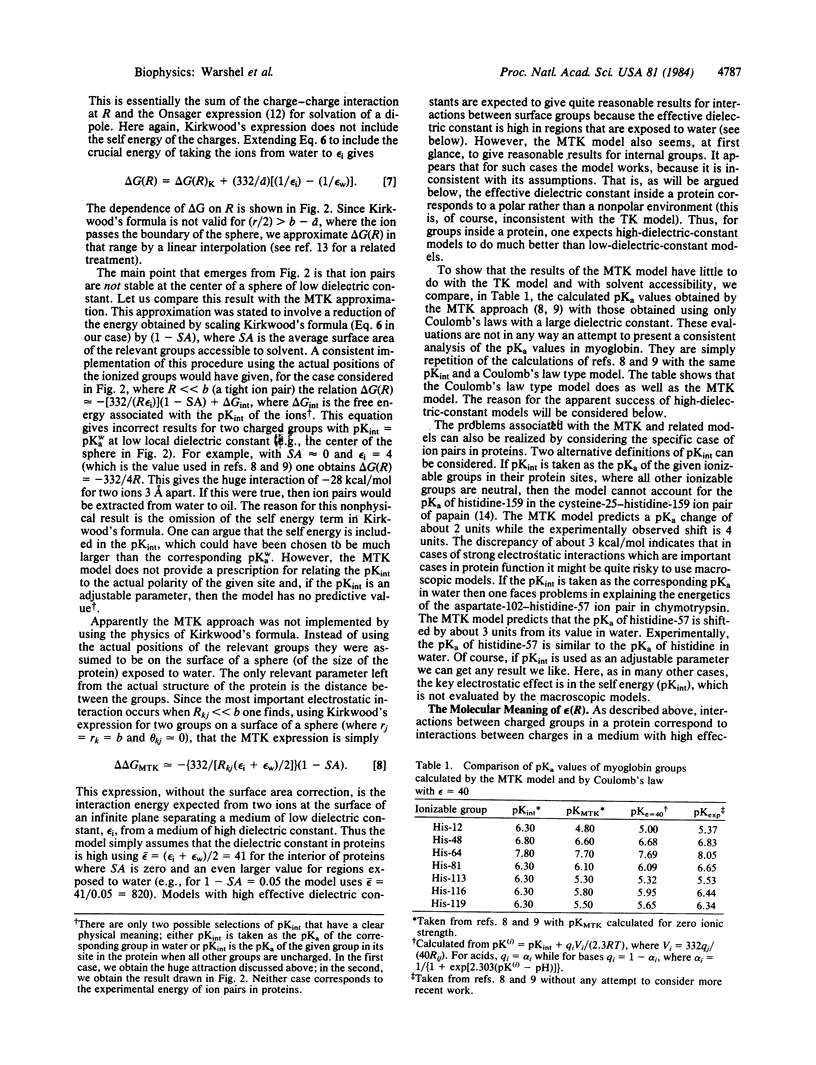
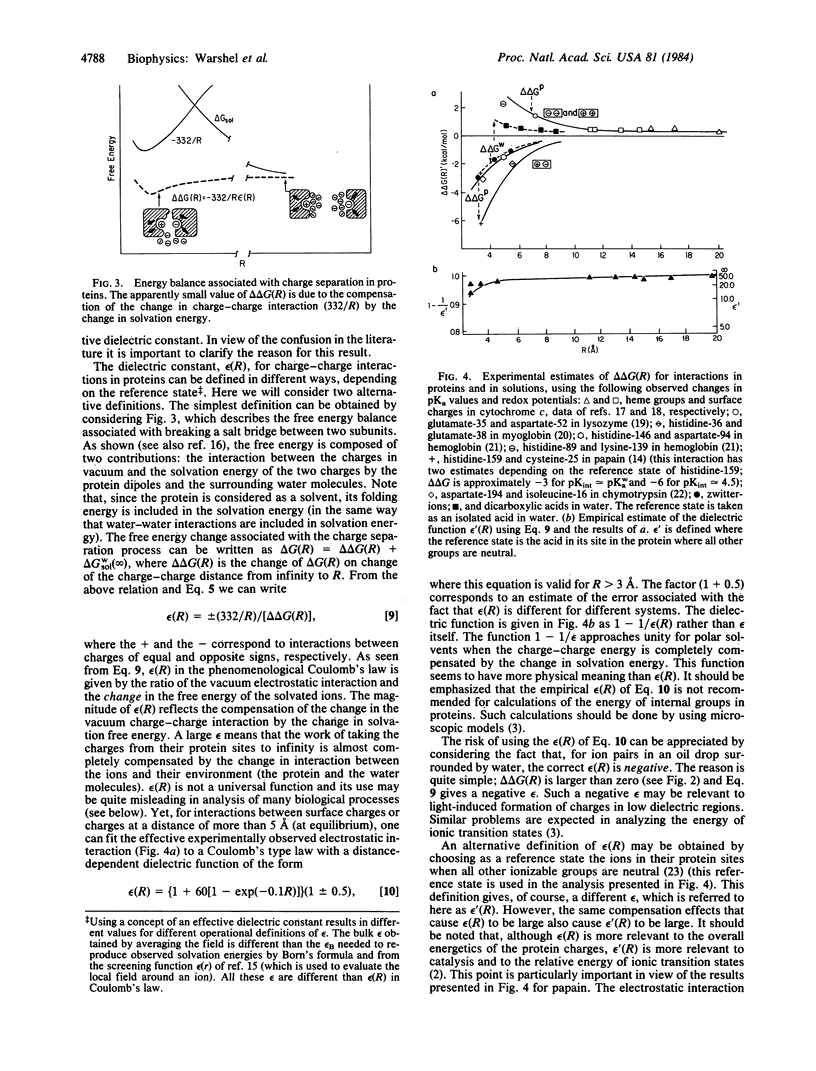
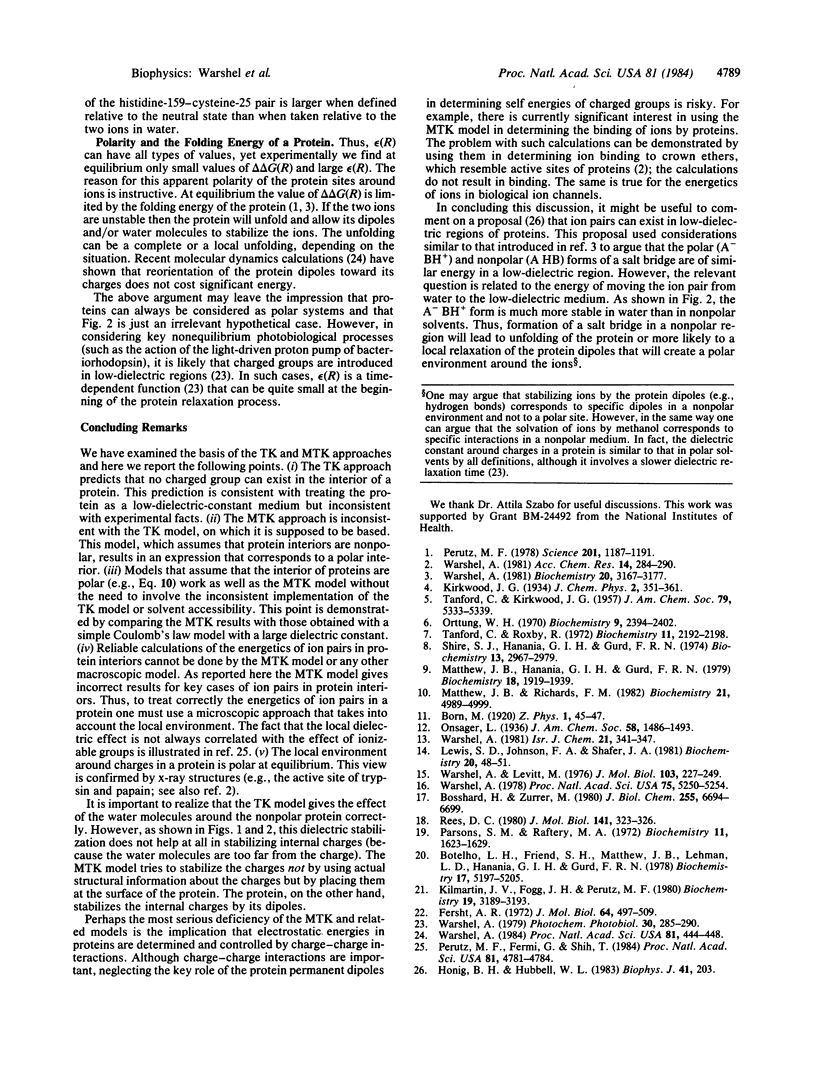
Selected References
These references are in PubMed. This may not be the complete list of references from this article.
- Bosshard H. R., Zürrer M. The conformation of cytochrome c in solution. Localization of a conformational difference between ferri- and ferrocytochrome c on the surface of the molecule. J Biol Chem. 1980 Jul 25;255(14):6694–6699. [PubMed] [Google Scholar]
- Botelho L. H., Friend S. H., Matthew J. B., Lehman L. D., Hanania G. I., Gurd F. R. Proton nuclear magnetic resonance study of histidine ionizations in myoglobins of various species. Comparison of observed and computed pK values. Biochemistry. 1978 Nov 28;17(24):5197–5205. doi: 10.1021/bi00617a020. [DOI] [PubMed] [Google Scholar]
- Fersht A. R. Conformational equilibria in -and -chymotrypsin. The energetics and importance of the salt bridge. J Mol Biol. 1972 Mar 14;64(2):497–509. doi: 10.1016/0022-2836(72)90513-x. [DOI] [PubMed] [Google Scholar]
- Kilmartin J. V., Fogg J. H., Perutz M. F. Role of C-terminal histidine in the alkaline Bohr effect of human hemoglobin. Biochemistry. 1980 Jul 8;19(14):3189–3183. doi: 10.1021/bi00555a013. [DOI] [PubMed] [Google Scholar]
- Lewis S. D., Johnson F. A., Shafer J. A. Effect of cysteine-25 on the ionization of histidine-159 in papain as determined by proton nuclear magnetic resonance spectroscopy. Evidence for a his-159--Cys-25 ion pair and its possible role in catalysis. Biochemistry. 1981 Jan 6;20(1):48–51. doi: 10.1021/bi00504a009. [DOI] [PubMed] [Google Scholar]
- Matthew J. B., Hanania G. I., Gurd F. R. Electrostatic effects in hemoglobin: hydrogen ion equilibria in human deoxy- and oxyhemoglobin A. Biochemistry. 1979 May 15;18(10):1919–1928. doi: 10.1021/bi00577a011. [DOI] [PubMed] [Google Scholar]
- Matthew J. B., Richards F. M. Anion binding and pH-dependent electrostatic effects in ribonuclease. Biochemistry. 1982 Sep 28;21(20):4989–4999. doi: 10.1021/bi00263a024. [DOI] [PubMed] [Google Scholar]
- Orttung W. H. Proton binding and dipole moment of hemoglobin. Refined calculations. Biochemistry. 1970 Jun 9;9(12):2394–2402. doi: 10.1021/bi00814a002. [DOI] [PubMed] [Google Scholar]
- Parsons S. M., Raftery M. A. Ionization behavior of the catalytic carboxyls of lysozyme. Effects of ionic strength. Biochemistry. 1972 Apr 25;11(9):1623–1629. doi: 10.1021/bi00759a013. [DOI] [PubMed] [Google Scholar]
- Perutz M. F. Electrostatic effects in proteins. Science. 1978 Sep 29;201(4362):1187–1191. doi: 10.1126/science.694508. [DOI] [PubMed] [Google Scholar]
- Perutz M. F., Fermi G., Shih T. B. Structure of deoxyhemoglobin Cowtown [His HC3(146) beta----Leu]: origin of the alkaline Bohr effect and electrostatic interactions in hemoglobin. Proc Natl Acad Sci U S A. 1984 Aug;81(15):4781–4784. doi: 10.1073/pnas.81.15.4781. [DOI] [PMC free article] [PubMed] [Google Scholar]
- Rees D. C. Experimental evaluation of the effective dielectric constant of proteins. J Mol Biol. 1980 Aug 15;141(3):323–326. doi: 10.1016/0022-2836(80)90184-9. [DOI] [PubMed] [Google Scholar]
- Shire S. J., Hanania G. I., Gurd F. R. Electrostatic effects in myoglobin. pH and ionic strength variations of ionization equilibria for individual groups in sperm whale ferrimyoglobin. Biochemistry. 1974 Jul 2;13(14):2974–2979. doi: 10.1021/bi00711a029. [DOI] [PubMed] [Google Scholar]
- Tanford C., Roxby R. Interpretation of protein titration curves. Application to lysozyme. Biochemistry. 1972 May 23;11(11):2192–2198. doi: 10.1021/bi00761a029. [DOI] [PubMed] [Google Scholar]
- Warshel A. Calculations of enzymatic reactions: calculations of pKa, proton transfer reactions, and general acid catalysis reactions in enzymes. Biochemistry. 1981 May 26;20(11):3167–3177. doi: 10.1021/bi00514a028. [DOI] [PubMed] [Google Scholar]
- Warshel A. Conversion of light energy to electrostatic energy in the proton pump of Halobacterium halobium. Photochem Photobiol. 1979 Aug;30(2):285–290. doi: 10.1111/j.1751-1097.1979.tb07148.x. [DOI] [PubMed] [Google Scholar]
- Warshel A. Dynamics of enzymatic reactions. Proc Natl Acad Sci U S A. 1984 Jan;81(2):444–448. doi: 10.1073/pnas.81.2.444. [DOI] [PMC free article] [PubMed] [Google Scholar]
- Warshel A. Energetics of enzyme catalysis. Proc Natl Acad Sci U S A. 1978 Nov;75(11):5250–5254. doi: 10.1073/pnas.75.11.5250. [DOI] [PMC free article] [PubMed] [Google Scholar]
- Warshel A., Levitt M. Theoretical studies of enzymic reactions: dielectric, electrostatic and steric stabilization of the carbonium ion in the reaction of lysozyme. J Mol Biol. 1976 May 15;103(2):227–249. doi: 10.1016/0022-2836(76)90311-9. [DOI] [PubMed] [Google Scholar]