Abstract
Soret-excited resonance Raman spectroscopy yields direct information regarding the iron-carbon bonding interactions in the cyanomet and carbonmonoxy complexes of hemoglobin III from Chironomus thummi thummi (CTT III) in solution. By isotope exchange in cyanide (13CN-, C15N-, and 13C15N-) and carbon monoxide (13CO, C18O, and 13C18O), we have assigned the Fe(III)-CN- stretching at 453 cm-1, the Fe(III)-C-N- bending at 412 cm-1, the Fe(II)-CO stretching at 500 cm-1, the Fe(II)-C-O bending at 574 cm-1, and the C-O stretching at 1960 cm-1. The resonance Raman data, in conjunction with those obtained from heme model complexes with well-known Fe-C bond distances, strongly suggest that the Fe(III)-CN- bond (approximately 1.91 A) is longer (hence weaker) than the Fe(II)-CO bond (approximately 1.80 A). This result disagrees with those of x-ray crystallographic studies [Steigemann, W. & Weber, E. (1979) J. Mol. Biol. 127, 309-338] in which the Fe-C bond lengths were reported as 2.2 A in cyanomet and 2.4 A in carbonmonoxy CTT III. Based on Badger's rule and normal mode calculations, the x-ray data would lead to the prediction of 279 cm-1 for the Fe(II)-CO stretching frequency in CTT III . CO, which was not observed. On the other hand, we estimate the Fe-CO bond as approximately equal to 1.82 A, which is very similar to the 1.80-A value in human Hb . CO crystals. Furthermore, we have used isotope shift data to estimate the Fe-C-O angle as 169 +/- 5 degrees, somewhat larger than the 161 degrees value found by Steigemann and Weber. We therefore conclude that there must be errors in the x-ray crystallographic refinement for the ligand geometry in carbonmonoxy and cyanomet CTT III.
Full text
PDF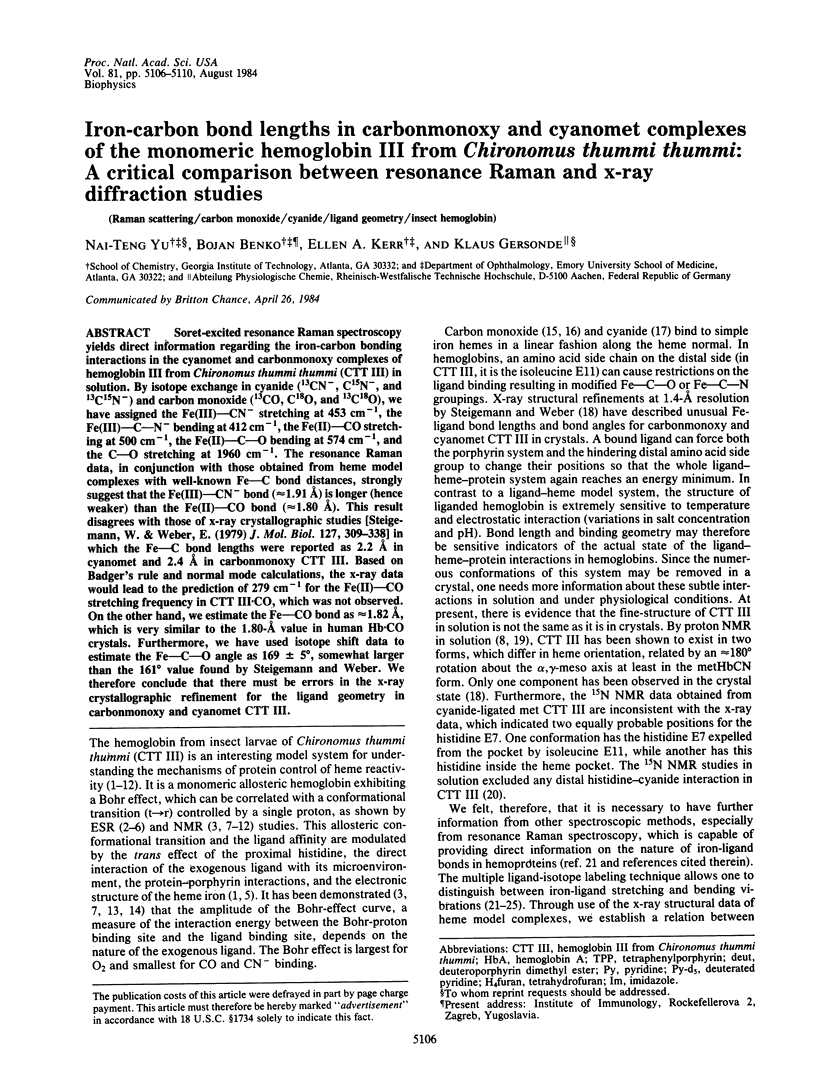
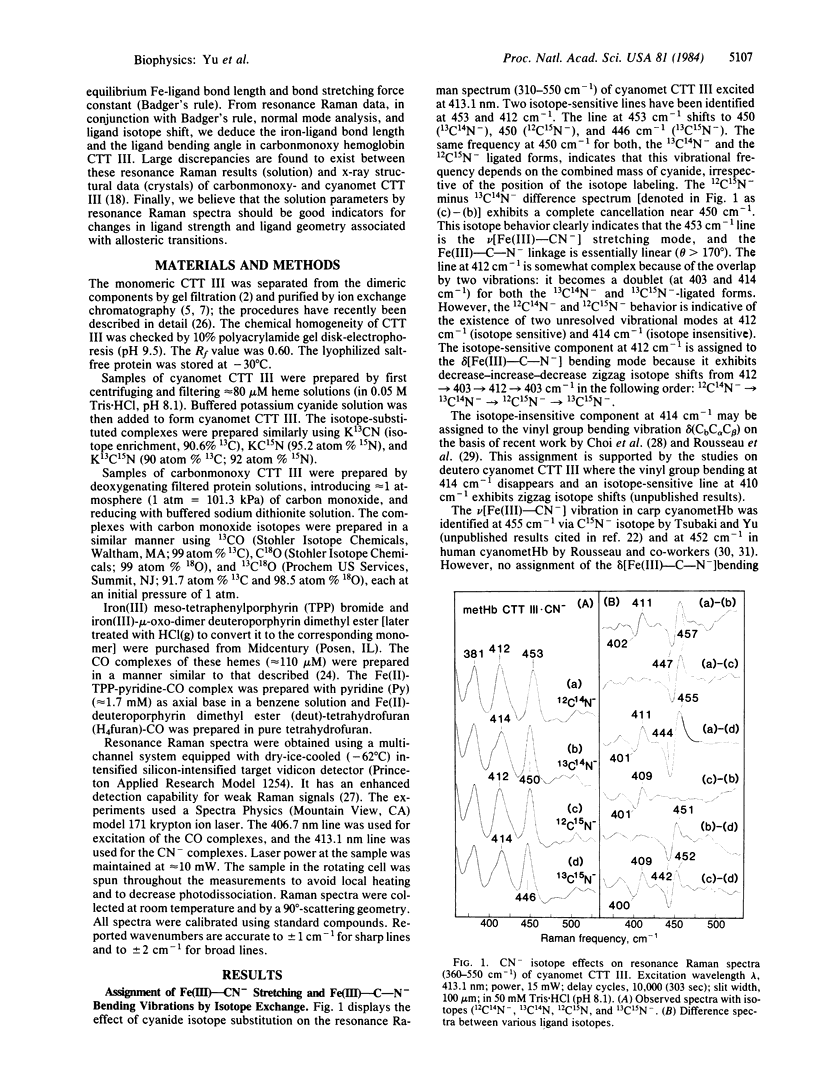
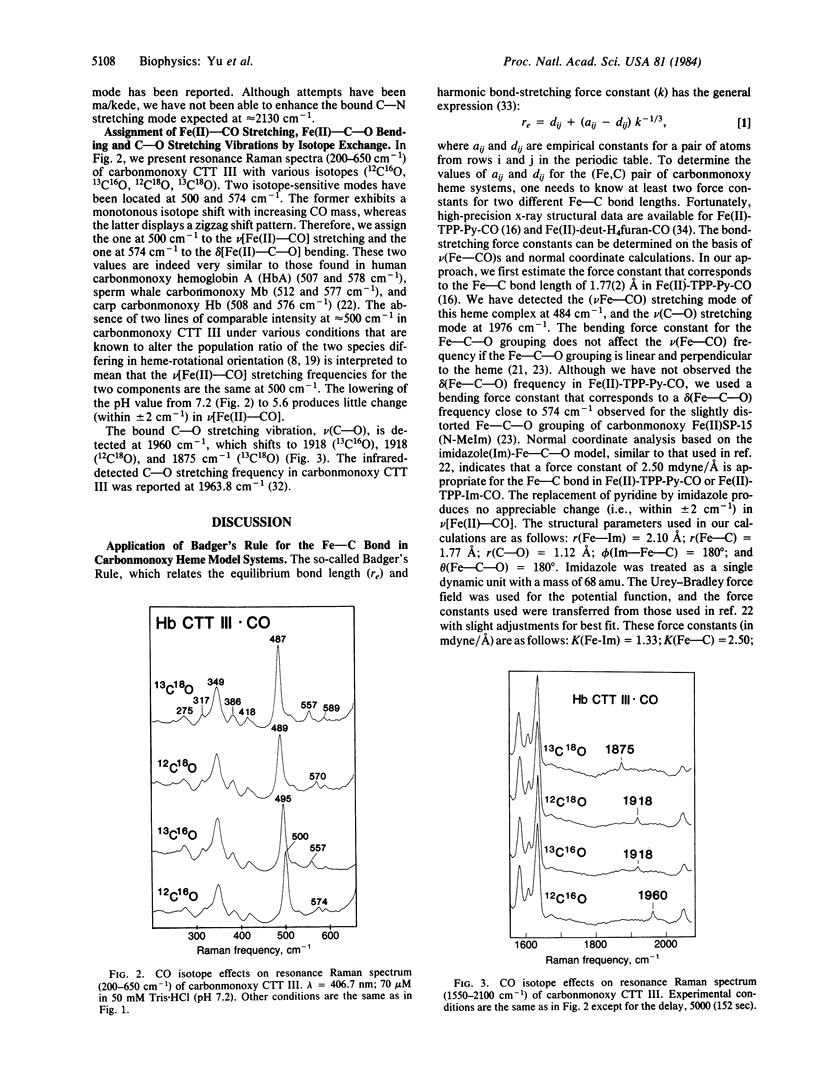
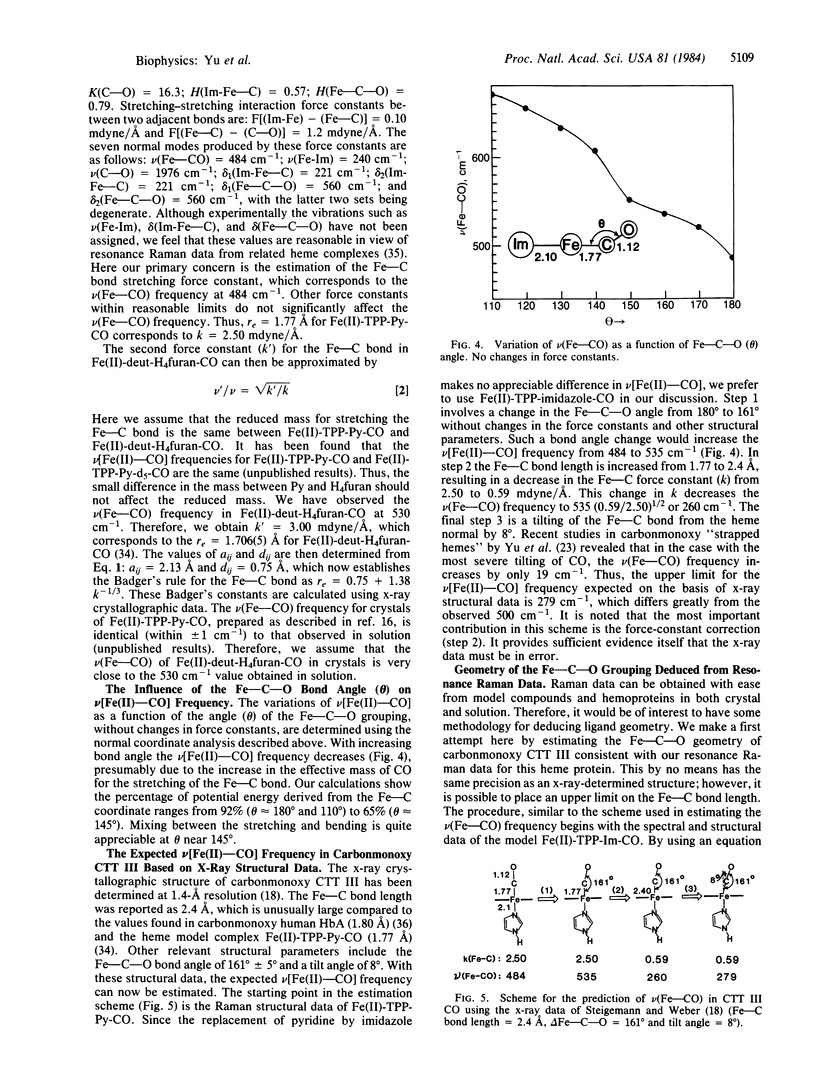
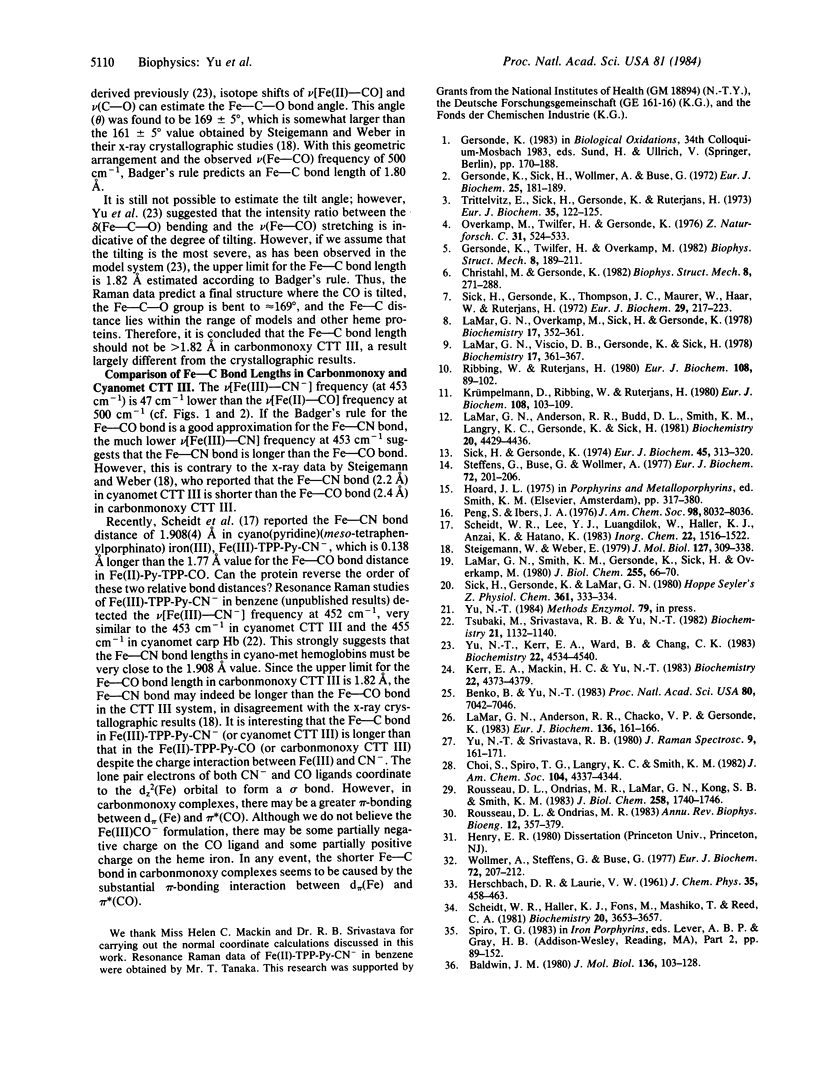
Selected References
These references are in PubMed. This may not be the complete list of references from this article.
- Baldwin J. M. The structure of human carbonmonoxy haemoglobin at 2.7 A resolution. J Mol Biol. 1980 Jan 15;136(2):103–128. doi: 10.1016/0022-2836(80)90308-3. [DOI] [PubMed] [Google Scholar]
- Benko B., Yu N. T. Resonance Raman studies of nitric oxide binding to ferric and ferrous hemoproteins: detection of Fe(III)--NO stretching, Fe(III)--N--O bending, and Fe(II)--N--O bending vibrations. Proc Natl Acad Sci U S A. 1983 Nov;80(22):7042–7046. doi: 10.1073/pnas.80.22.7042. [DOI] [PMC free article] [PubMed] [Google Scholar]
- Gersonde K., Sick H., Wollmer A., Buse G. Heterotropic allosterism of monomeric haemoglobins of Chironomus thummi thummi. Eur J Biochem. 1972 Jan 31;25(1):181–189. doi: 10.1111/j.1432-1033.1972.tb01683.x. [DOI] [PubMed] [Google Scholar]
- Gersonde K., Twilfer H., Overkamp M. Bohr-effect and pH-dependence of electron spin resonance spectra of a cobalt-substituted monomeric insect haemoglobin. Biophys Struct Mech. 1982;8(3):189–211. doi: 10.1007/BF00535459. [DOI] [PubMed] [Google Scholar]
- Kerr E. A., Mackin H. C., Yu N. T. Resonance Raman studies of carbon monoxide binding to iron "picket fence" porphyrin with unhindered and hindered axial bases. An inverse relationship between binding affinity and the strength of iron-carbon bond. Biochemistry. 1983 Sep 13;22(19):4373–4379. doi: 10.1021/bi00288a005. [DOI] [PubMed] [Google Scholar]
- Krümpelmann D., Ribbing W., Rüterjans H. Isomeric incorporation of the haem into monomeric haemoglobins of Chironomus thummi thummi 3. Comparative study of components, I, III and IV. Eur J Biochem. 1980;108(1):103–109. doi: 10.1111/j.1432-1033.1980.tb04700.x. [DOI] [PubMed] [Google Scholar]
- La Mar G. N., Anderson R. R., Budd D. L., Smith K. M., Langry K. C., Gersonde K., Sick H. Proton nuclear magnetic resonance investigation of the nature of solution conformational equilibria of monomeric insect deoxyhemoglobins. Biochemistry. 1981 Jul 21;20(15):4429–4436. doi: 10.1021/bi00518a030. [DOI] [PubMed] [Google Scholar]
- La Mar G. N., Anderson R. R., Chacko V. P., Gersonde K. High-resolution proton NMR as indicator of a silent mutation in the haem cavity of a monomeric allosteric haemoglobin. Eur J Biochem. 1983 Oct 17;136(1):161–166. doi: 10.1111/j.1432-1033.1983.tb07721.x. [DOI] [PubMed] [Google Scholar]
- La Mar G. N., Overkamp M., Sick H., Gersonde K. Proton nuclear magnetic resonance hyperfine shifts as indicators of tertiary structural changes accompanying the Bohr effect in monomeric insect hemoglobins. Biochemistry. 1978 Jan 24;17(2):352–361. doi: 10.1021/bi00595a025. [DOI] [PubMed] [Google Scholar]
- La Mar G. N., Smith K. M., Gersonde K., Sick H., Overkamp M. Proton nuclear nagnetic resonance characterization of heme disorder in monomeric insect hemoglobins. J Biol Chem. 1980 Jan 10;255(1):66–70. [PubMed] [Google Scholar]
- La Mar G. N., Viscio D. B., Gersonde K., Sick H. Proton nuclear magnetic resonance study of the rotational position and oscillatory mobility of vinyl groups in allosteric monomeric insect hemoglobins. Biochemistry. 1978 Jan 24;17(2):361–367. doi: 10.1021/bi00595a026. [DOI] [PubMed] [Google Scholar]
- Overkamp M., Twilfer H., Gersonde K. Conformation-controlled trans-effect of the proximal histidine in haemoglobins. An electron spin resonance study of monomeric nitrosyl-57Fe-haemoglobins. Z Naturforsch C. 1976 Sep-Oct;31(9-10):524–533. doi: 10.1515/znc-1976-9-1009. [DOI] [PubMed] [Google Scholar]
- Peng S. M., Ibers J. A. Stereochemistry of carbonylmetalloporphyrins. The structure of (pyridine)(carbonyl)(5, 10, 15, 20-tetraphenylprophinato)iron(II). J Am Chem Soc. 1976 Dec 8;98(25):8032–8036. doi: 10.1021/ja00441a025. [DOI] [PubMed] [Google Scholar]
- Ribbing W., Rüterjans H. Isomeric incorporation of the Haem into monomeric haemoglobins of Chironomus thummi thummi. 2. The Bohr effect of the component III explained on a molecular basis and functional differences between the two isomeric structures. Eur J Biochem. 1980;108(1):89–102. doi: 10.1111/j.1432-1033.1980.tb04699.x. [DOI] [PubMed] [Google Scholar]
- Rousseau D. L., Ondrias M. R., LaMar G. N., Kong S. B., Smith K. M. Resonance Raman spectra of the heme in leghemoglobin. Evidence for the absence of ruffling and the influence of the vinyl groups. J Biol Chem. 1983 Feb 10;258(3):1740–1746. [PubMed] [Google Scholar]
- Rousseau D. L., Ondrias M. R. Resonance Raman scattering studies of the quaternary structure transition in hemoglobin. Annu Rev Biophys Bioeng. 1983;12:357–380. doi: 10.1146/annurev.bb.12.060183.002041. [DOI] [PubMed] [Google Scholar]
- Scheidt W. R., Haller K. J., Fons M., Mashiko T., Reed C. A. A (carbonmonoxy)heme complex with a weak proximal bond. Molecular stereochemistry of carbonyl(deuteroporphinato)(tetrahydrofuran)iron(II). Biochemistry. 1981 Jun 9;20(12):3653–3657. doi: 10.1021/bi00515a054. [DOI] [PubMed] [Google Scholar]
- Sick H., Gersonde K. Ligand-specific Bohr effect in haemoglobins. Eur J Biochem. 1974 Jun 1;45(1):313–320. doi: 10.1111/j.1432-1033.1974.tb03556.x. [DOI] [PubMed] [Google Scholar]
- Sick H., Gersonde K., Thompson J. C., Maurer W., Haar W., Rüterjans H. The Bohr proton-binding site in a monomeric haemoglobin. A nuclear-magnetic-resonance study. Eur J Biochem. 1972 Sep 18;29(2):217–223. doi: 10.1111/j.1432-1033.1972.tb01978.x. [DOI] [PubMed] [Google Scholar]
- Steffens G., Buse G., Wollmer A. Ligand-dependent Bohr effect of Chrionomus hemoglobins. Eur J Biochem. 1977 Jan 3;72(1):201–206. doi: 10.1111/j.1432-1033.1977.tb11240.x. [DOI] [PubMed] [Google Scholar]
- Steigemann W., Weber E. Structure of erythrocruorin in different ligand states refined at 1.4 A resolution. J Mol Biol. 1979 Jan 25;127(3):309–338. doi: 10.1016/0022-2836(79)90332-2. [DOI] [PubMed] [Google Scholar]
- Trittelvitz E., Sick H., Gersonde K., Rüterjans H. Reduced Bohr effect in NO-ligated chironomus haemoglobin. Eur J Biochem. 1973 May;35(1):122–125. doi: 10.1111/j.1432-1033.1973.tb02817.x. [DOI] [PubMed] [Google Scholar]
- Tsubaki M., Srivastava R. B., Yu N. T. Resonance Raman investigation of carbon monoxide bonding in (carbon monoxy)hemoglobin and -myoglobin: detection of Fe-CO stretching and Fe-C-O bending vibrations and influence of the quaternary structure change. Biochemistry. 1982 Mar 16;21(6):1132–1140. doi: 10.1021/bi00535a004. [DOI] [PubMed] [Google Scholar]
- Wollmer A., Steffens G., Buse G. Inequivalent conformational response of Chironomus hemoglobins to ligation with O2 and CO. A circular-dichrosim and infrared-spectroscopic study. Eur J Biochem. 1977 Jan 3;72(1):207–212. doi: 10.1111/j.1432-1033.1977.tb11241.x. [DOI] [PubMed] [Google Scholar]
- Yu N. T., Kerr E. A., Ward B., Chang C. K. Resonance Raman detection of Fe-CO stretching and Fe-C-O bending vibrations in sterically hindered carbonmonoxy "strapped hemes". A structural probe of Fe-C-O distortion. Biochemistry. 1983 Sep 13;22(19):4534–4540. doi: 10.1021/bi00288a028. [DOI] [PubMed] [Google Scholar]