Abstract
Nitrogenase and nitrogenase reductase dissociate after each electron is transferred between them, as shown by the occurrence of a lag phase approximately as long as the average turnover time of nitrogenase before hydrogen evolution occurs. Because nitrogenase was present in the reaction mixture in large excess over nitrogenase reductase, the electrons donated by nitrogenase reductase must have been distributed randomly over all of the nitrogenase present. This is accomplished by nitrogenase reductase molecules associating randomly with nitrogenase molecules for each cycle of electrons transferred. The fact that ATP is hydrolyzed without a lag indicates both that electron transfer occurs during the lag and the ATP hydrolysis is coupled to electron transfer from nitrogenase reductase to nitrogenase and not to substrate reduction. The observations support the suggestion that it now is desirable to alter nomenclature to designate the MoFe protein as nitrogenase and the Fe protein as nitrogenase reductase.
Full text
PDF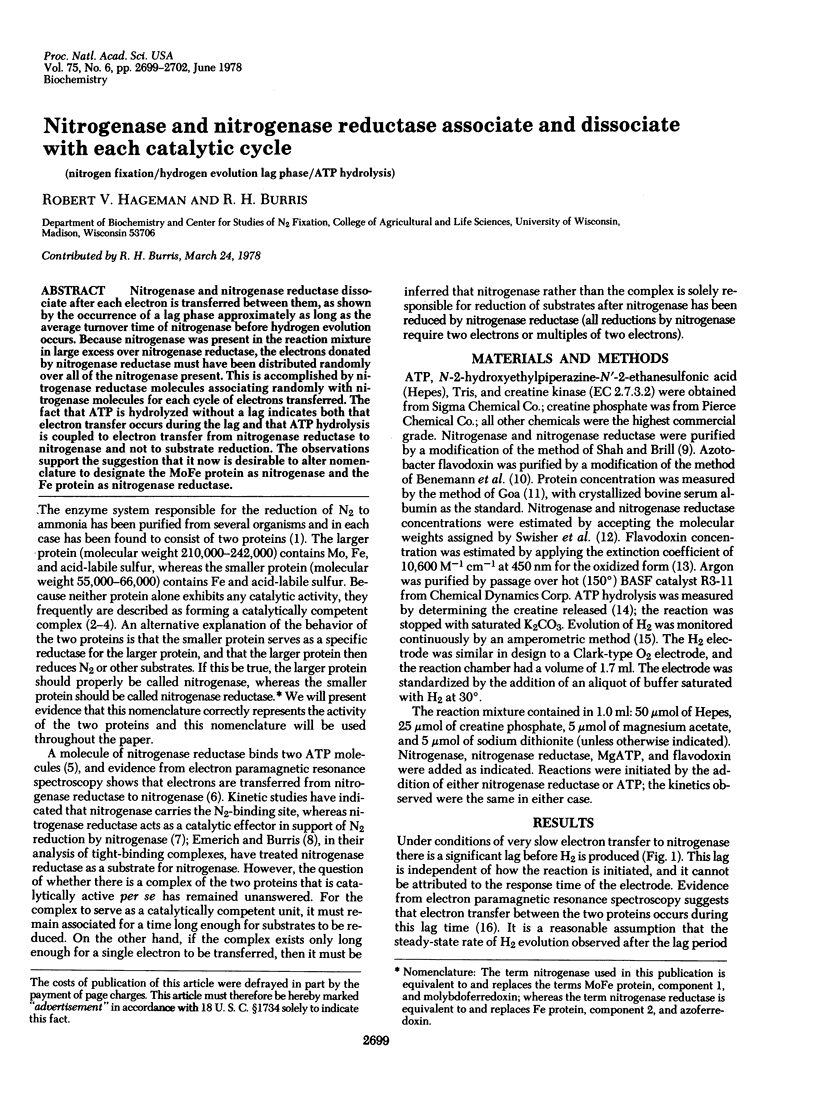
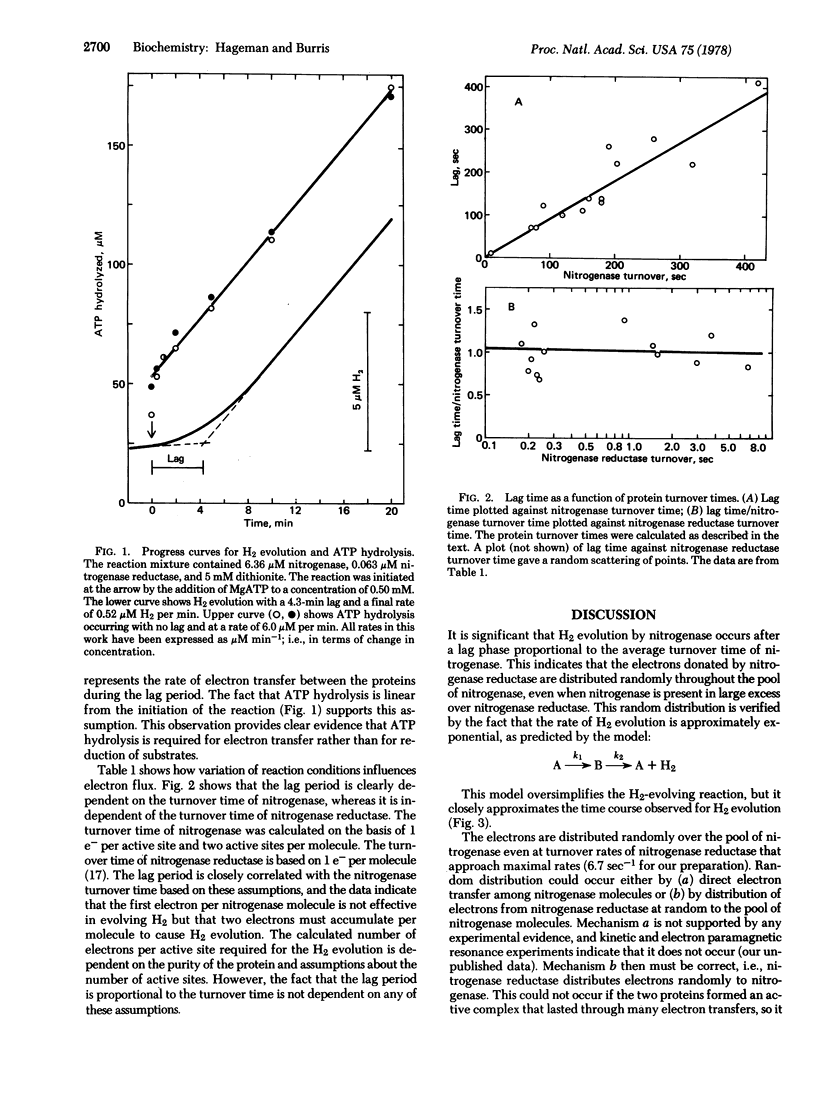
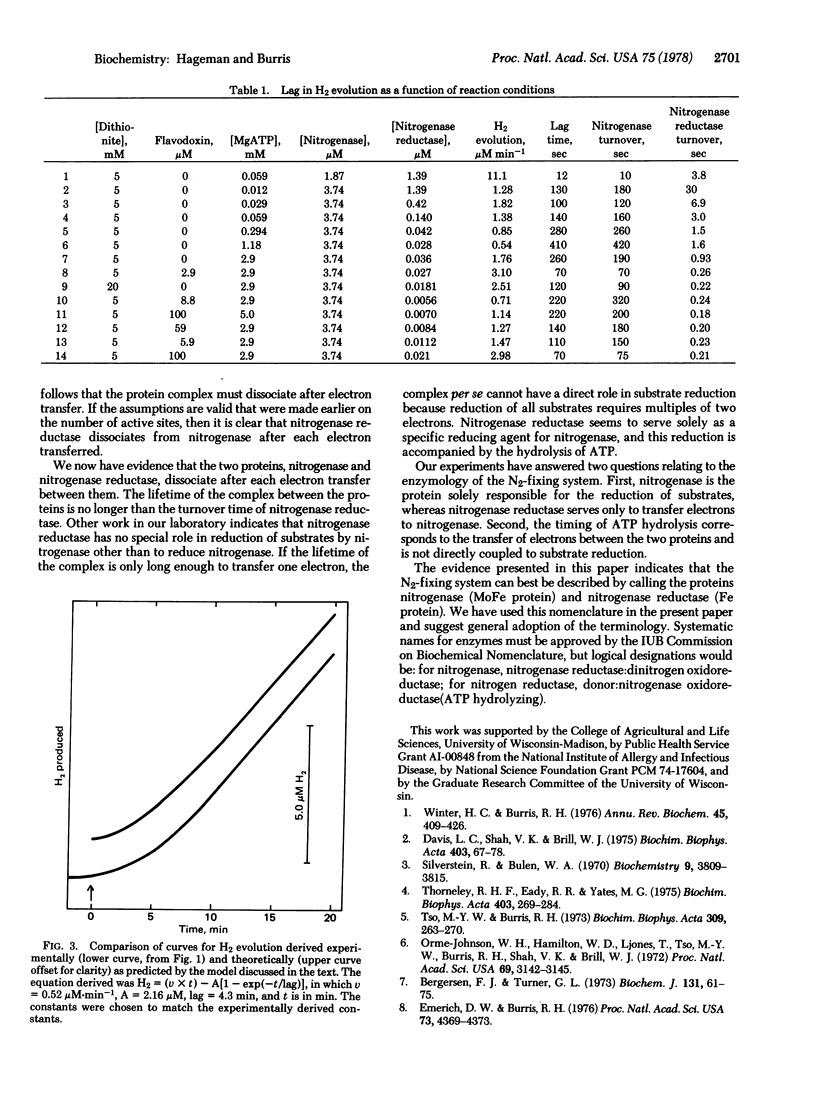
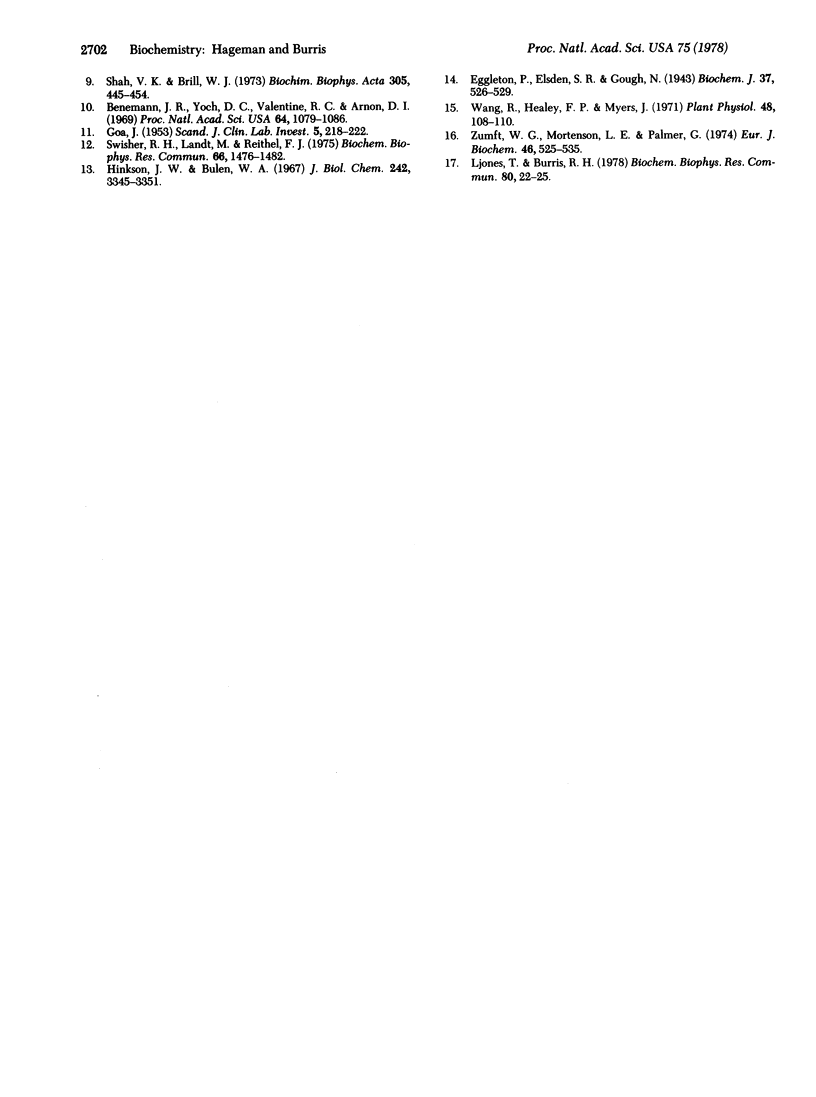
Selected References
These references are in PubMed. This may not be the complete list of references from this article.
- Benemann J. R., Yoch D. C., Valentine R. C., Arnon D. I. The electron transport system in nitrogen fixation by Azotobacter. I. Azotoflavin as an electron carrier. Proc Natl Acad Sci U S A. 1969 Nov;64(3):1079–1086. doi: 10.1073/pnas.64.3.1079. [DOI] [PMC free article] [PubMed] [Google Scholar]
- Bergersen F. J., Turner G. L. Kinetic studies of nitrogenase from soya-bean root-nodule bacteroids. Biochem J. 1973 Jan;131(1):61–75. doi: 10.1042/bj1310061. [DOI] [PMC free article] [PubMed] [Google Scholar]
- Davis L. C., Shah V. K., Brill W. J. Nitrogenase. VII. Effect of component ratio, ATP and H2 on the distribution of electrons to alternative substrates. Biochim Biophys Acta. 1975 Sep 22;403(1):67–78. doi: 10.1016/0005-2744(75)90009-1. [DOI] [PubMed] [Google Scholar]
- Eggleton P., Elsden S. R., Gough N. The estimation of creatine and of diacetyl. Biochem J. 1943;37(5):526–529. doi: 10.1042/bj0370526. [DOI] [PMC free article] [PubMed] [Google Scholar]
- Emerich D. W., Burris R. H. Interactions of heterologous nitrogenase components that generate catalytically inactive complexes. Proc Natl Acad Sci U S A. 1976 Dec;73(12):4369–4373. doi: 10.1073/pnas.73.12.4369. [DOI] [PMC free article] [PubMed] [Google Scholar]
- GOA J. A micro biuret method for protein determination; determination of total protein in cerebrospinal fluid. Scand J Clin Lab Invest. 1953;5(3):218–222. doi: 10.3109/00365515309094189. [DOI] [PubMed] [Google Scholar]
- Hinkson J. W., Bulen W. A. A free radical flavoprotein from Azotobacter. Isolation, crystallization, and properties. J Biol Chem. 1967 Jul 25;242(14):3345–3351. [PubMed] [Google Scholar]
- Ljones T., Burris R. H. Evidence for one-electron transfer by the Fe protein of nitrogenase. Biochem Biophys Res Commun. 1978 Jan 13;80(1):22–25. doi: 10.1016/0006-291x(78)91098-7. [DOI] [PubMed] [Google Scholar]
- Orme-Johnson W. H., Hamilton W. D., Jones T. L., Tso M. Y., Burris R. H., Shah V. K., Brill W. J. Electron paramagnetic resonance of nitrogenase and nitrogenase components from Clostridium pasteurianum W5 and Azotobacter vinelandii OP. Proc Natl Acad Sci U S A. 1972 Nov;69(11):3142–3145. doi: 10.1073/pnas.69.11.3142. [DOI] [PMC free article] [PubMed] [Google Scholar]
- Shah V. K., Brill W. J. Nitrogenase. IV. Simple method of purification to homogeneity of nitrogenase components from Azotobacter vinelandii. Biochim Biophys Acta. 1973 May 30;305(2):445–454. doi: 10.1016/0005-2728(73)90190-4. [DOI] [PubMed] [Google Scholar]
- Silverstein R., Bulen W. A. Kinetic studies of the nitrogense-catalyzed hydrogen volution and nitrogen reduction reactions. Biochemistry. 1970 Sep 15;9(19):3809–3815. doi: 10.1021/bi00821a021. [DOI] [PubMed] [Google Scholar]
- Swisher R. H., Landt M., Reithel F. J. Molecular weights of nitrogenase components from Azotobacter vinelandii. Biochem Biophys Res Commun. 1975 Oct 27;66(4):1476–1482. doi: 10.1016/0006-291x(75)90525-2. [DOI] [PubMed] [Google Scholar]
- Thorneley R. N., Eady R. R., Yates M. G. Nitrogenases of Klebsiella pneumoniae and Azotobacter chroococum. Complex formation between the component proteins. Biochim Biophys Acta. 1975 Oct 22;403(2):269–284. doi: 10.1016/0005-2744(75)90057-1. [DOI] [PubMed] [Google Scholar]
- Tso M. Y., Burris R. H. The binding of ATP and ADP by nitrogenase components from Clostridium pasteurianum. Biochim Biophys Acta. 1973 Jun 6;309(2):263–270. doi: 10.1016/0005-2744(73)90024-7. [DOI] [PubMed] [Google Scholar]
- Wang R., Healey F. P., Myers J. Amperometric measurement of hydrogen evolution in chlamydomonas. Plant Physiol. 1971 Jul;48(1):108–110. doi: 10.1104/pp.48.1.108. [DOI] [PMC free article] [PubMed] [Google Scholar]
- Winter H. C., Burris R. H. Nitrogenase. Annu Rev Biochem. 1976;45:409–426. doi: 10.1146/annurev.bi.45.070176.002205. [DOI] [PubMed] [Google Scholar]
- Zumft W. G., Mortenson L. E., Palmer G. Electron-paramagnetic-resonance studies on nitrogenase. Investigation of the oxidation-reduction behaviour of azoferredoxin and molybdoferredoxin with potentiometric and rapid-freeze techniques. Eur J Biochem. 1974 Aug 1;46(3):525–535. doi: 10.1111/j.1432-1033.1974.tb03646.x. [DOI] [PubMed] [Google Scholar]