Abstract
In order to verify more directly our earlier measurements showing that, on the average, close to four vectorial H+ are rejected per pair of electrons passing each of the three energy-conserving sites of the mitochondrial electron transport chain, direct tests of the H+/2e- ratio for sites 2 and 3 were carried out in the presence of permeant charge-compensating cations. Site 2 was examined by utilizing succinate as electron donor and ferricyanide as electron acceptor from mitochondrial cytochrome c; the directly measured H+/2e- ratio was close to 4. Energy-conserving site 3 was isolated for study with ferrocyanide or ascorbate plus tetramethylphenylenediamine as electron donors to cytochrome c and with oxygen as electron acceptor. The directly measured H+/2e- ratio for site 3 was close to 4. The H+/ATP ratio (number of vectorial H+ ejected per ATP hydrolyzed) was determined with a new method in which the steady-state rates of both H+ ejection and ATP hydrolysis were measured in the presence of K+ + valinomycin. The H+/ATP ratio was found to approach 3.0. A proton cycle for oxidative phosphorylation is proposed, in which four electrochemical H+ equivalents are ejected per pair of electrons passing each energy-conserving site; three of the H+ equivalents pass inward to derive ATP synthesis from ADP and phosphate and the fourth H+ is used to bring about the energy-requiring electrogenic expulsion of ATP4- in exchange for extramitochondrial ADP3-, via the H+/H2PO4- symporter.
Keywords: electron transport, ATP hydrolysis, oxidative phosphorylation, H+ transport
Full text
PDF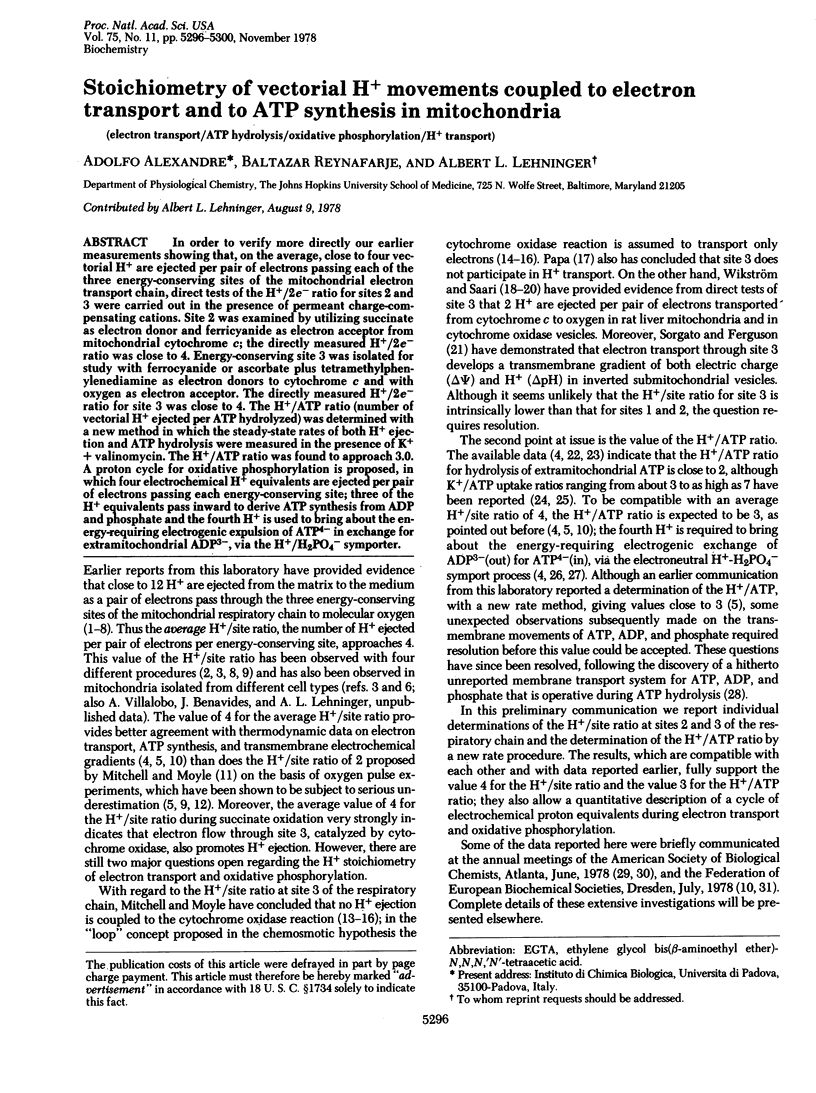
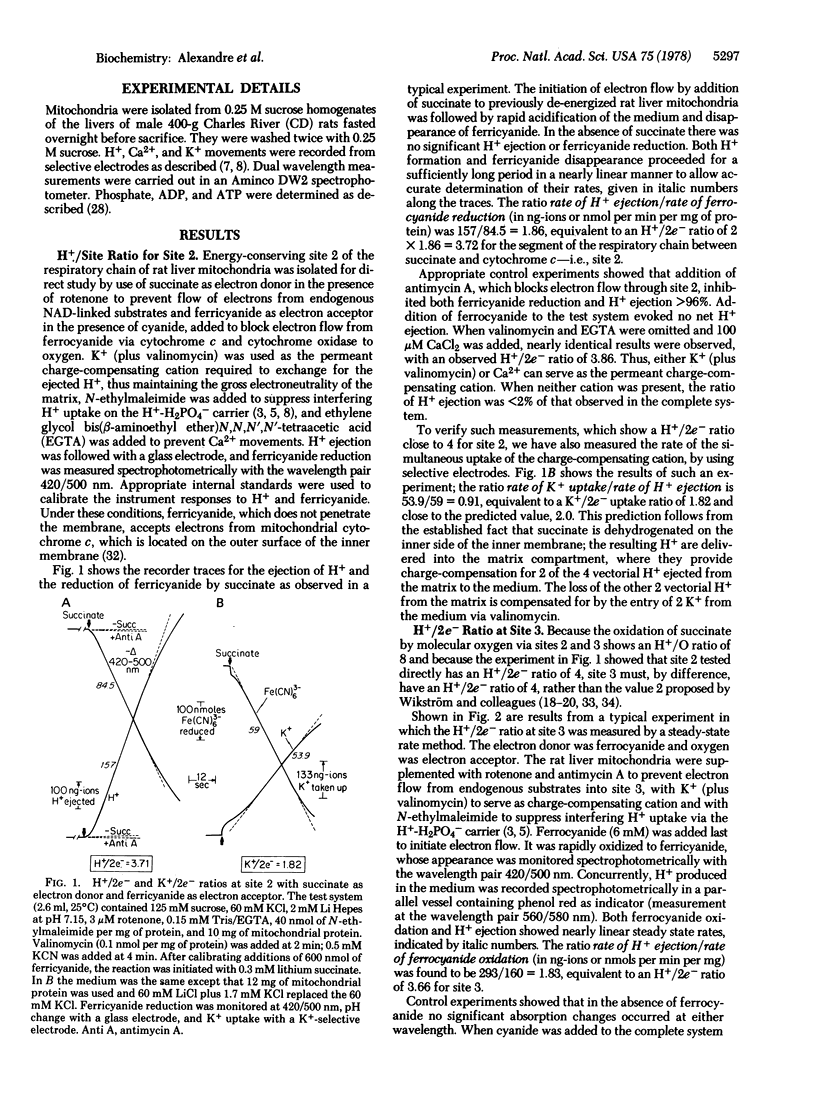
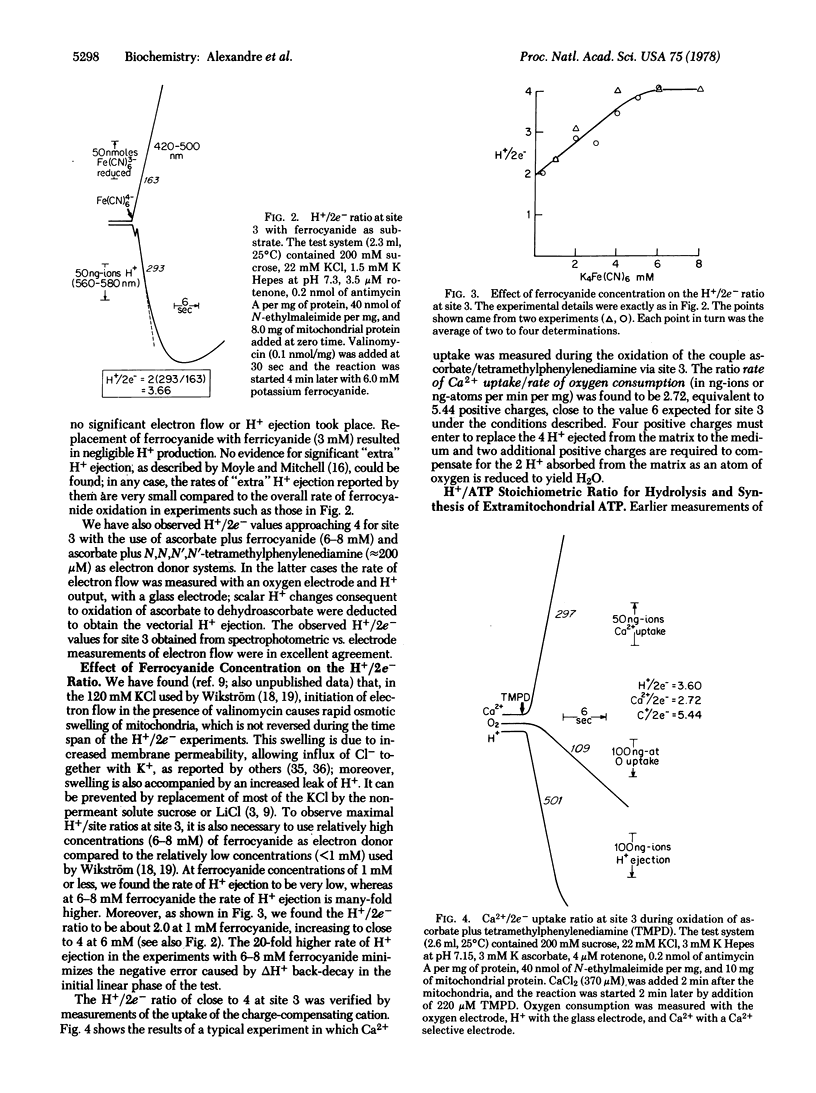
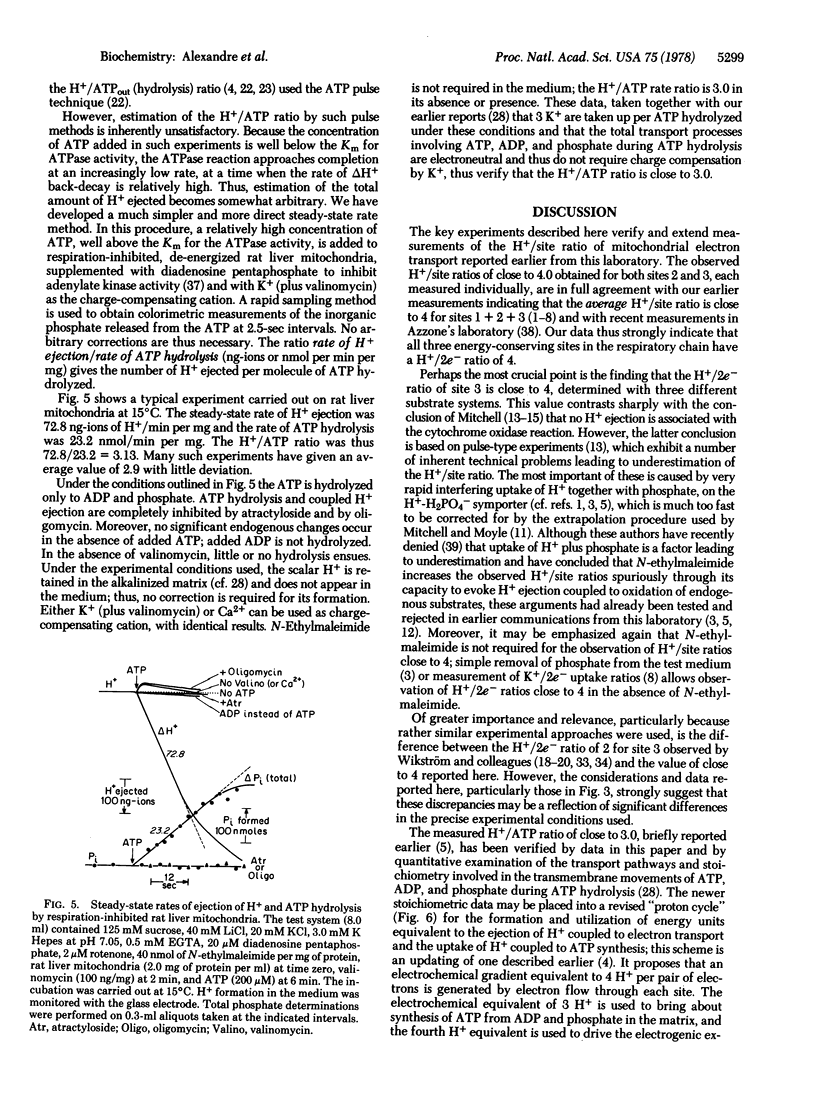
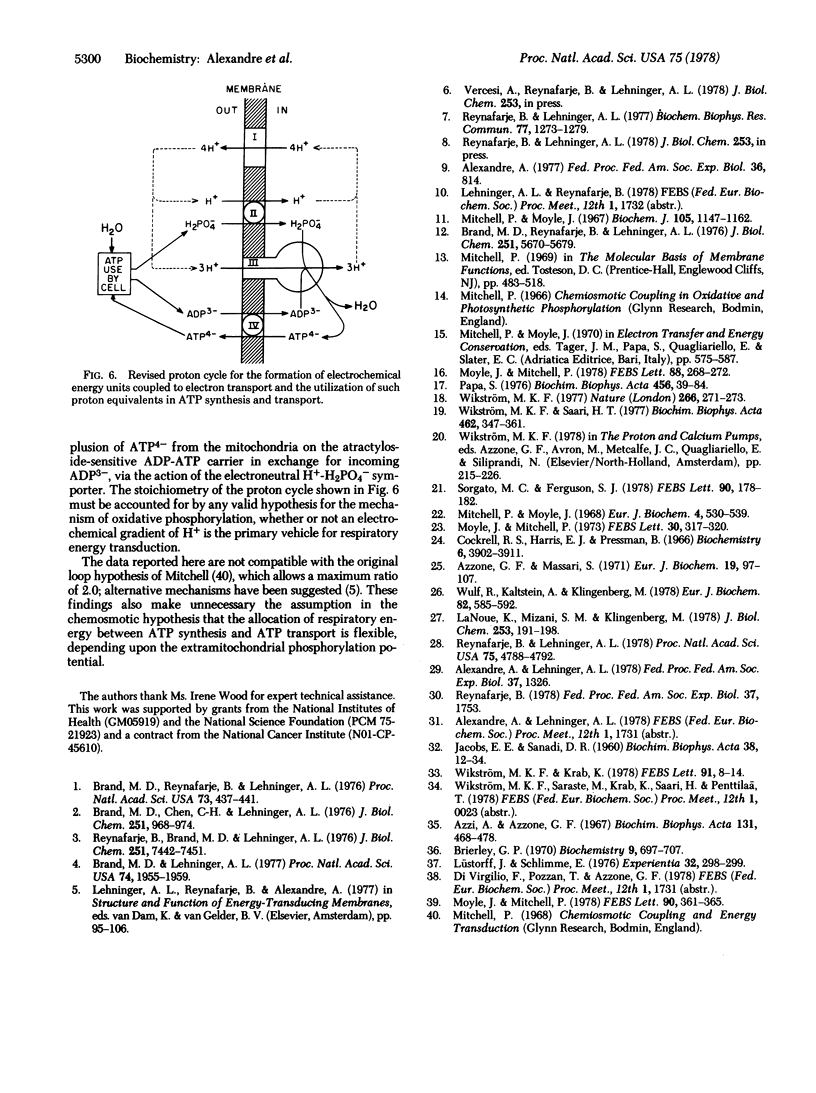
Selected References
These references are in PubMed. This may not be the complete list of references from this article.
- Azzi A., Azzone G. F. Swelling and shrinkage phenomena in liver mitochondria. VI. Metabolism-independent swelling coupled to ion movement. Biochim Biophys Acta. 1967 May 9;131(3):468–478. doi: 10.1016/0005-2728(67)90006-0. [DOI] [PubMed] [Google Scholar]
- Azzone G. F., Massari S. Thermodynamic and kinetic aspects of the interconversion of chemical and osmotic energies in mitochondria. Eur J Biochem. 1971 Mar 1;19(1):97–107. doi: 10.1111/j.1432-1033.1971.tb01292.x. [DOI] [PubMed] [Google Scholar]
- Brand M. D., Chen C. H., Lehninger A. L. Stoichiometry of H+ ejection during respiration-dependent accumulation of Ca2+ by rat liver mitochondria. J Biol Chem. 1976 Feb 25;251(4):968–974. [PubMed] [Google Scholar]
- Brand M. D., Lehninger A. L. H+/ATP ratio during ATP hydrolysis by mitochondria: modification of the chemiosmotic theory. Proc Natl Acad Sci U S A. 1977 May;74(5):1955–1959. doi: 10.1073/pnas.74.5.1955. [DOI] [PMC free article] [PubMed] [Google Scholar]
- Brand M. D., Reynafarje B., Lehninger A. L. Re-evaluation of the H+/site ratio of mitochondrial electron transport with the oxygen pulse technique. J Biol Chem. 1976 Sep 25;251(18):5670–5679. [PubMed] [Google Scholar]
- Brand M. D., Reynafarje B., Lehninger A. L. Stoichiometric relationship between energy-dependent proton ejection and electron transport in mitochondria. Proc Natl Acad Sci U S A. 1976 Feb;73(2):437–441. doi: 10.1073/pnas.73.2.437. [DOI] [PMC free article] [PubMed] [Google Scholar]
- Brierley G. P. Energy-linked alteration of the permeability of heart mitochondria to chloride and other anions. Biochemistry. 1970 Feb 17;9(4):697–707. doi: 10.1021/bi00806a001. [DOI] [PubMed] [Google Scholar]
- JACOBS E. E., SANADI D. R. Phosphorylation coupled to electron transport mediated by high potential electron carriers. Biochim Biophys Acta. 1960 Feb 12;38:12–34. doi: 10.1016/0006-3002(60)91192-6. [DOI] [PubMed] [Google Scholar]
- LaNoue K., Mizani S. M., Klingenberg M. Electrical imbalance of adenine nucleotide transport across the mitochondrial membrane. J Biol Chem. 1978 Jan 10;253(1):191–198. [PubMed] [Google Scholar]
- Lüstorff J., Schlimme E. Does an inhibitor of mitochondrial adenylate kinase also affect oxidative phosphorylation? Experientia. 1976 Mar 15;32(3):298–299. doi: 10.1007/BF01940801. [DOI] [PubMed] [Google Scholar]
- Mitchell P., Moyle J. Proton translocation coupled to ATP hydrolysis in rat liver mitochondria. Eur J Biochem. 1968 May;4(4):530–539. doi: 10.1111/j.1432-1033.1968.tb00245.x. [DOI] [PubMed] [Google Scholar]
- Mitchell P., Moyle J. Respiration-driven proton translocation in rat liver mitochondria. Biochem J. 1967 Dec;105(3):1147–1162. doi: 10.1042/bj1051147. [DOI] [PMC free article] [PubMed] [Google Scholar]
- Moyle J., Mitchell P. Cytochrome c oxidase is not a proton pump. FEBS Lett. 1978 Apr 15;88(2):268–272. doi: 10.1016/0014-5793(78)80190-2. [DOI] [PubMed] [Google Scholar]
- Moyle J., Mitchell P. Measurements of mitochondrial comes from H+/O quotients: effects of phosphate and N-ethylmaleimide. FEBS Lett. 1978 Jun 15;90(2):361–365. doi: 10.1016/0014-5793(78)80405-0. [DOI] [PubMed] [Google Scholar]
- Moyle J., Mitchell P. Proton translocation quotient for the adenosine triphosphatase of rat liver mitochondria. FEBS Lett. 1973 Mar 15;30(3):317–320. doi: 10.1016/0014-5793(73)80678-7. [DOI] [PubMed] [Google Scholar]
- Papa S. Proton translocation reactions in the respiratory chains. Biochim Biophys Acta. 1976 Apr 30;456(1):39–84. doi: 10.1016/0304-4173(76)90008-2. [DOI] [PubMed] [Google Scholar]
- Reynafarje B., Brand M. D., Lehninger A. L. Evaluation of the H+/site ratio of mitochondrial electron transport from rate measurements. J Biol Chem. 1976 Dec 10;251(23):7442–7451. [PubMed] [Google Scholar]
- Reynafarje B., Lehninger A. L. An alternative membrane transport pathway for phosphate and adenine nucleotides in mitochondria and its possible function. Proc Natl Acad Sci U S A. 1978 Oct;75(10):4788–4792. doi: 10.1073/pnas.75.10.4788. [DOI] [PMC free article] [PubMed] [Google Scholar]
- Reynafarje B., Lehninger A. L. Electric charge stoichiometry of calcium translocation in mitochondria. Biochem Biophys Res Commun. 1977 Aug 22;77(4):1273–1279. doi: 10.1016/s0006-291x(77)80117-4. [DOI] [PubMed] [Google Scholar]
- Sorgato M. C., Ferguson S. J. Measurements of the components of the protonmotive force generated by cytochrome oxidase in submitochondrial particles. FEBS Lett. 1978 Jun 1;90(1):178–182. doi: 10.1016/0014-5793(78)80324-x. [DOI] [PubMed] [Google Scholar]
- Wikstrom M. K. Proton pump coupled to cytochrome c oxidase in mitochondria. Nature. 1977 Mar 17;266(5599):271–273. doi: 10.1038/266271a0. [DOI] [PubMed] [Google Scholar]
- Wikström M. K., Saari H. T. The mechanism of energy conservation and transduction by mitochondrial cytochrome c oxidase. Biochim Biophys Acta. 1977 Nov 17;462(2):347–361. doi: 10.1016/0005-2728(77)90133-5. [DOI] [PubMed] [Google Scholar]
- Wikström M., Krab K. Cytochrome c oxidase is a proton pump: a rejoinder to recent criticism. FEBS Lett. 1978 Jul 1;91(1):8–14. doi: 10.1016/0014-5793(78)80006-4. [DOI] [PubMed] [Google Scholar]
- Wulf R., Kaltstein A., Klingenberg M. H+ and cation movements associated with ADP, ATP transport in mitochondria. Eur J Biochem. 1978 Jan 16;82(2):585–592. doi: 10.1111/j.1432-1033.1978.tb12054.x. [DOI] [PubMed] [Google Scholar]