Abstract
The actions of the phenothiazines chlorpromazine, prochlorperazine, and trifluoperazine were studied on the acetylcholine receptor-ionic channel complex of frog and rat skeletal muscle and of Torpedo californica to determine their role in pharmacological desensitization and their interactions with different states of the receptor-ionic channel complex. The phenothiazines depressed the peak amplitude of spontaneous and evoked endplate currents while having negligible effect on the decay time constants. Mean channel lifetime and single channel conductance were not altered by these drugs. They also produced a frequency-dependent depression of the peak amplitude of endplate potentials evoked by repetitive microiontophoresis at the extrajunctional region. In addition, these drugs enhanced the ability of carbamoylcholine to displace 125I-labeled alpha-bungarotoxin from receptor-rich membrane preparations of T. californica when used in concentrations that had no effect on 125I-labeled alpha-bungarotoxin binding alone (10 microM). Similarly, the phenothiazines inhibited the binding of tritiated ionic channel ligands, such as phencyclidine and perhydrohistrionicotoxin, a process also enhanced by the presence of carbamoylcholine. These data suggest that the phenothiazines augment agonist-induced desensitization primarily by interacting with the receptor-ionic channel complex prior to channel opening.
Full text
PDF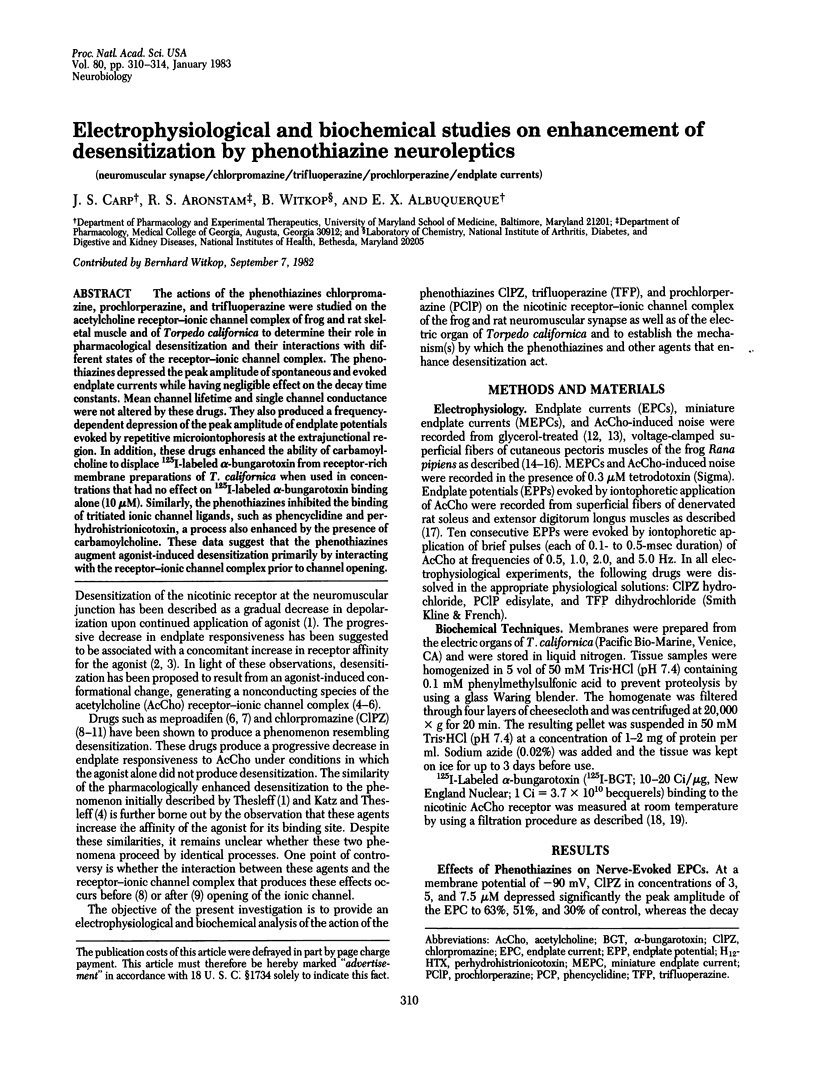
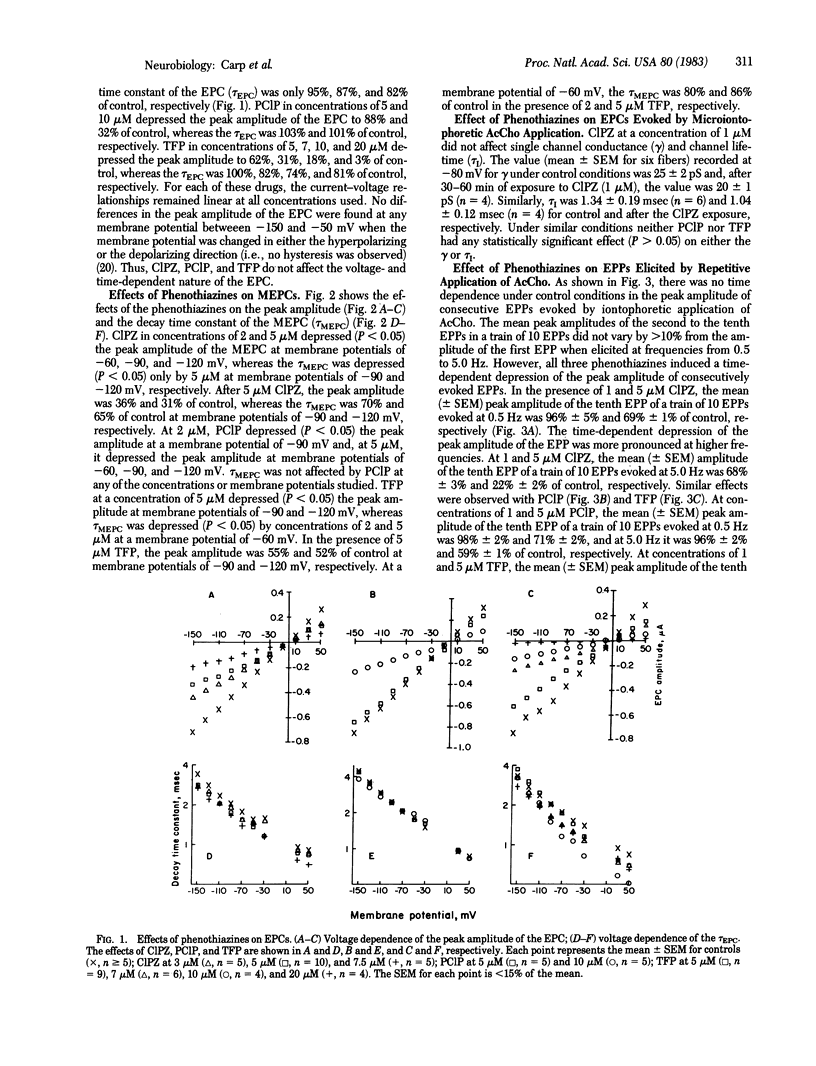
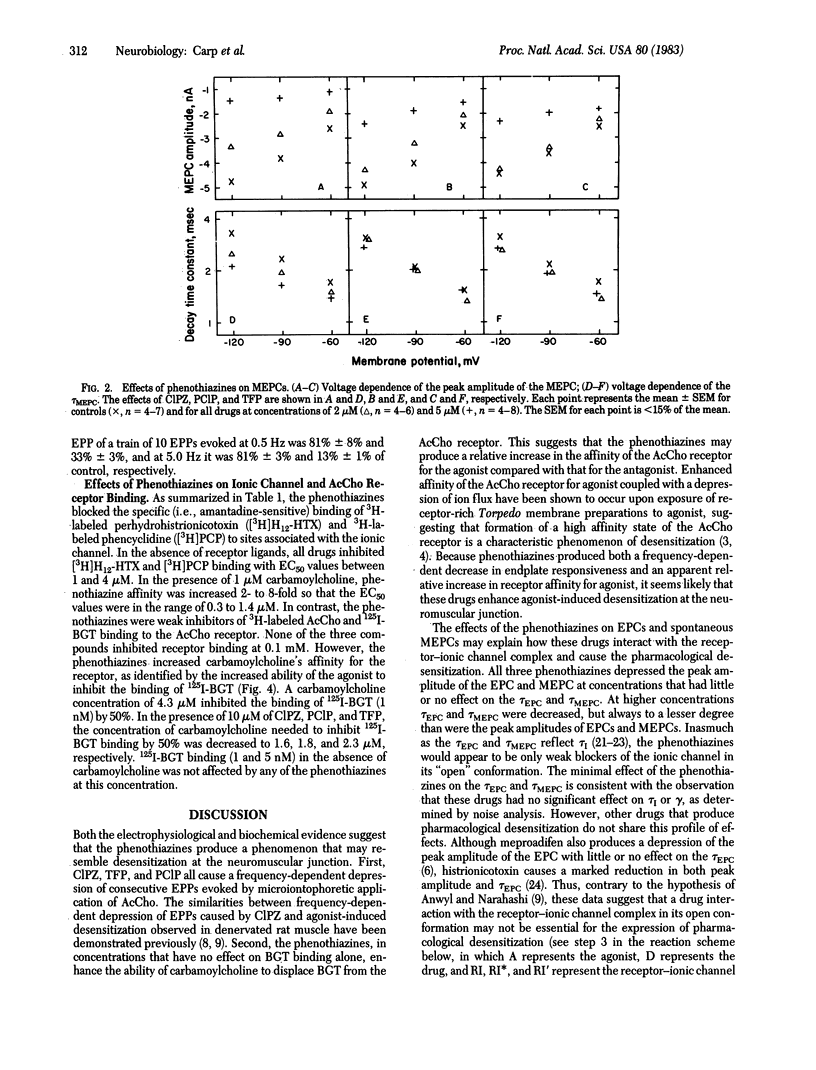
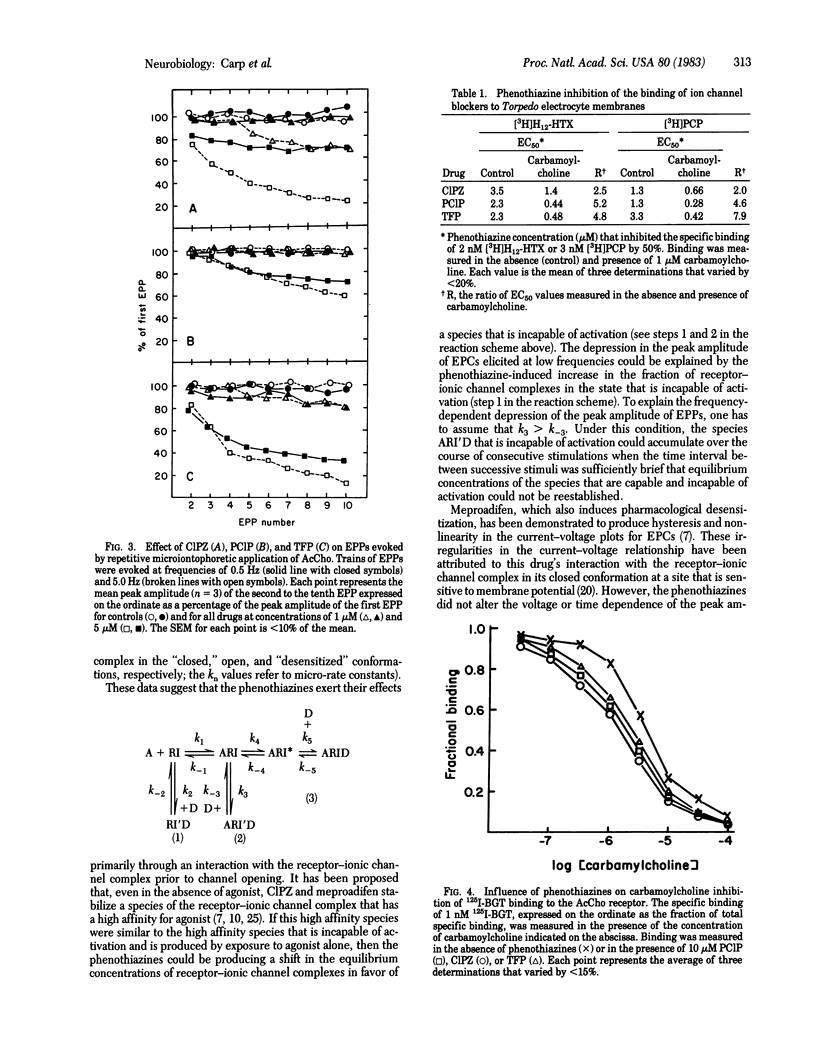
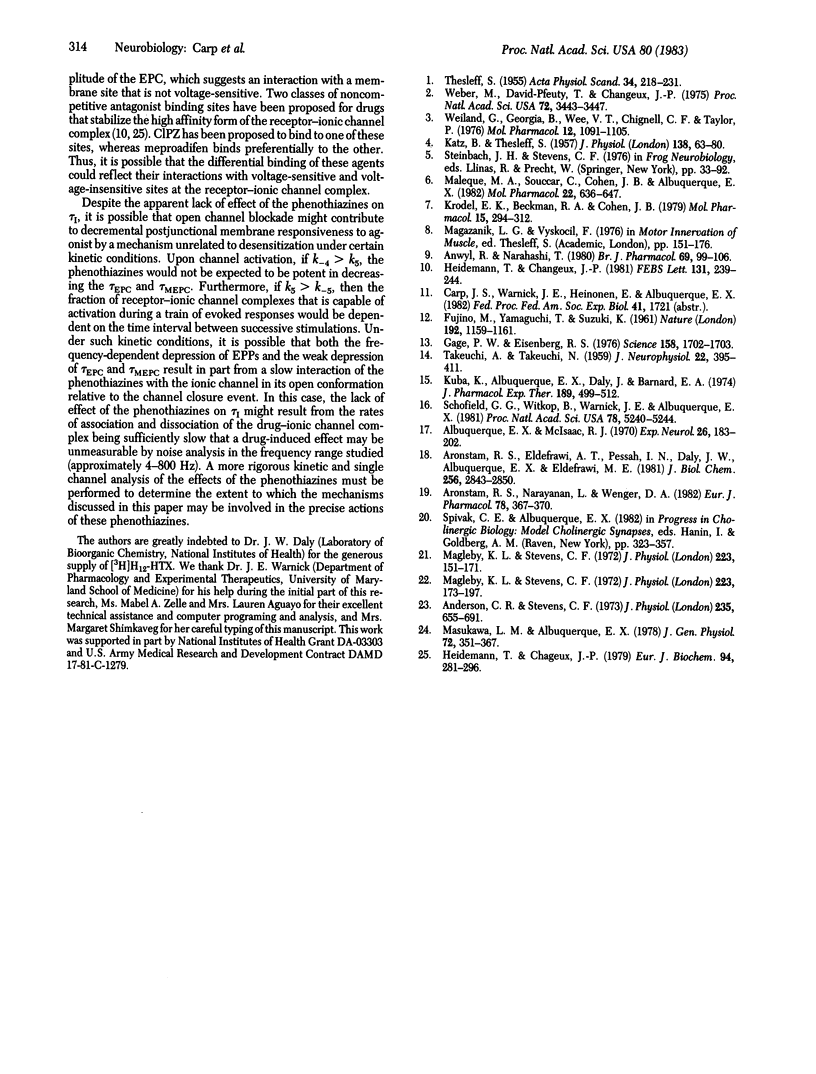
Selected References
These references are in PubMed. This may not be the complete list of references from this article.
- Albuquerque E. X., McIsaac R. J. Fast and slow mammalian muscles after denervation. Exp Neurol. 1970 Jan;26(1):183–202. doi: 10.1016/0014-4886(70)90099-3. [DOI] [PubMed] [Google Scholar]
- Anderson C. R., Stevens C. F. Voltage clamp analysis of acetylcholine produced end-plate current fluctuations at frog neuromuscular junction. J Physiol. 1973 Dec;235(3):655–691. doi: 10.1113/jphysiol.1973.sp010410. [DOI] [PMC free article] [PubMed] [Google Scholar]
- Anwyl R., Narahashi T. Comparison of desensitization and time-dependent block of the acetylcholine receptor responses by chlorpromazine, cytochalasin B, triton X-100 and other agents. Br J Pharmacol. 1980 May;69(1):99–106. doi: 10.1111/j.1476-5381.1980.tb10887.x. [DOI] [PMC free article] [PubMed] [Google Scholar]
- Aronstam R. S., Eldefrawi A. T., Pessah I. N., Daly J. W., Albuquerque E. X., Eldefrawi M. E. Regulation of [3H]perhydrohistrionicotoxin binding to Torpedo ocellata electroplax by effectors of the acetylcholine receptor. J Biol Chem. 1981 Mar 25;256(6):2843–2850. [PubMed] [Google Scholar]
- Aronstam R. S., Narayanan L., Wenger D. A. Ketamine inhibition of ligand binding to cholinergic receptors and ion channels. Eur J Pharmacol. 1982 Mar 12;78(3):367–370. doi: 10.1016/0014-2999(82)90040-1. [DOI] [PubMed] [Google Scholar]
- FUJINO M., YAMAGUCHI T., SUZUKI K. 'Glycerol effect' and the mechanism linking excitation of the plasma membrane with contraction. Nature. 1961 Dec 23;192:1159–1161. doi: 10.1038/1921159a0. [DOI] [PubMed] [Google Scholar]
- Gage P. W., Eisenberg R. S. Action potentials without contraction in frog skeletal muscle fibers with disrupted transverse tubules. Science. 1967 Dec 29;158(3809):1702–1703. doi: 10.1126/science.158.3809.1702. [DOI] [PubMed] [Google Scholar]
- Heidmann T., Changeux J. P. Fast kinetic studies on the allosteric interactions between acetylcholine receptor and local anesthetic binding sites. Eur J Biochem. 1979 Feb 15;94(1):281–296. doi: 10.1111/j.1432-1033.1979.tb12894.x. [DOI] [PubMed] [Google Scholar]
- Heidmann T., Changeux J. P. Stabilization of the high affinity state of the membrane-bound acetylcholine receptor from Torpedo marmorata by noncompetitive-blockers. Evidence for dual interaction and pharmacological selectivity. FEBS Lett. 1981 Aug 31;131(2):239–244. doi: 10.1016/0014-5793(81)80375-4. [DOI] [PubMed] [Google Scholar]
- KATZ B., THESLEFF S. A study of the desensitization produced by acetylcholine at the motor end-plate. J Physiol. 1957 Aug 29;138(1):63–80. doi: 10.1113/jphysiol.1957.sp005838. [DOI] [PMC free article] [PubMed] [Google Scholar]
- Krodel E. K., Beckman R. A., Cohen J. B. Identification of a local anesthetic binding site in nicotinic post-synaptic membranes isolated from Torpedo marmorata electric tissue. Mol Pharmacol. 1979 Mar;15(2):294–312. [PubMed] [Google Scholar]
- Kuba K., Albuquerque E. X., Daly J., Barnard E. A. A study of the irreversible cholinesterase inhibitor, diisopropylfluorophosphate, on time course of end-plate currents in frog sartorius muscle. J Pharmacol Exp Ther. 1974 May;189(2):499–512. [PubMed] [Google Scholar]
- Magleby K. L., Stevens C. F. A quantitative description of end-plate currents. J Physiol. 1972 May;223(1):173–197. doi: 10.1113/jphysiol.1972.sp009840. [DOI] [PMC free article] [PubMed] [Google Scholar]
- Magleby K. L., Stevens C. F. The effect of voltage on the time course of end-plate currents. J Physiol. 1972 May;223(1):151–171. doi: 10.1113/jphysiol.1972.sp009839. [DOI] [PMC free article] [PubMed] [Google Scholar]
- Maleque M. A., Souccar C., Cohen J. B., Albuquerque E. X. Meproadifen reaction with the ionic channel of the acetylcholine receptor: potentiation of agonist-induced desensitization at the frog neuromuscular junction. Mol Pharmacol. 1982 Nov;22(3):636–647. [PubMed] [Google Scholar]
- Masukawa L. M., Albuquerque E. X. Voltage- and time-dependent action of histrionicotoxin on the endplate current of the frog muscle. J Gen Physiol. 1978 Sep;72(3):351–367. doi: 10.1085/jgp.72.3.351. [DOI] [PMC free article] [PubMed] [Google Scholar]
- Schofield G. G., Witkop B., Warnick J. E., Albuquerque E. X. Differentiation of the open and closed states of the ionic channels of nicotinic acetylcholine receptors by tricyclic antidepressants. Proc Natl Acad Sci U S A. 1981 Aug;78(8):5240–5244. doi: 10.1073/pnas.78.8.5240. [DOI] [PMC free article] [PubMed] [Google Scholar]
- TAKEUCHI A., TAKEUCHI N. Active phase of frog's end-plate potential. J Neurophysiol. 1959 Jul;22(4):395–411. doi: 10.1152/jn.1959.22.4.395. [DOI] [PubMed] [Google Scholar]
- THESLEFT S. The mode of neuromuscular block caused by acetylcholine, nicotine, decamethonium and succinylcholine. Acta Physiol Scand. 1955 Oct 27;34(2-3):218–231. doi: 10.1111/j.1748-1716.1955.tb01242.x. [DOI] [PubMed] [Google Scholar]
- Weber M., David-Pfeuty T., Changeux J. P. Regulation of binding properties of the nicotinic receptor protein by cholinergic ligands in membrane fragments from Torpedo marmorata. Proc Natl Acad Sci U S A. 1975 Sep;72(9):3443–3447. doi: 10.1073/pnas.72.9.3443. [DOI] [PMC free article] [PubMed] [Google Scholar]
- Weiland G., Georgia B., Wee V. T., Chignell C. F., Taylor P. Ligand interactions with cholinergic receptor-enriched membranes from Torpedo: influence of agonist exposure on receptor properties. Mol Pharmacol. 1976 Nov;12(6):1091–1105. [PubMed] [Google Scholar]