Abstract
Phospholamban is a 52 amino acid calcium regulatory protein found as pentamers in cardiac SR membranes. The pentamers form through interactions between its transmembrane domains, and are stable in SDS. We have employed a saturation mutagenesis approach to study the detailed interactions between the transmembrane segments, using a chimeric protein construct in which staphylococcal nuclease (a monomeric soluble protein) is fused to the N-terminus of phospholamban. The chimera forms pentamers observable in SDS-PAGE, allowing the effects of mutations upon the oligomeric association to be determined by electrophoresis. The disruptive effects of amino acid substitutions in the transmembrane domain were classified as sensitive, moderately sensitive or insensitive. Residues of the same class lined up on faces of a 3.5 amino acids/turn helical projection, allowing the construction of a model of the interacting surfaces in which the helices are associated in a left-handed pentameric coiled-coil configuration. Molecular modeling simulations (to be described elsewhere in detail) confirm that the helices readily form a left-handed coiled-coil helical bundle and have yielded molecular models for the interacting surfaces, the best of which is identical to that predicted by the mutagenesis. Residues lining the pore show considerable structural sensitivity to mutation, indicating that care must be taken in interpreting the results of mutagenesis studies of channels. The cylindrical ion pore (minimal diameter of 2 A) appears to be defined largely by hydrophobic residues (I40, L43 and I47) with only two mildly polar elements contributed by sulfurs in residues C36 and M50.
Full text
PDF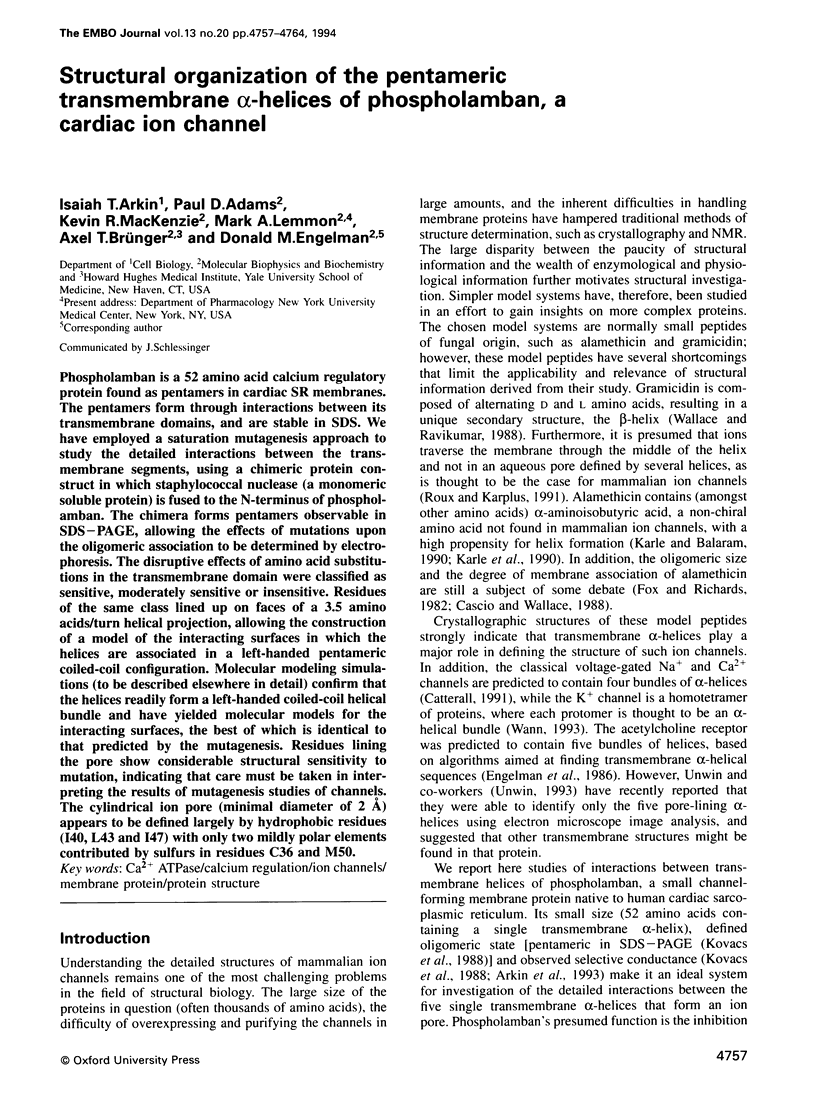
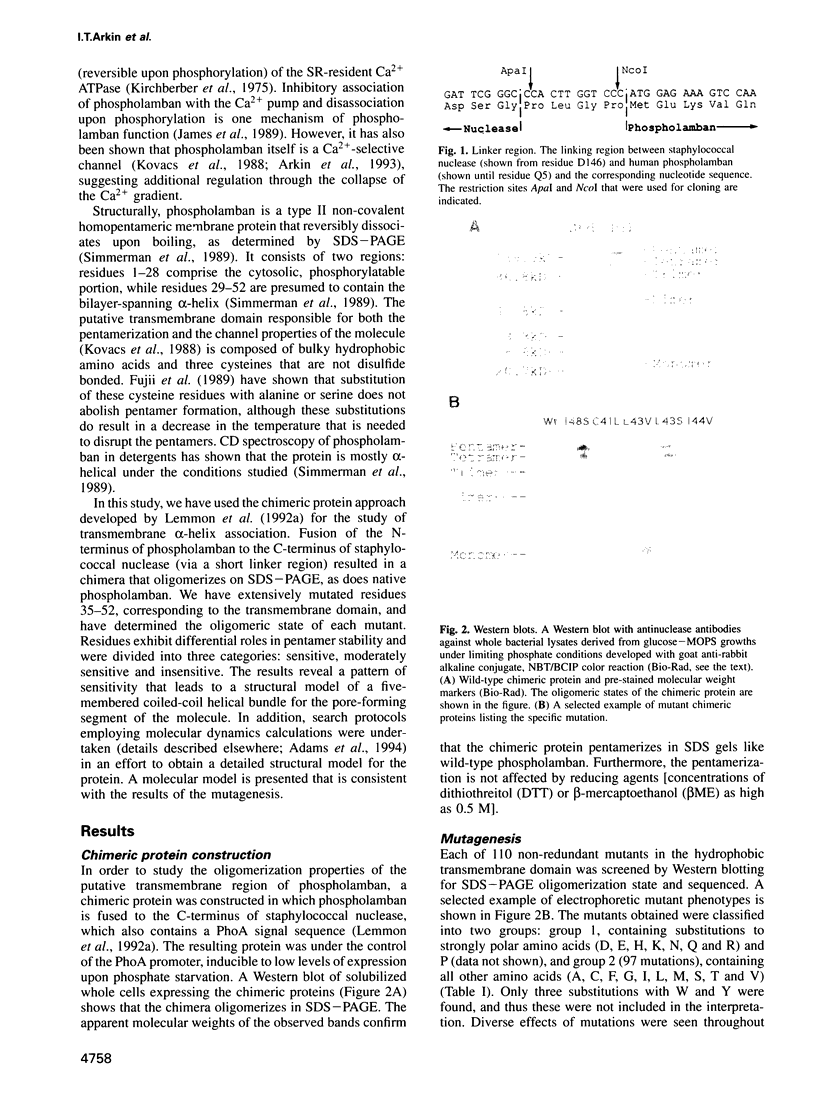
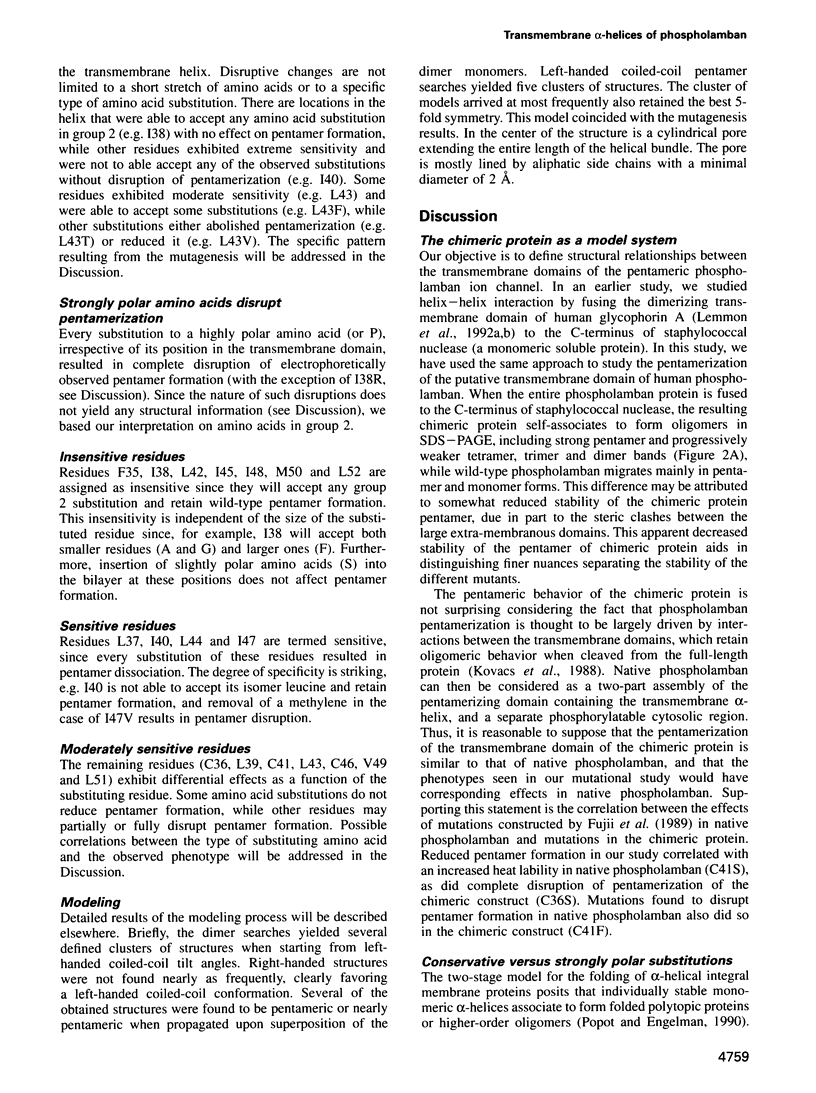
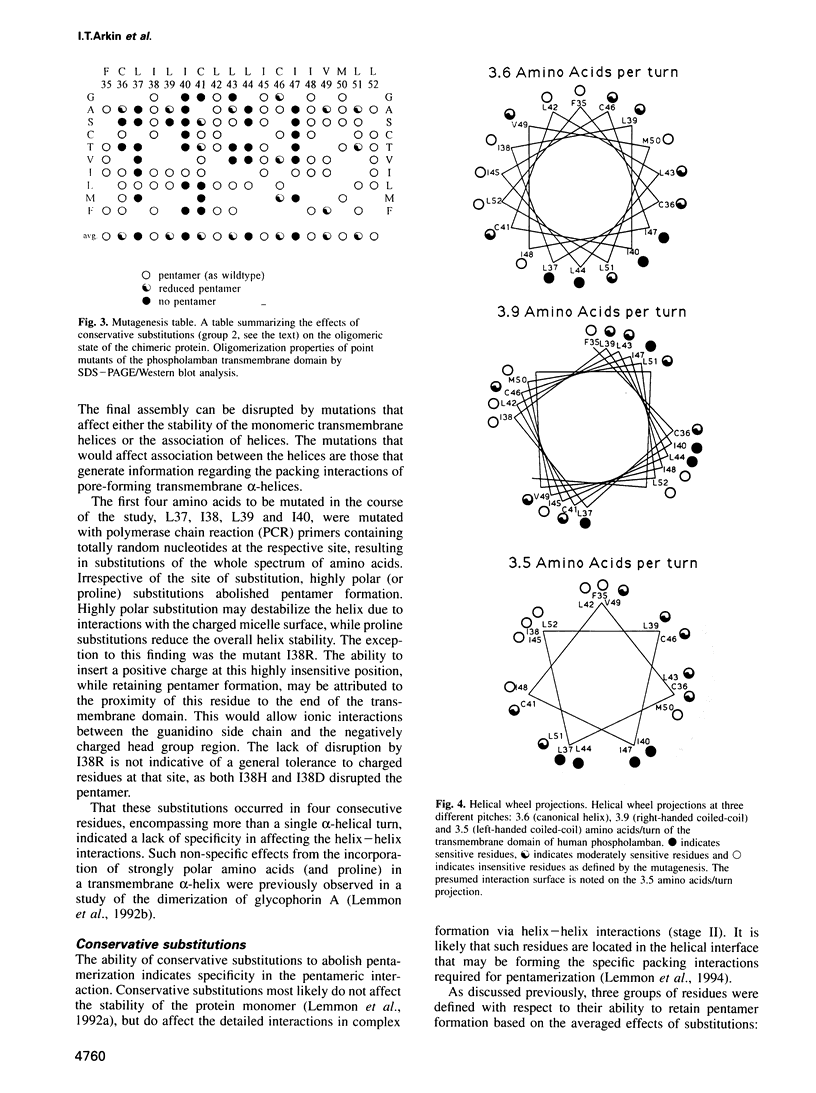
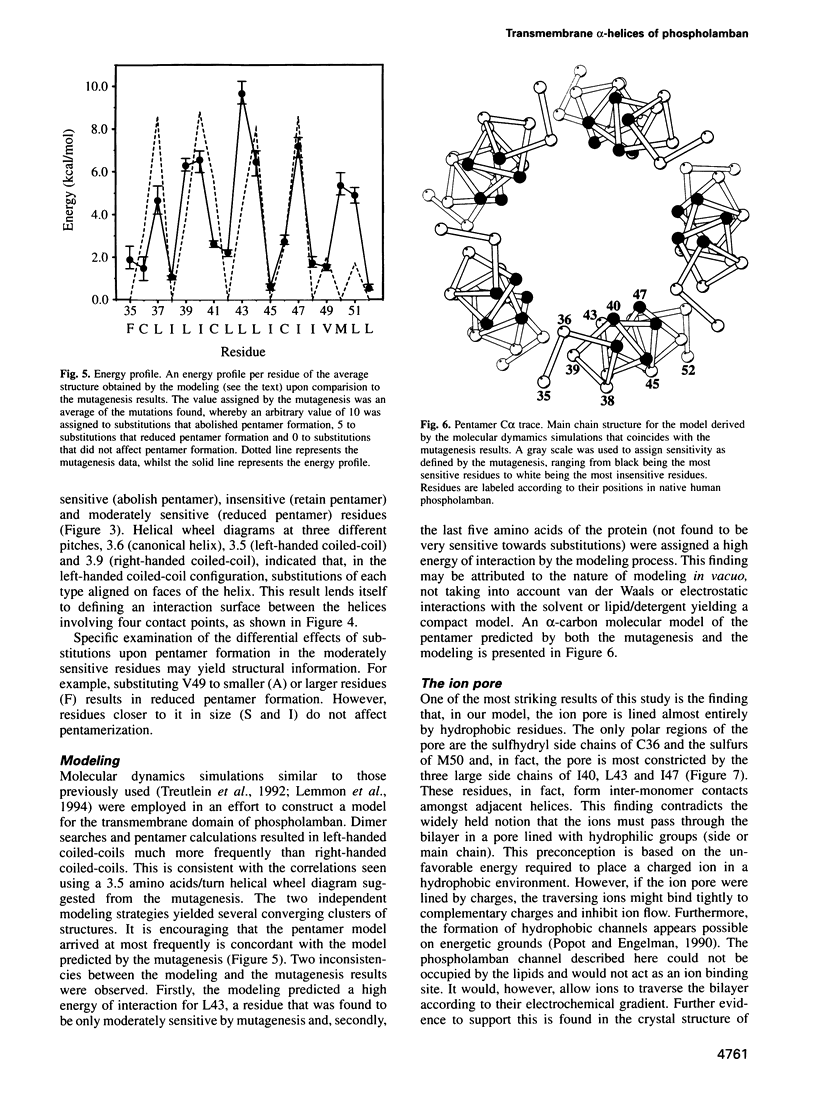
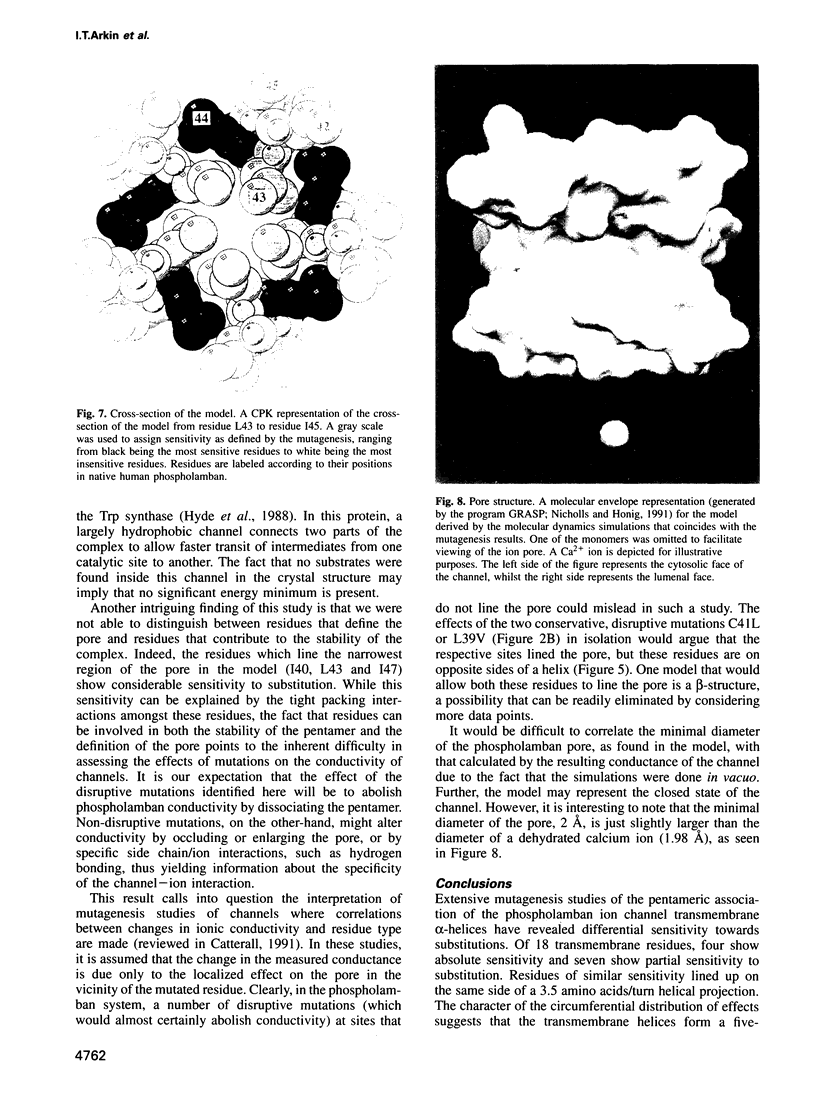
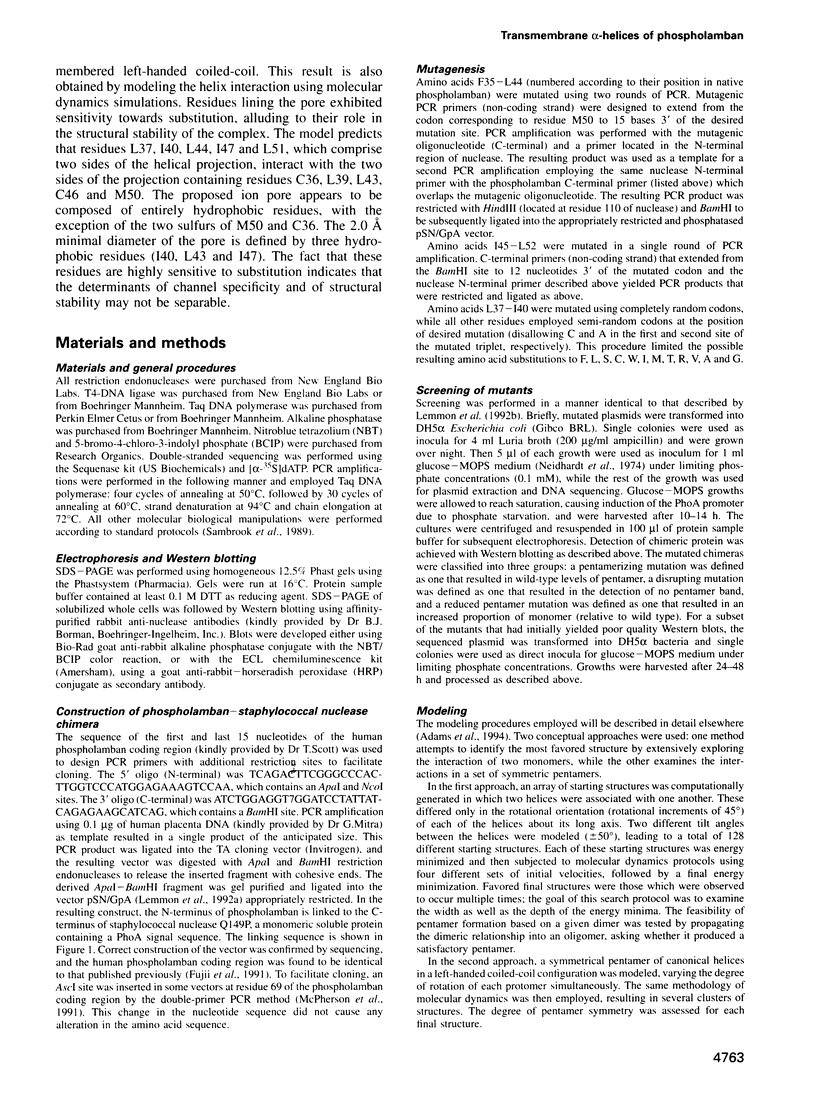
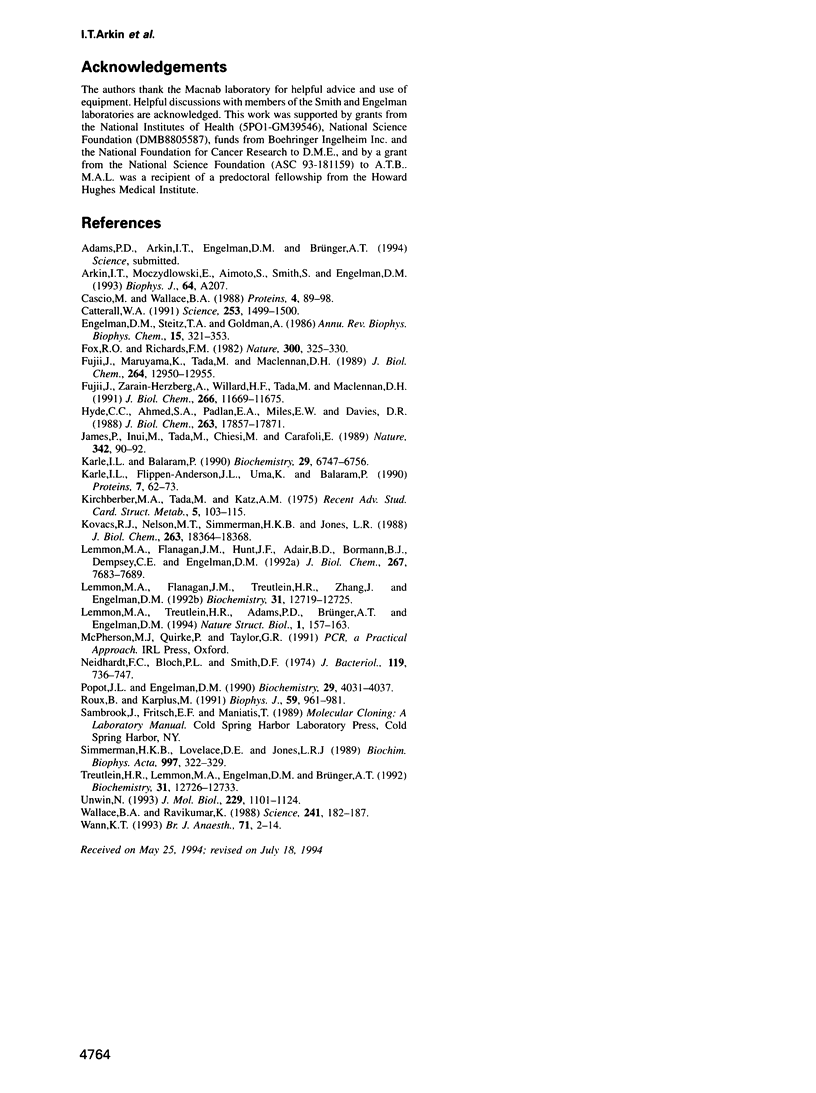
Images in this article
Selected References
These references are in PubMed. This may not be the complete list of references from this article.
- Cascio M., Wallace B. A. Conformation of alamethicin in phospholipid vesicles: implications for insertion models. Proteins. 1988;4(2):89–98. doi: 10.1002/prot.340040203. [DOI] [PubMed] [Google Scholar]
- Catterall W. A. Functional subunit structure of voltage-gated calcium channels. Science. 1991 Sep 27;253(5027):1499–1500. doi: 10.1126/science.1654596. [DOI] [PubMed] [Google Scholar]
- Engelman D. M., Steitz T. A., Goldman A. Identifying nonpolar transbilayer helices in amino acid sequences of membrane proteins. Annu Rev Biophys Biophys Chem. 1986;15:321–353. doi: 10.1146/annurev.bb.15.060186.001541. [DOI] [PubMed] [Google Scholar]
- Fox R. O., Jr, Richards F. M. A voltage-gated ion channel model inferred from the crystal structure of alamethicin at 1.5-A resolution. Nature. 1982 Nov 25;300(5890):325–330. doi: 10.1038/300325a0. [DOI] [PubMed] [Google Scholar]
- Fujii J., Maruyama K., Tada M., MacLennan D. H. Expression and site-specific mutagenesis of phospholamban. Studies of residues involved in phosphorylation and pentamer formation. J Biol Chem. 1989 Aug 5;264(22):12950–12955. [PubMed] [Google Scholar]
- Fujii J., Zarain-Herzberg A., Willard H. F., Tada M., MacLennan D. H. Structure of the rabbit phospholamban gene, cloning of the human cDNA, and assignment of the gene to human chromosome 6. J Biol Chem. 1991 Jun 25;266(18):11669–11675. [PubMed] [Google Scholar]
- Hyde C. C., Ahmed S. A., Padlan E. A., Miles E. W., Davies D. R. Three-dimensional structure of the tryptophan synthase alpha 2 beta 2 multienzyme complex from Salmonella typhimurium. J Biol Chem. 1988 Nov 25;263(33):17857–17871. [PubMed] [Google Scholar]
- James P., Inui M., Tada M., Chiesi M., Carafoli E. Nature and site of phospholamban regulation of the Ca2+ pump of sarcoplasmic reticulum. Nature. 1989 Nov 2;342(6245):90–92. doi: 10.1038/342090a0. [DOI] [PubMed] [Google Scholar]
- Karle I. L., Balaram P. Structural characteristics of alpha-helical peptide molecules containing Aib residues. Biochemistry. 1990 Jul 24;29(29):6747–6756. doi: 10.1021/bi00481a001. [DOI] [PubMed] [Google Scholar]
- Karle I. L., Flippen-Anderson J. L., Uma K., Balaram P. Apolar peptide models for conformational heterogeneity, hydration, and packing of polypeptide helices: crystal structure of hepta- and octapeptides containing alpha-aminoisobutyric acid. Proteins. 1990;7(1):62–73. doi: 10.1002/prot.340070107. [DOI] [PubMed] [Google Scholar]
- Kirchberber M. A., Tada M., Katz A. M. Phospholamban: a regulatory protein of the cardiac sarcoplasmic reticulum. Recent Adv Stud Cardiac Struct Metab. 1975;5:103–115. [PubMed] [Google Scholar]
- Kovacs R. J., Nelson M. T., Simmerman H. K., Jones L. R. Phospholamban forms Ca2+-selective channels in lipid bilayers. J Biol Chem. 1988 Dec 5;263(34):18364–18368. [PubMed] [Google Scholar]
- Lemmon M. A., Flanagan J. M., Hunt J. F., Adair B. D., Bormann B. J., Dempsey C. E., Engelman D. M. Glycophorin A dimerization is driven by specific interactions between transmembrane alpha-helices. J Biol Chem. 1992 Apr 15;267(11):7683–7689. [PubMed] [Google Scholar]
- Lemmon M. A., Flanagan J. M., Treutlein H. R., Zhang J., Engelman D. M. Sequence specificity in the dimerization of transmembrane alpha-helices. Biochemistry. 1992 Dec 29;31(51):12719–12725. doi: 10.1021/bi00166a002. [DOI] [PubMed] [Google Scholar]
- Lemmon M. A., Treutlein H. R., Adams P. D., Brünger A. T., Engelman D. M. A dimerization motif for transmembrane alpha-helices. Nat Struct Biol. 1994 Mar;1(3):157–163. doi: 10.1038/nsb0394-157. [DOI] [PubMed] [Google Scholar]
- Neidhardt F. C., Bloch P. L., Smith D. F. Culture medium for enterobacteria. J Bacteriol. 1974 Sep;119(3):736–747. doi: 10.1128/jb.119.3.736-747.1974. [DOI] [PMC free article] [PubMed] [Google Scholar]
- Popot J. L., Engelman D. M. Membrane protein folding and oligomerization: the two-stage model. Biochemistry. 1990 May 1;29(17):4031–4037. doi: 10.1021/bi00469a001. [DOI] [PubMed] [Google Scholar]
- Roux B., Karplus M. Ion transport in a model gramicidin channel. Structure and thermodynamics. Biophys J. 1991 May;59(5):961–981. doi: 10.1016/S0006-3495(91)82311-6. [DOI] [PMC free article] [PubMed] [Google Scholar]
- Simmerman H. K., Lovelace D. E., Jones L. R. Secondary structure of detergent-solubilized phospholamban, a phosphorylatable, oligomeric protein of cardiac sarcoplasmic reticulum. Biochim Biophys Acta. 1989 Aug 31;997(3):322–329. doi: 10.1016/0167-4838(89)90203-3. [DOI] [PubMed] [Google Scholar]
- Treutlein H. R., Lemmon M. A., Engelman D. M., Brünger A. T. The glycophorin A transmembrane domain dimer: sequence-specific propensity for a right-handed supercoil of helices. Biochemistry. 1992 Dec 29;31(51):12726–12732. doi: 10.1021/bi00166a003. [DOI] [PubMed] [Google Scholar]
- Unwin N. Nicotinic acetylcholine receptor at 9 A resolution. J Mol Biol. 1993 Feb 20;229(4):1101–1124. doi: 10.1006/jmbi.1993.1107. [DOI] [PubMed] [Google Scholar]
- Wallace B. A., Ravikumar K. The gramicidin pore: crystal structure of a cesium complex. Science. 1988 Jul 8;241(4862):182–187. doi: 10.1126/science.2455344. [DOI] [PubMed] [Google Scholar]
- Wann K. T. Neuronal sodium and potassium channels: structure and function. Br J Anaesth. 1993 Jul;71(1):2–14. doi: 10.1093/bja/71.1.2. [DOI] [PubMed] [Google Scholar]