Abstract
In the cerebral cortex, the small volume of the extracellular space in relation to the volume enclosed by synapses suggests an important functional role for this relationship. It is well known that there are atoms and molecules in the extracellular space that are absolutely necessary for synapses to function (e.g., calcium). I propose here the hypothesis that the rapid shift of these atoms and molecules from extracellular to intrasynaptic compartments represents the consumption of a shared, limited resource available to local volumes of neural tissue. Such consumption results in a dramatic competition among synapses for resources necessary for their function. In this paper, I explore a theory in which this resource consumption plays a critical role in the way local volumes of neural tissue operate. On short time scales, this principle of resource consumption permits a tissue volume to choose those synapses that function in a particular context and thereby helps to integrate the many neural signals that impinge on a tissue volume at any given moment. On longer time scales, the same principle aids in the stable storage and recall of information. The theory provides one framework for understanding how cerebral cortical tissue volumes integrate, attend to, store, and recall information. In this account, the capacity of neural tissue to attend to stimuli is intimately tied to the way tissue volumes are organized at fine spatial scales.
Full text
PDF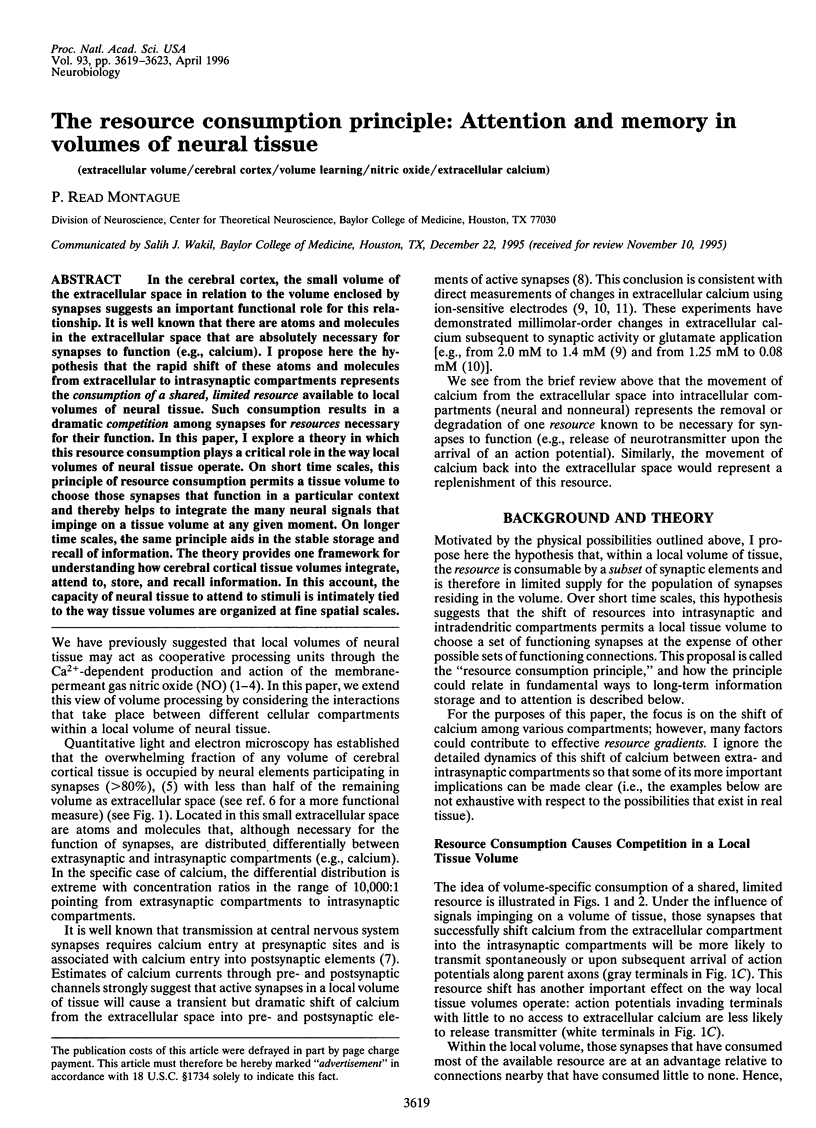
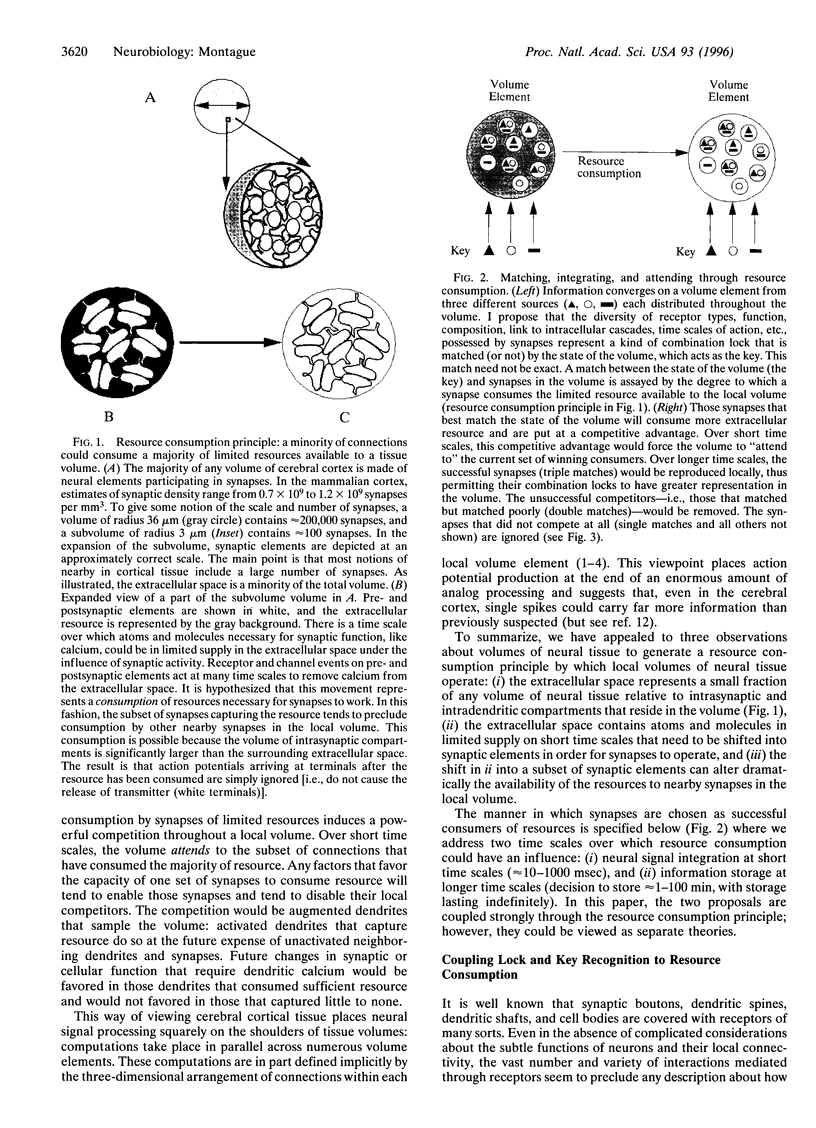
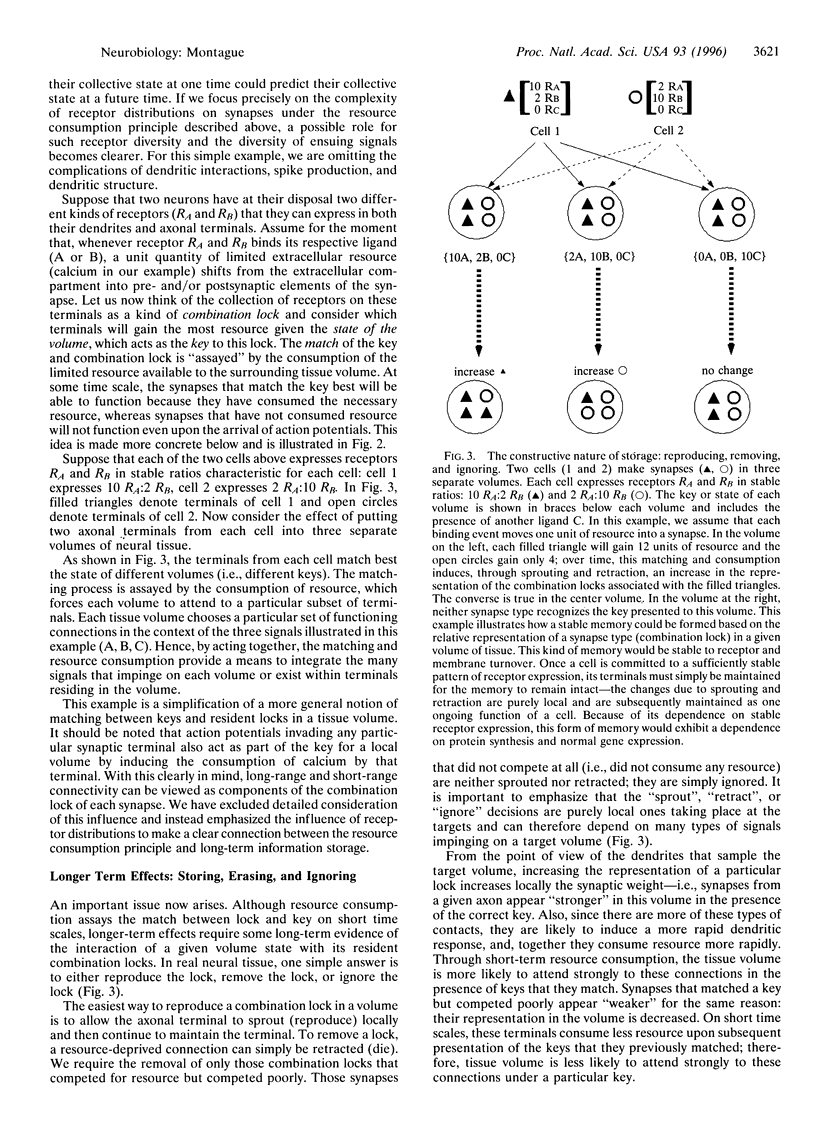
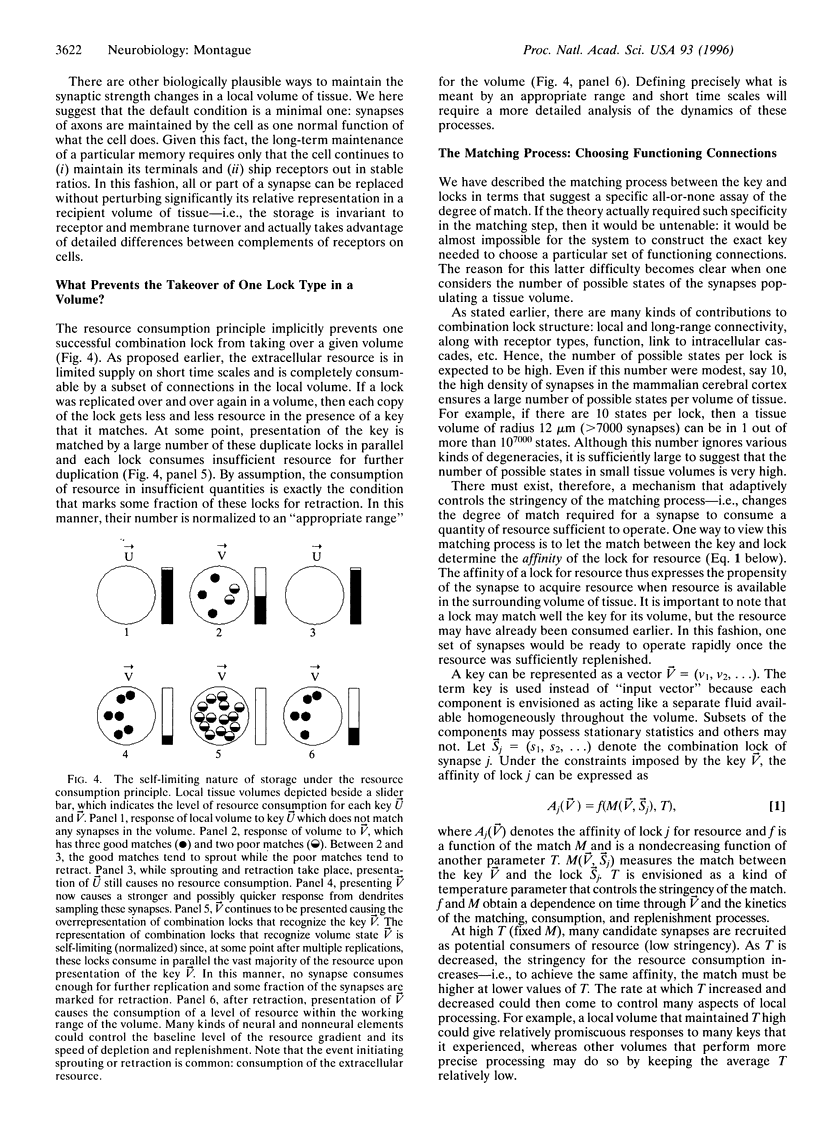
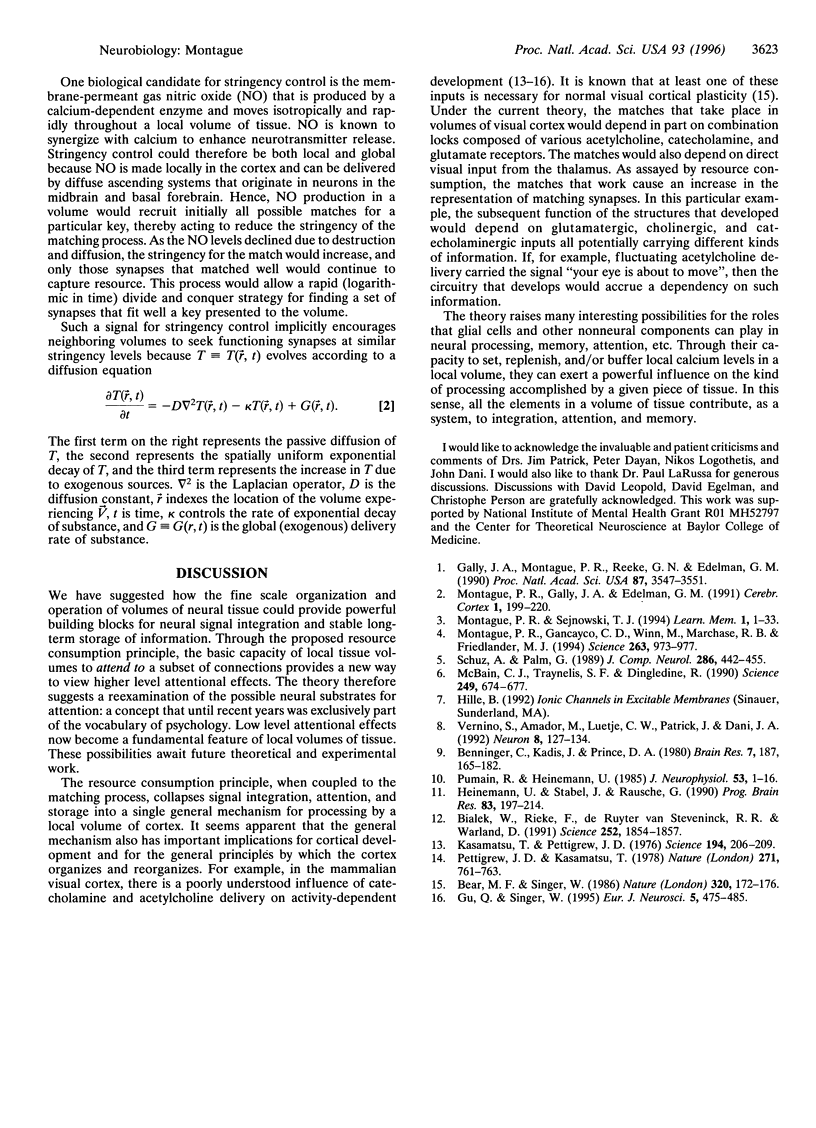
Images in this article
Selected References
These references are in PubMed. This may not be the complete list of references from this article.
- Bear M. F., Singer W. Modulation of visual cortical plasticity by acetylcholine and noradrenaline. Nature. 1986 Mar 13;320(6058):172–176. doi: 10.1038/320172a0. [DOI] [PubMed] [Google Scholar]
- Benninger C., Kadis J., Prince D. A. Extracellular calcium and potassium changes in hippocampal slices. Brain Res. 1980 Apr 7;187(1):165–182. doi: 10.1016/0006-8993(80)90502-8. [DOI] [PubMed] [Google Scholar]
- Bialek W., Rieke F., de Ruyter van Steveninck R. R., Warland D. Reading a neural code. Science. 1991 Jun 28;252(5014):1854–1857. doi: 10.1126/science.2063199. [DOI] [PubMed] [Google Scholar]
- Gally J. A., Montague P. R., Reeke G. N., Jr, Edelman G. M. The NO hypothesis: possible effects of a short-lived, rapidly diffusible signal in the development and function of the nervous system. Proc Natl Acad Sci U S A. 1990 May;87(9):3547–3551. doi: 10.1073/pnas.87.9.3547. [DOI] [PMC free article] [PubMed] [Google Scholar]
- Gu Q., Singer W. Effects of intracortical infusion of anticholinergic drugs on neuronal plasticity in kitten striate cortex. Eur J Neurosci. 1993 May 1;5(5):475–485. doi: 10.1111/j.1460-9568.1993.tb00514.x. [DOI] [PubMed] [Google Scholar]
- Heinemann U., Stabel J., Rausche G. Activity-dependent ionic changes and neuronal plasticity in rat hippocampus. Prog Brain Res. 1990;83:197–214. doi: 10.1016/s0079-6123(08)61250-9. [DOI] [PubMed] [Google Scholar]
- Kasamatsu T., Pettigrew J. D. Depletion of brain catecholamines: failure of ocular dominance shift after monocular occlusion in kittens. Science. 1976 Oct 8;194(4261):206–209. doi: 10.1126/science.959850. [DOI] [PubMed] [Google Scholar]
- McBain C. J., Traynelis S. F., Dingledine R. Regional variation of extracellular space in the hippocampus. Science. 1990 Aug 10;249(4969):674–677. doi: 10.1126/science.2382142. [DOI] [PubMed] [Google Scholar]
- Montague P. R., Gally J. A., Edelman G. M. Spatial signaling in the development and function of neural connections. Cereb Cortex. 1991 May-Jun;1(3):199–220. doi: 10.1093/cercor/1.3.199. [DOI] [PubMed] [Google Scholar]
- Montague P. R., Gancayco C. D., Winn M. J., Marchase R. B., Friedlander M. J. Role of NO production in NMDA receptor-mediated neurotransmitter release in cerebral cortex. Science. 1994 Feb 18;263(5149):973–977. doi: 10.1126/science.7508638. [DOI] [PubMed] [Google Scholar]
- Montague P. R., Sejnowski T. J. The predictive brain: temporal coincidence and temporal order in synaptic learning mechanisms. Learn Mem. 1994 May-Jun;1(1):1–33. [PubMed] [Google Scholar]
- Pettigrew J. D., Kasamatsu T. Local perfusion of noradrenaline maintains visual cortical plasticity. Nature. 1978 Feb 23;271(5647):761–763. doi: 10.1038/271761a0. [DOI] [PubMed] [Google Scholar]
- Pumain R., Heinemann U. Stimulus- and amino acid-induced calcium and potassium changes in rat neocortex. J Neurophysiol. 1985 Jan;53(1):1–16. doi: 10.1152/jn.1985.53.1.1. [DOI] [PubMed] [Google Scholar]
- Schüz A., Palm G. Density of neurons and synapses in the cerebral cortex of the mouse. J Comp Neurol. 1989 Aug 22;286(4):442–455. doi: 10.1002/cne.902860404. [DOI] [PubMed] [Google Scholar]
- Vernino S., Amador M., Luetje C. W., Patrick J., Dani J. A. Calcium modulation and high calcium permeability of neuronal nicotinic acetylcholine receptors. Neuron. 1992 Jan;8(1):127–134. doi: 10.1016/0896-6273(92)90114-s. [DOI] [PubMed] [Google Scholar]