Abstract
Energy-linked reverse electron transport from succinate to endogenous NAD in tightly coupled mung bean (Phaseolus aureus) mitochondria may be driven by ATP if the two terminal oxidases of these mitochondria are inhibited, or may be driven by the free energy of succinate oxidation. This reaction is specific to the first site of energy conservation of the respiratory chain; it does not occur in the presence of uncoupler. If mung bean mitochondria become anaerobic during oxidation of succinate, their endogenous NAD becomes reduced in the presence of uncoupler, provided that both inorganic phosphate (Pi) and ATP are present. No reduction occurs in the absence of Pi, even in the presence of ATP added to provide a high phosphate potential. If fluorooxaloacetate is present in the uncoupled, aerobic steady state, no reduction of endogenous NAD occurs on anaerobiosis; this compound is an inhibitor of malate dehydrogenase. This result implies that endogenous NAD is reduced by malate formed from the fumarate generated during succinate oxidation. The source of free energy is most probably the endogenous energy stores in the form of acetyl CoA, or intermediates convertible to acetyl CoA, which removes the oxaloacetate formed from malate, thus driving the reaction towards reduction of NAD.
In the absence of Pi and presence of oligomycin, oxidation of succinate by the alternative cyanide-insensitive oxidase pathway, in the presence of sulfide to inhibit cytochrome oxidase, does not reduce endogenous NAD, either in the aerobic steady state or in anaerobiosis. Under these conditions, only the reversed electron transport pathway from succinate to endogenous NAD is active and ATP cannot interact with the respiratory chain. The source of energy for NAD reduction must come from the respiratory chain, and this result shows that oxidation of succinate through the alternate pathway does not provide this energy.
Full text
PDF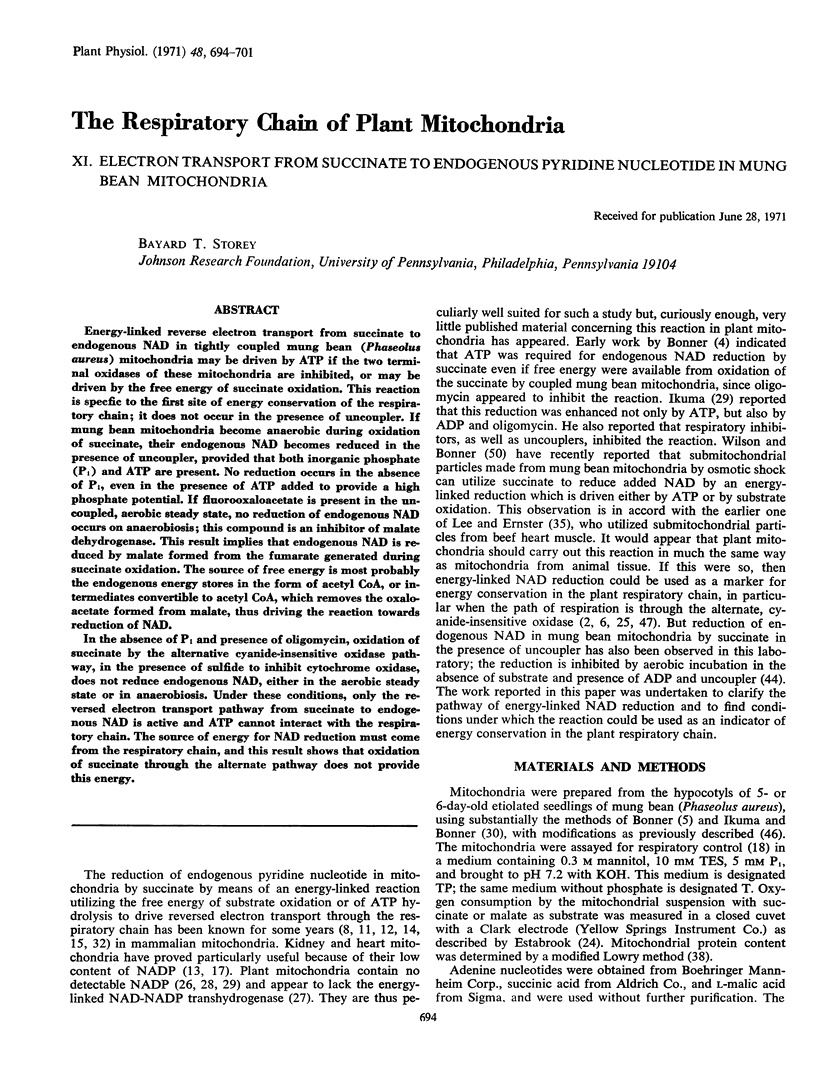
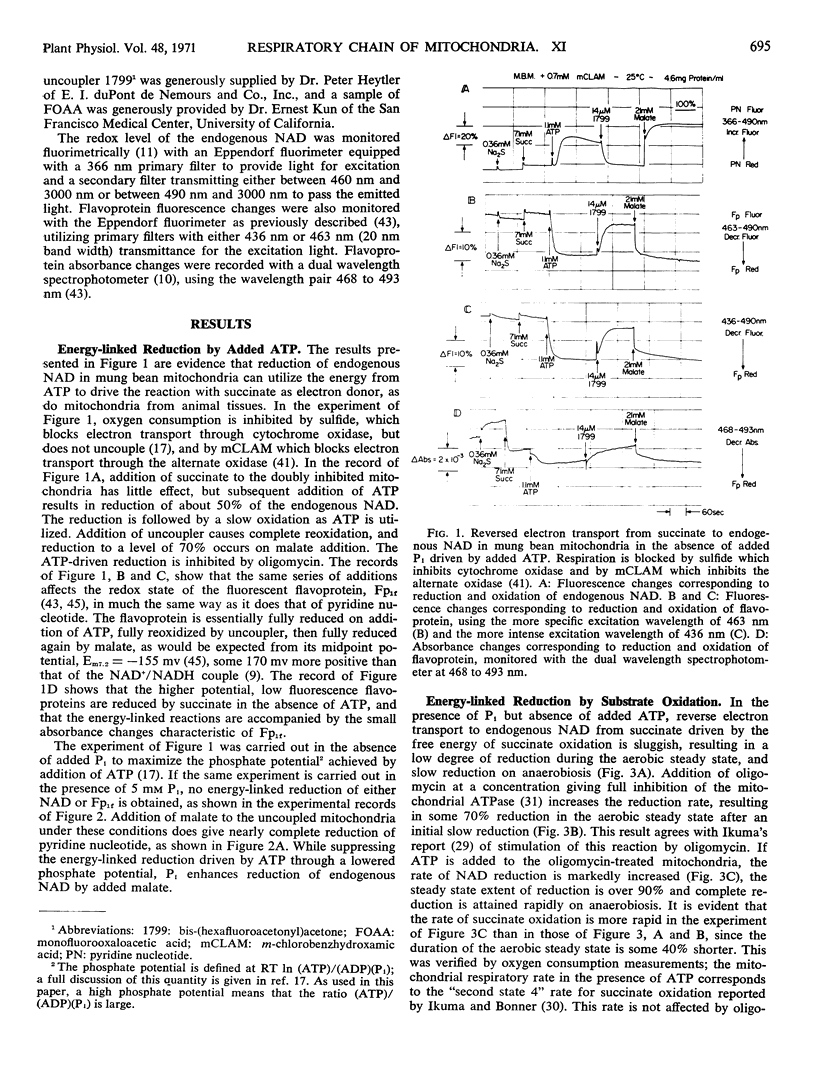
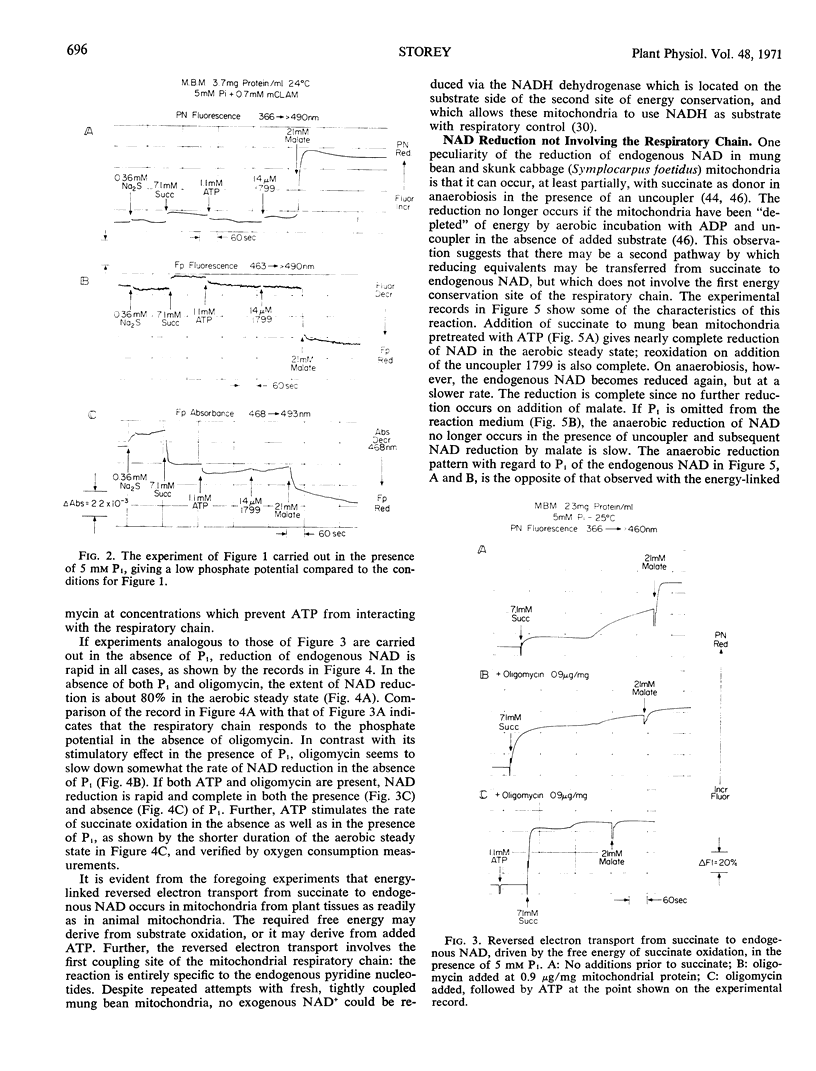
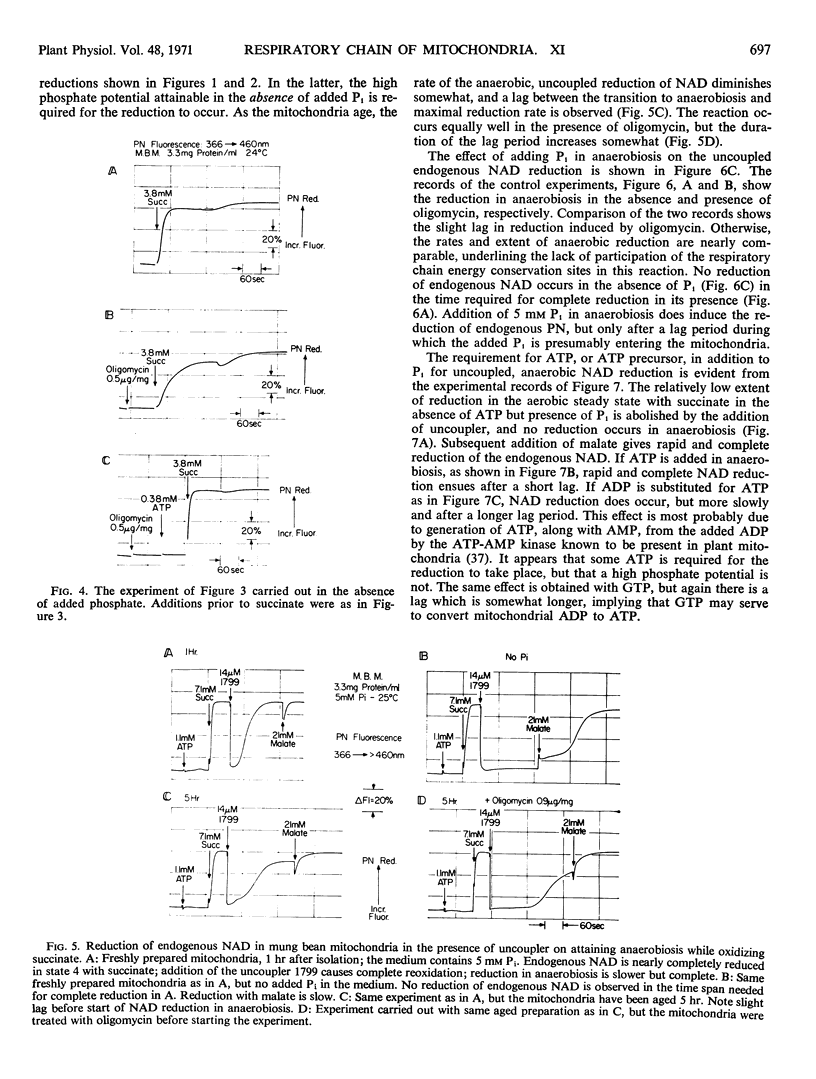
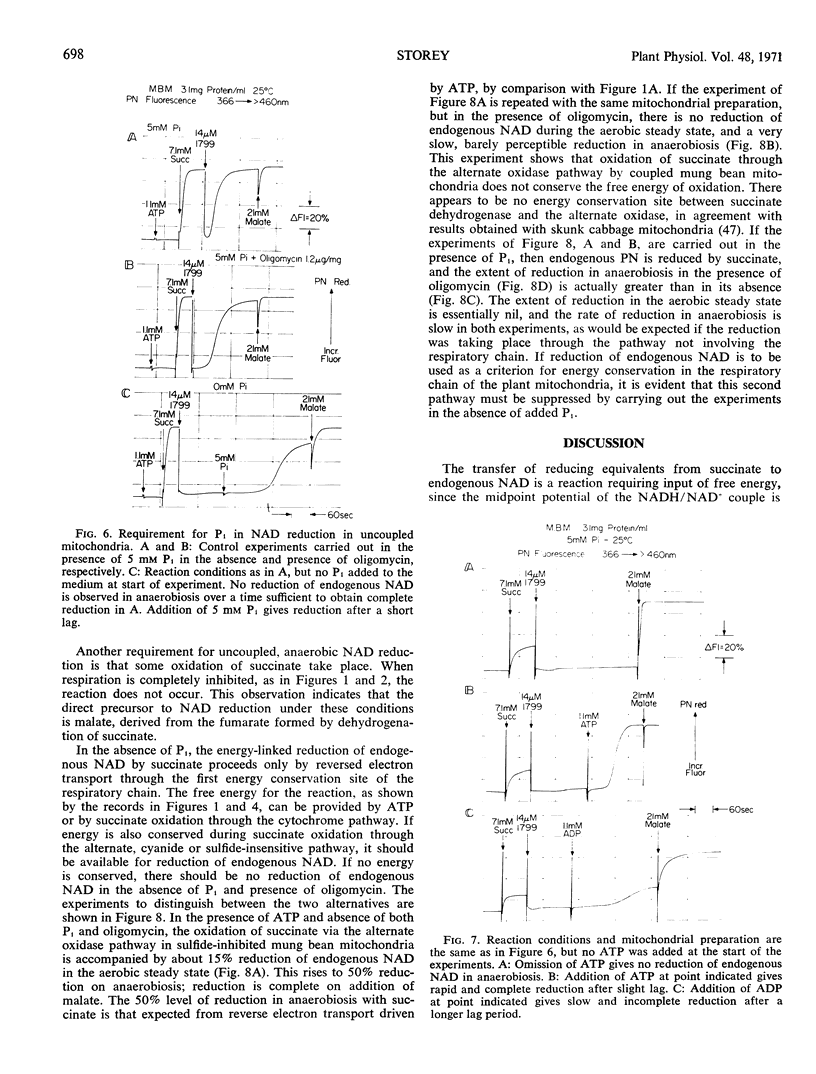
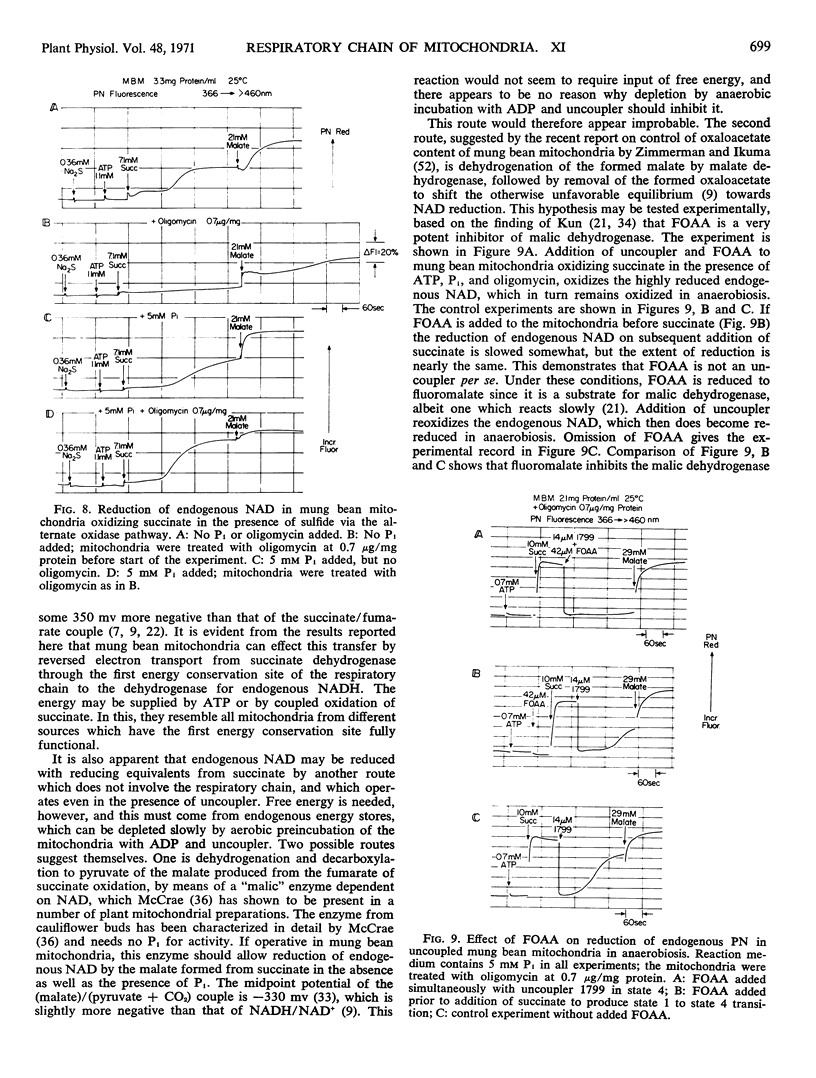
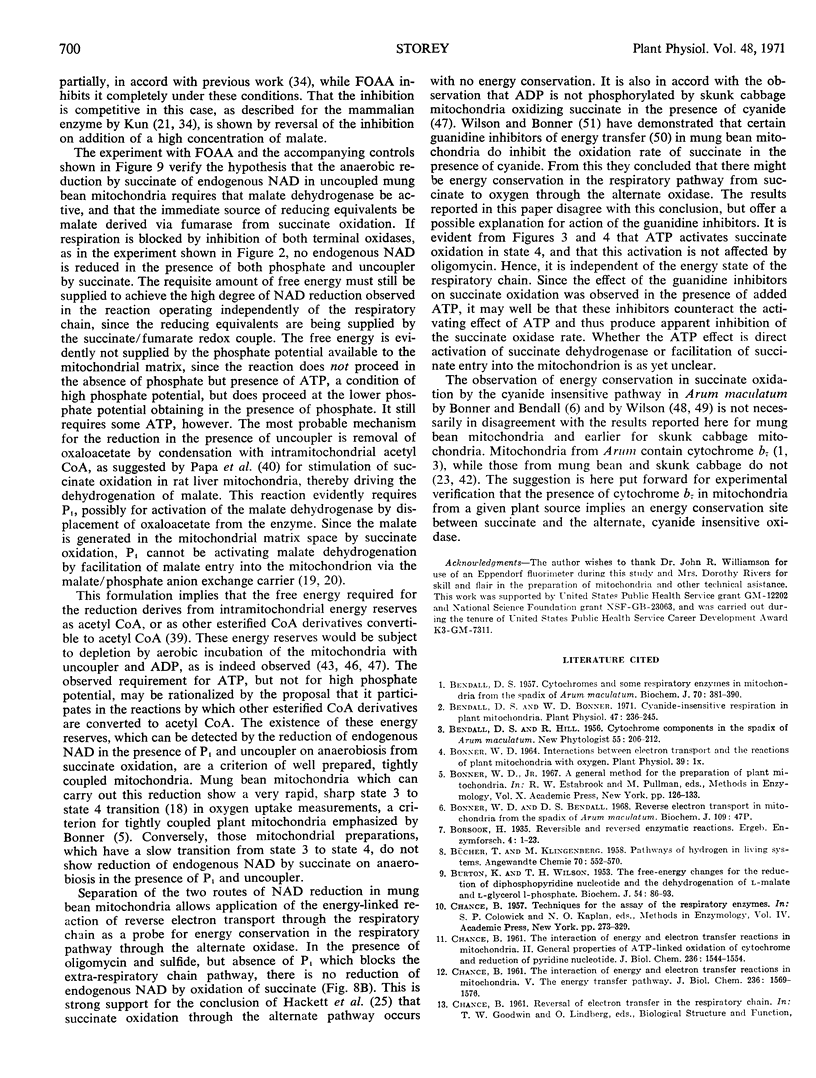
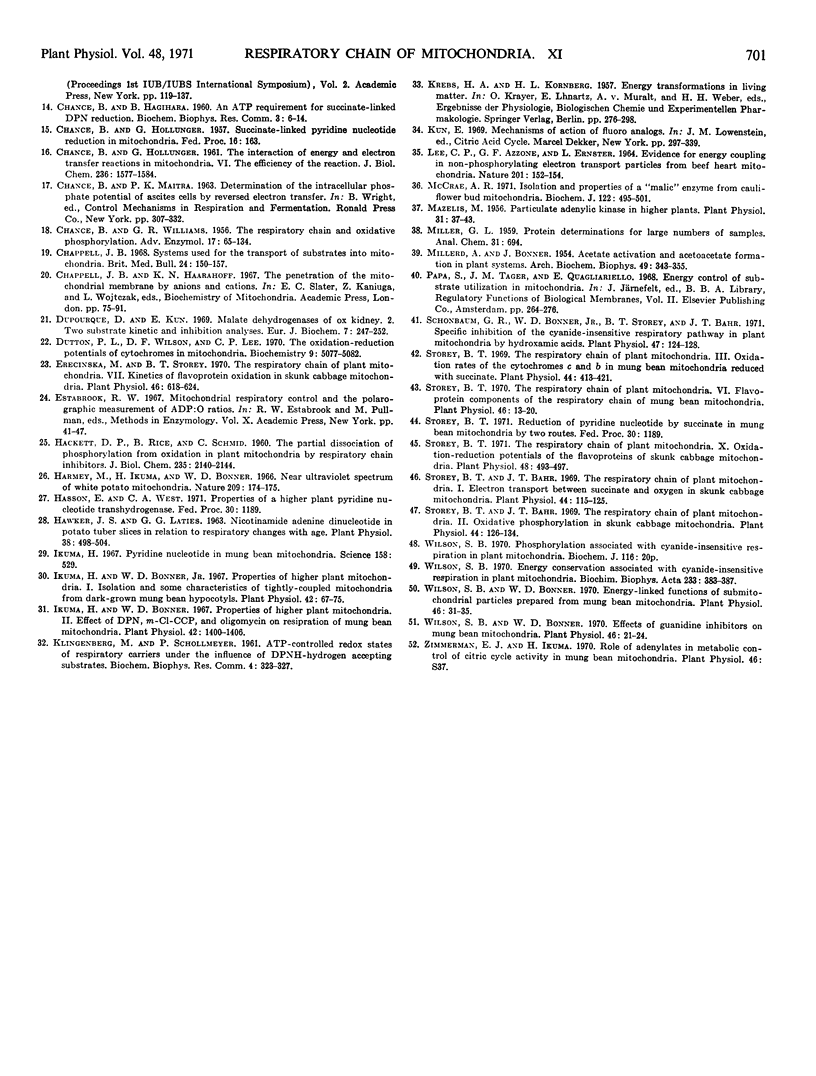
Selected References
These references are in PubMed. This may not be the complete list of references from this article.
- BENDALL D. S. Cytochromes and some respiratory enzymes in mitochondria from the spadix of Arum maculatum. Biochem J. 1958 Nov;70(3):381–390. doi: 10.1042/bj0700381. [DOI] [PMC free article] [PubMed] [Google Scholar]
- BURTON K., WILSON T. H. The free-energy changes for the reduction of diphosphopyridine nucleotide and the dehydrogenation of L-malate and L-glycerol 1-phosphate. Biochem J. 1953 Apr;54(1):86–94. doi: 10.1042/bj0540086. [DOI] [PMC free article] [PubMed] [Google Scholar]
- Bendall D. S., Bonner W. D. Cyanide-insensitive Respiration in Plant Mitochondria. Plant Physiol. 1971 Feb;47(2):236–245. doi: 10.1104/pp.47.2.236. [DOI] [PMC free article] [PubMed] [Google Scholar]
- Bonner W. D., Bendall D. S. Reversed electron transport in mitochondria from the spadix of Arum maculatum. Biochem J. 1968 Sep;109(3):47P–47P. doi: 10.1042/bj1090047pa. [DOI] [PMC free article] [PubMed] [Google Scholar]
- CHANCE B., HOLLUNGER G. The interaction of energy and electron transfer reactions in mitochondria. VI. The efficiency of the reaction. J Biol Chem. 1961 May;236:1577–1584. [PubMed] [Google Scholar]
- CHANCE B. The interaction of energy and electron transfer reactions in mitochondria. II. General properties of adenosine triphosphate-linked oxidation of cytochrome and reduction of pyridine nucleotide. J Biol Chem. 1961 May;236:1544–1554. [PubMed] [Google Scholar]
- CHANCE B. The interaction of energy and electron transfer reactions in mitochondria. V. The energy transfer pathway. J Biol Chem. 1961 May;236:1569–1576. [PubMed] [Google Scholar]
- CHANCE B., WILLIAMS G. R. The respiratory chain and oxidative phosphorylation. Adv Enzymol Relat Subj Biochem. 1956;17:65–134. doi: 10.1002/9780470122624.ch2. [DOI] [PubMed] [Google Scholar]
- Chappell J. B. Systems used for the transport of substrates into mitochondria. Br Med Bull. 1968 May;24(2):150–157. doi: 10.1093/oxfordjournals.bmb.a070618. [DOI] [PubMed] [Google Scholar]
- Deverall B. J., Daly J. M. Metabolism of Indoleacetic Acid in Rust Diseases. II. Metabolites of Carboxyl-labeled Indoleacetic Acid in Tissues. Plant Physiol. 1964 Jan;39(1):1–9. doi: 10.1104/pp.39.1.1. [DOI] [PMC free article] [PubMed] [Google Scholar]
- Dupourque D., Kun E. Malate dehydrogenases of ox kidney. 2. Two substrate kinetic and inhibition analyses. Eur J Biochem. 1969 Jan;7(2):247–252. doi: 10.1111/j.1432-1033.1969.tb19599.x. [DOI] [PubMed] [Google Scholar]
- Dutton P. L., Wilson D. F., Lee C. P. Oxidation-reduction potentials of cytochromes in mitochondria. Biochemistry. 1970 Dec 22;9(26):5077–5082. doi: 10.1021/bi00828a006. [DOI] [PubMed] [Google Scholar]
- Erecinska M., Storey B. T. The Respiratory Chain of Plant Mitochondria: VII. Kinetics of Flavoprotein Oxidation in Skunk Cabbage Mitochondria. Plant Physiol. 1970 Oct;46(4):618–624. doi: 10.1104/pp.46.4.618. [DOI] [PMC free article] [PubMed] [Google Scholar]
- HACKETT D. P., RICE B., SCHMID C. The partial dissociation of phosphorylation from oxidation in plant mitochondria by respiratory chain inhibitors. J Biol Chem. 1960 Jul;235:2140–2144. [PubMed] [Google Scholar]
- Hawker J. S., Laties G. G. Nicotinamide Adenine Dinucleotide in Potato Tuber Slices in Relation to Respiratory Changes with Age. Plant Physiol. 1963 Sep;38(5):498–500. doi: 10.1104/pp.38.5.498. [DOI] [PMC free article] [PubMed] [Google Scholar]
- Ikuma H., Bonner W. D. Properties of Higher Plant Mitochondria. I. Isolation and Some Characteristics of Tightly-coupled Mitochondria from Dark-grown Mung Bean Hypocotyls. Plant Physiol. 1967 Jan;42(1):67–75. doi: 10.1104/pp.42.1.67. [DOI] [PMC free article] [PubMed] [Google Scholar]
- Ikuma H., Bonner W. D. Properties of Higher Plant Mitochondria. II. Effects of DNP, m-Cl-CCP, and Oligomycin on Respiration of Mung Bean Mitochondria. Plant Physiol. 1967 Oct;42(10):1400–1406. doi: 10.1104/pp.42.10.1400. [DOI] [PMC free article] [PubMed] [Google Scholar]
- Ikuma H. Pyridine nucleotide in mung bean mitochondria. Science. 1967 Oct 27;158(3800):529–529. doi: 10.1126/science.158.3800.529-b. [DOI] [PubMed] [Google Scholar]
- KLINGENBERG M., SCHOLLMEYER P. ATP controlled redox states of respiratory carriers under the influence of DPNH-hydrogen accepting substrates. Biochem Biophys Res Commun. 1961 Apr 7;4:323–327. doi: 10.1016/0006-291x(61)90211-x. [DOI] [PubMed] [Google Scholar]
- LEE C. P., AZZONE G. F., ERNSTER L. EVIDENCE FOR ENERGY-COUPLING IN NON-PHOSPHORYLATING ELECTRON TRANSPORT PARTICLES FROM BEEF-HEART MITOCHONDRIA. Nature. 1964 Jan 11;201:152–155. doi: 10.1038/201152a0. [DOI] [PubMed] [Google Scholar]
- MILLERD A., BONNER J. Acetate activation and acetoacetate formation in plant systems. Arch Biochem Biophys. 1954 Apr;49(2):343–355. doi: 10.1016/0003-9861(54)90204-0. [DOI] [PubMed] [Google Scholar]
- Mazelis M. Particulate Adenylic Kinase in Higher Plants. Plant Physiol. 1956 Jan;31(1):37–43. doi: 10.1104/pp.31.1.37. [DOI] [PMC free article] [PubMed] [Google Scholar]
- Schonbaum G. R., Bonner W. D., Jr, Storey B. T., Bahr J. T. Specific inhibition of the cyanide-insensitive respiratory pathway in plant mitochondria by hydroxamic acids. Plant Physiol. 1971 Jan;47(1):124–128. doi: 10.1104/pp.47.1.124. [DOI] [PMC free article] [PubMed] [Google Scholar]
- Storey B. T., Bahr J. T. The respiratory chain of plant mitochondria. I. Electron transport between succinate and oxygen in skunk cabbage mitochondria. Plant Physiol. 1969 Jan;44(1):115–125. doi: 10.1104/pp.44.1.115. [DOI] [PMC free article] [PubMed] [Google Scholar]
- Storey B. T., Bahr J. T. The respiratory chain of plant mitochondria. II. Oxidative phosphorylation in skunk cabbage mitochondria. Plant Physiol. 1969 Jan;44(1):126–134. doi: 10.1104/pp.44.1.126. [DOI] [PMC free article] [PubMed] [Google Scholar]
- Storey B. T. The Respiratory Chain of Plant Mitochondria. III. Oxidation Rates of the Cytochromes c and b in Mung Bean Mitochondria Reduced With Succinate. Plant Physiol. 1969 Mar;44(3):413–421. doi: 10.1104/pp.44.3.413. [DOI] [PMC free article] [PubMed] [Google Scholar]
- Storey B. T. The Respiratory Chain of Plant Mitochondria: VI. Flavoprotein Components of the Respiratory Chain of Mung Bean Mitochondria. Plant Physiol. 1970 Jul;46(1):13–20. doi: 10.1104/pp.46.1.13. [DOI] [PMC free article] [PubMed] [Google Scholar]
- Storey B. T. The respiratory chain of plant mitochondria: x. Oxidation-reduction potentials of the flavoproteins of skunk cabbage mitochondria. Plant Physiol. 1971 Oct;48(4):493–497. doi: 10.1104/pp.48.4.493. [DOI] [PMC free article] [PubMed] [Google Scholar]
- Wilson S. B., Bonner W. D. Effects of guanidine inhibitors on mung bean mitochondria. Plant Physiol. 1970 Jul;46(1):21–24. doi: 10.1104/pp.46.1.21. [DOI] [PMC free article] [PubMed] [Google Scholar]
- Wilson S. B., Bonner W. D. Energy-linked Functions of Submitochondrial Particles Prepared from Mung Bean Mitochondria. Plant Physiol. 1970 Jul;46(1):31–35. doi: 10.1104/pp.46.1.31. [DOI] [PMC free article] [PubMed] [Google Scholar]
- Wilson S. B. Energy conservation associated with cyanide-insensitive respiration in plant mitochondria. Biochim Biophys Acta. 1970 Dec 8;223(2):383–387. doi: 10.1016/0005-2728(70)90195-7. [DOI] [PubMed] [Google Scholar]