Abstract
The effect of temperature from 5 degrees C to 50 degrees C on the retention of dansyl derivatives of amino acids in hydrophobic interaction chromatography (HIC) was investigated by HPLC on three stationary phases. Plots of the logarithmic retention factor against the reciprocal temperature in a wide range were nonlinear, indicative of a large negative heat capacity change associated with retention. By using Kirchoff's relations, the enthalpy, entropy, and heat capacity changes were evaluated from the logarithmic retention factor at various temperatures by fitting the data to a logarithmic equation and a quadratic equation that are based on the invariance and on an inverse square dependence of the heat capacity on temperature, respectively. In the experimental temperature interval, the heat capacity change was found to increase with temperature and could be approximated by the arithmetic average. For HIC retention of a set of dansylamino acids, both enthalpy and entropy changes were positive at low temperatures but negative at high temperatures as described in the literature for other processes based on the hydrophobic effect. The approach presented here shows that chromatographic measurements can be not only a useful adjunct to calorimetry but also an alternative means for the evaluation of thermodynamic parameters.
Full text
PDF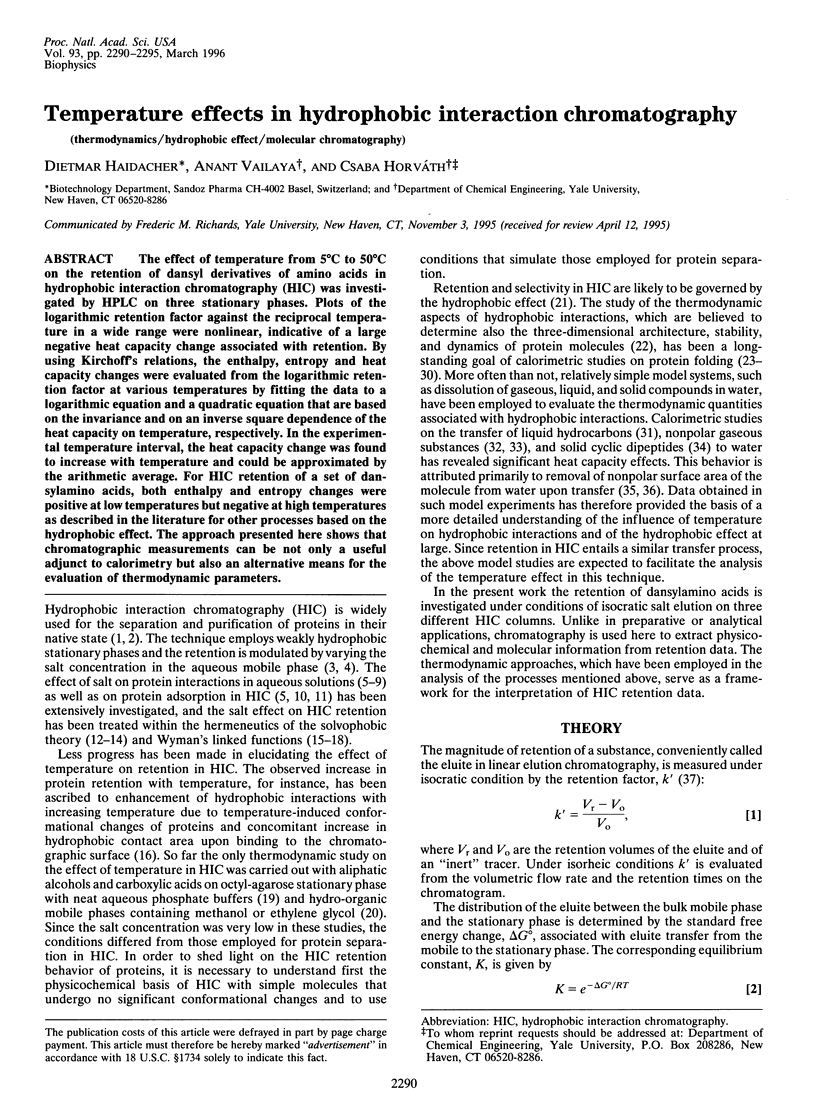
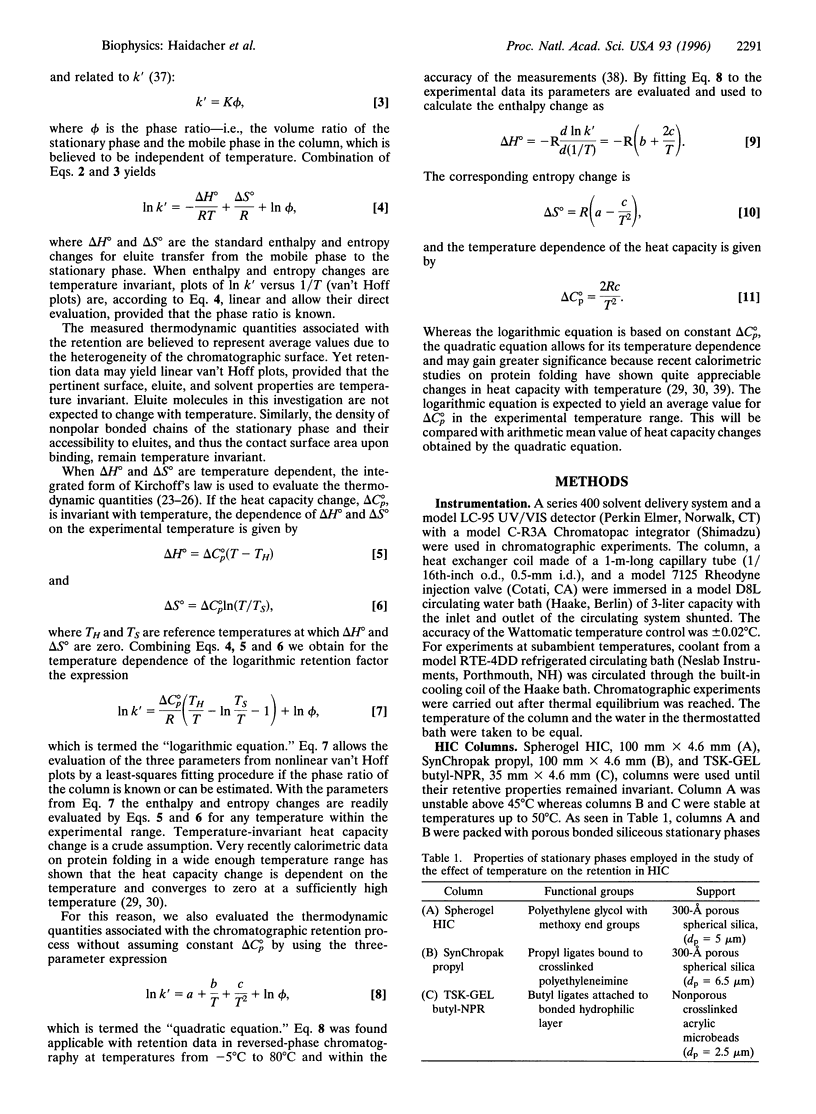
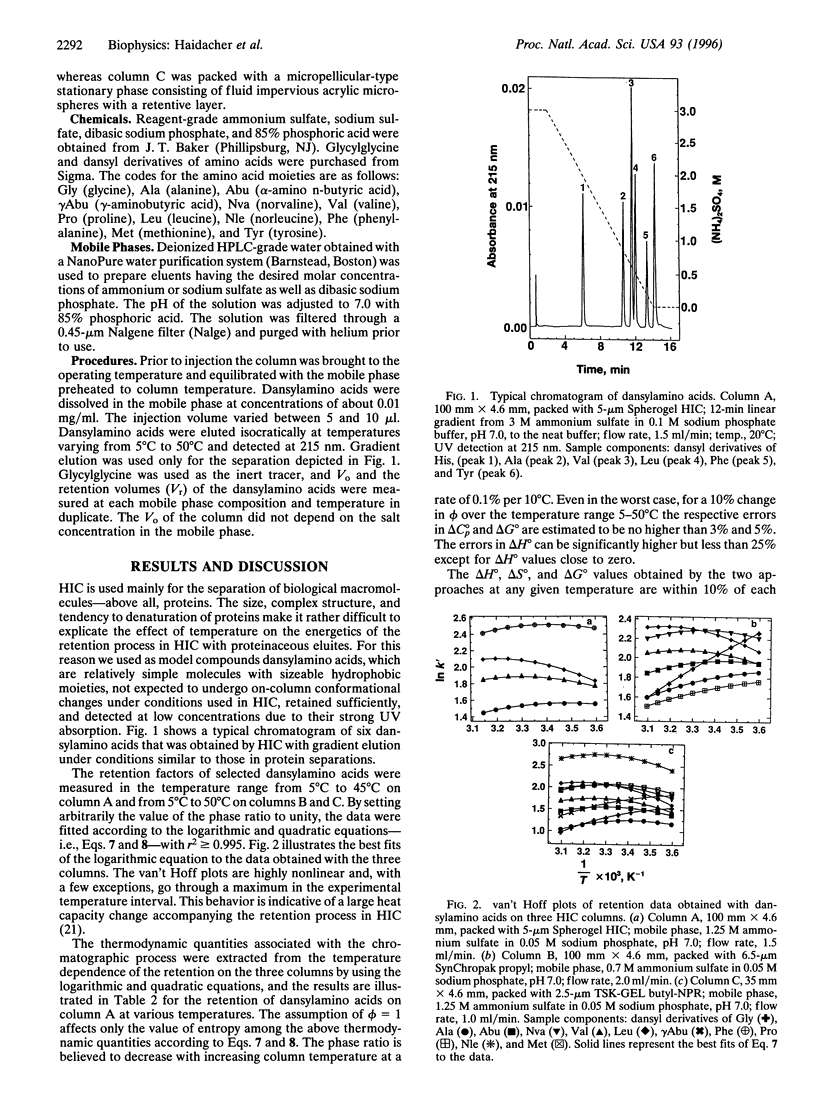
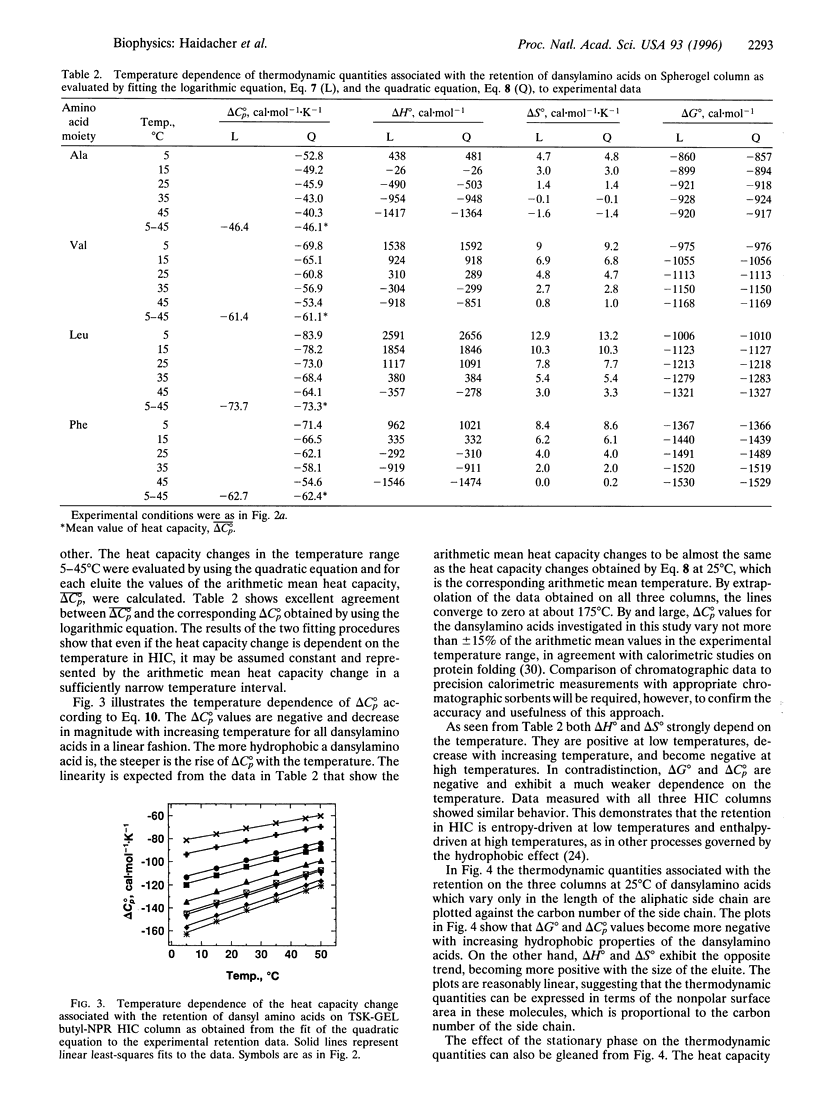
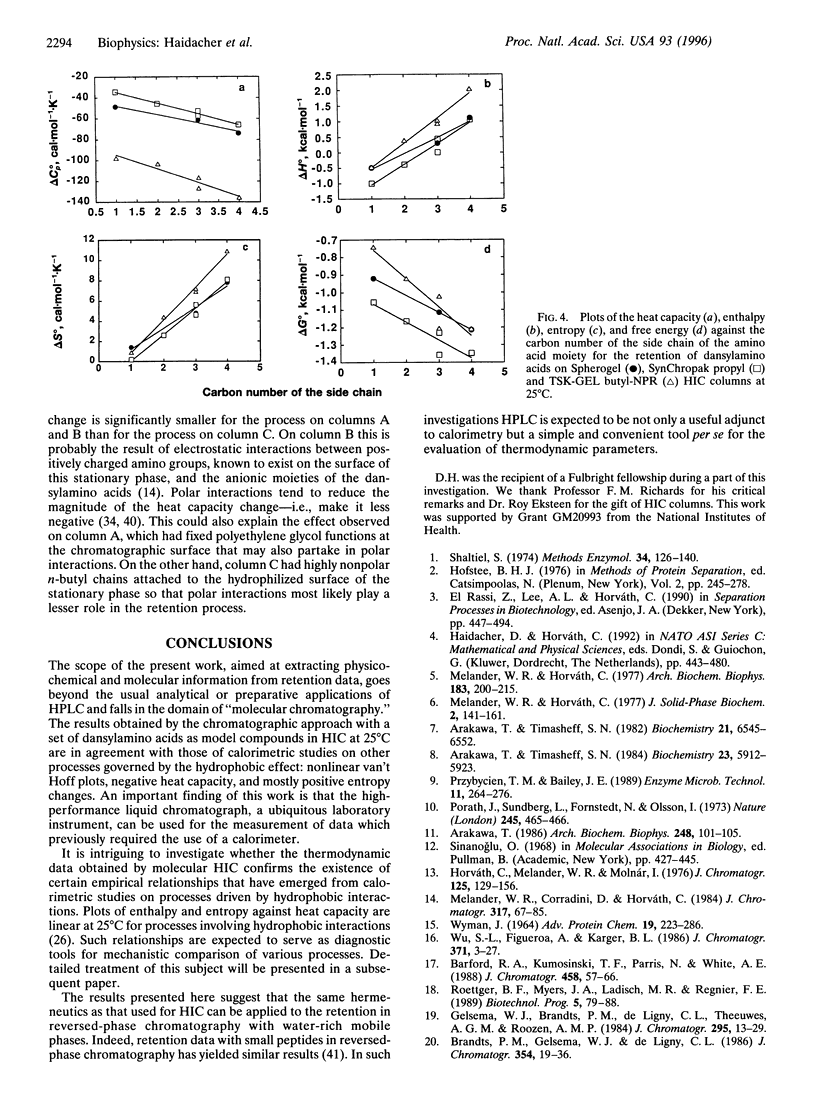
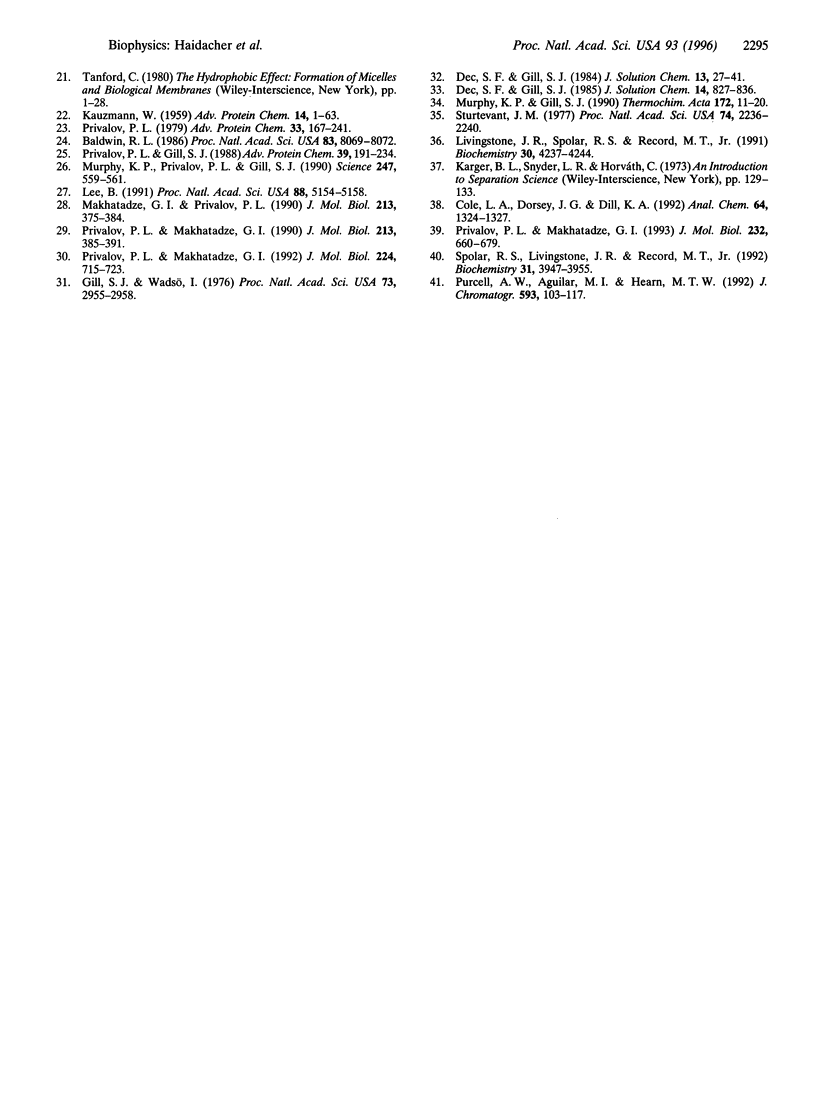
Selected References
These references are in PubMed. This may not be the complete list of references from this article.
- Arakawa T. Thermodynamic analysis of the effect of concentrated salts on protein interaction with hydrophobic and polysaccharide columns. Arch Biochem Biophys. 1986 Jul;248(1):101–105. doi: 10.1016/0003-9861(86)90405-4. [DOI] [PubMed] [Google Scholar]
- Arakawa T., Timasheff S. N. Mechanism of protein salting in and salting out by divalent cation salts: balance between hydration and salt binding. Biochemistry. 1984 Dec 4;23(25):5912–5923. doi: 10.1021/bi00320a004. [DOI] [PubMed] [Google Scholar]
- Arakawa T., Timasheff S. N. Preferential interactions of proteins with salts in concentrated solutions. Biochemistry. 1982 Dec 7;21(25):6545–6552. doi: 10.1021/bi00268a034. [DOI] [PubMed] [Google Scholar]
- Baldwin R. L. Temperature dependence of the hydrophobic interaction in protein folding. Proc Natl Acad Sci U S A. 1986 Nov;83(21):8069–8072. doi: 10.1073/pnas.83.21.8069. [DOI] [PMC free article] [PubMed] [Google Scholar]
- Cole L. A., Dorsey J. G., Dill K. A. Temperature dependence of retention in reversed-phase liquid chromatography. 2. Mobile-phase considerations. Anal Chem. 1992 Jul 1;64(13):1324–1327. doi: 10.1021/ac00037a005. [DOI] [PubMed] [Google Scholar]
- Gill S. J., Wadsö I. An equation of state describing hydrophobic interactions. Proc Natl Acad Sci U S A. 1976 Sep;73(9):2955–2958. doi: 10.1073/pnas.73.9.2955. [DOI] [PMC free article] [PubMed] [Google Scholar]
- KAUZMANN W. Some factors in the interpretation of protein denaturation. Adv Protein Chem. 1959;14:1–63. doi: 10.1016/s0065-3233(08)60608-7. [DOI] [PubMed] [Google Scholar]
- Lee B. Isoenthalpic and isoentropic temperatures and the thermodynamics of protein denaturation. Proc Natl Acad Sci U S A. 1991 Jun 15;88(12):5154–5158. doi: 10.1073/pnas.88.12.5154. [DOI] [PMC free article] [PubMed] [Google Scholar]
- Livingstone J. R., Spolar R. S., Record M. T., Jr Contribution to the thermodynamics of protein folding from the reduction in water-accessible nonpolar surface area. Biochemistry. 1991 Apr 30;30(17):4237–4244. doi: 10.1021/bi00231a019. [DOI] [PubMed] [Google Scholar]
- Makhatadze G. I., Privalov P. L. Heat capacity of proteins. I. Partial molar heat capacity of individual amino acid residues in aqueous solution: hydration effect. J Mol Biol. 1990 May 20;213(2):375–384. doi: 10.1016/S0022-2836(05)80197-4. [DOI] [PubMed] [Google Scholar]
- Melander W. R., Corradini D., Horváth C. Salt-mediated retention of proteins in hydrophobic-interaction chromatography. Application of solvophobic theory. J Chromatogr. 1984 Dec 28;317:67–85. doi: 10.1016/s0021-9673(01)91648-6. [DOI] [PubMed] [Google Scholar]
- Melander W., Horváth C. Salt effect on hydrophobic interactions in precipitation and chromatography of proteins: an interpretation of the lyotropic series. Arch Biochem Biophys. 1977 Sep;183(1):200–215. doi: 10.1016/0003-9861(77)90434-9. [DOI] [PubMed] [Google Scholar]
- Murphy K. P., Privalov P. L., Gill S. J. Common features of protein unfolding and dissolution of hydrophobic compounds. Science. 1990 Feb 2;247(4942):559–561. doi: 10.1126/science.2300815. [DOI] [PubMed] [Google Scholar]
- Porath J., Sundberg L., Fornstedt N., Olsson I. Salting-out in amphiphilic gels as a new approach to hydrophobic adsorption. Nature. 1973 Oct 26;245(5426):465–466. doi: 10.1038/245465a0. [DOI] [PubMed] [Google Scholar]
- Privalov P. L., Gill S. J. Stability of protein structure and hydrophobic interaction. Adv Protein Chem. 1988;39:191–234. doi: 10.1016/s0065-3233(08)60377-0. [DOI] [PubMed] [Google Scholar]
- Privalov P. L., Makhatadze G. I. Contribution of hydration and non-covalent interactions to the heat capacity effect on protein unfolding. J Mol Biol. 1992 Apr 5;224(3):715–723. doi: 10.1016/0022-2836(92)90555-x. [DOI] [PubMed] [Google Scholar]
- Privalov P. L., Makhatadze G. I. Contribution of hydration to protein folding thermodynamics. II. The entropy and Gibbs energy of hydration. J Mol Biol. 1993 Jul 20;232(2):660–679. doi: 10.1006/jmbi.1993.1417. [DOI] [PubMed] [Google Scholar]
- Privalov P. L., Makhatadze G. I. Heat capacity of proteins. II. Partial molar heat capacity of the unfolded polypeptide chain of proteins: protein unfolding effects. J Mol Biol. 1990 May 20;213(2):385–391. doi: 10.1016/S0022-2836(05)80198-6. [DOI] [PubMed] [Google Scholar]
- Privalov P. L. Stability of proteins: small globular proteins. Adv Protein Chem. 1979;33:167–241. doi: 10.1016/s0065-3233(08)60460-x. [DOI] [PubMed] [Google Scholar]
- Purcell A. W., Aguilar M. I., Hearn M. T. High-performance liquid chromatography of amino acids, peptides and proteins. CXV. Thermodynamic behaviour of peptides in reversed-phase chromatography. J Chromatogr. 1992 Feb 28;593(1-2):103–117. doi: 10.1016/0021-9673(92)80274-x. [DOI] [PubMed] [Google Scholar]
- Shaltiel S. Hydrophobic chromatography. Methods Enzymol. 1974;34:126–140. doi: 10.1016/s0076-6879(74)34012-8. [DOI] [PubMed] [Google Scholar]
- Spolar R. S., Livingstone J. R., Record M. T., Jr Use of liquid hydrocarbon and amide transfer data to estimate contributions to thermodynamic functions of protein folding from the removal of nonpolar and polar surface from water. Biochemistry. 1992 Apr 28;31(16):3947–3955. doi: 10.1021/bi00131a009. [DOI] [PubMed] [Google Scholar]
- Sturtevant J. M. Heat capacity and entropy changes in processes involving proteins. Proc Natl Acad Sci U S A. 1977 Jun;74(6):2236–2240. doi: 10.1073/pnas.74.6.2236. [DOI] [PMC free article] [PubMed] [Google Scholar]
- WYMAN J., Jr LINKED FUNCTIONS AND RECIPROCAL EFFECTS IN HEMOGLOBIN: A SECOND LOOK. Adv Protein Chem. 1964;19:223–286. doi: 10.1016/s0065-3233(08)60190-4. [DOI] [PubMed] [Google Scholar]
- Wu S. L., Figueroa A., Karger B. L. Protein conformational effects in hydrophobic interaction chromatography. Retention characterization and the role of mobile phase additives and stationary phase hydrophobicity. J Chromatogr. 1986 Dec 26;371:3–27. doi: 10.1016/s0021-9673(01)94689-8. [DOI] [PubMed] [Google Scholar]