Abstract
All major classes of protein chaperones, including DnaK (the Hsp70 eukaryotic equivalent) and GroEL (the Hsp60 eukaryotic equivalent) have been found in Escherichia coli. Molecular chaperones enhance the yields of correctly folded polypeptides by preventing aggregation and even by disaggregating certain protein aggregates. Previously, we identified the ClpX heat-shock protein of E. coli because it enables the ClpP catalytic protease to degrade the bacteriophage lambda O replication protein. Here we report that ClpX alone possesses all the properties expected of a molecular chaperone protein. Specifically, it can protect the lambda O protein from heat-induced aggregation, disaggregate preformed lambda O aggregates, and even promote efficient binding of lambda O to its DNA recognition sequence. A lambda O-ClpX specific protein-protein interaction can be detected either by a modified ELISA assay or through the stimulation of ClpX's weak ATPase activity by lambda O. Unlike the behaviour of the major DnaK and GroEL chaperones, ClpX requires the presence of ATP or its non-hydrolysable analogue ATP-gamma-S for efficient interaction with other proteins including the protection of lambda O from aggregation. However, ClpX's ability to disaggregate lambda O aggregates requires hydrolysable ATP. We propose that the ClpX protein is a bona fide chaperone, whose biological role includes the maintenance of certain polypeptides in a form competent for proteolysis by the ClpP protease. Furthermore, our results suggest that the ClpX protein also performs typical chaperone protein functions independent of ClpP.
Full text
PDF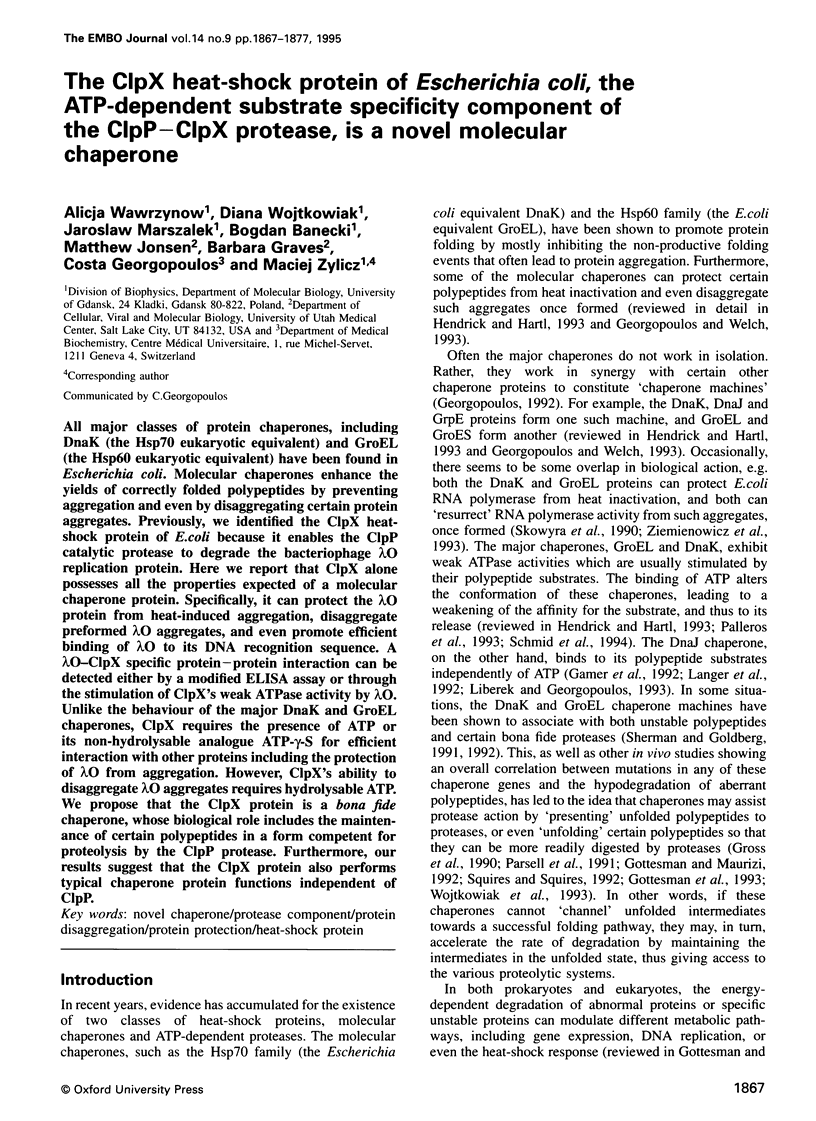
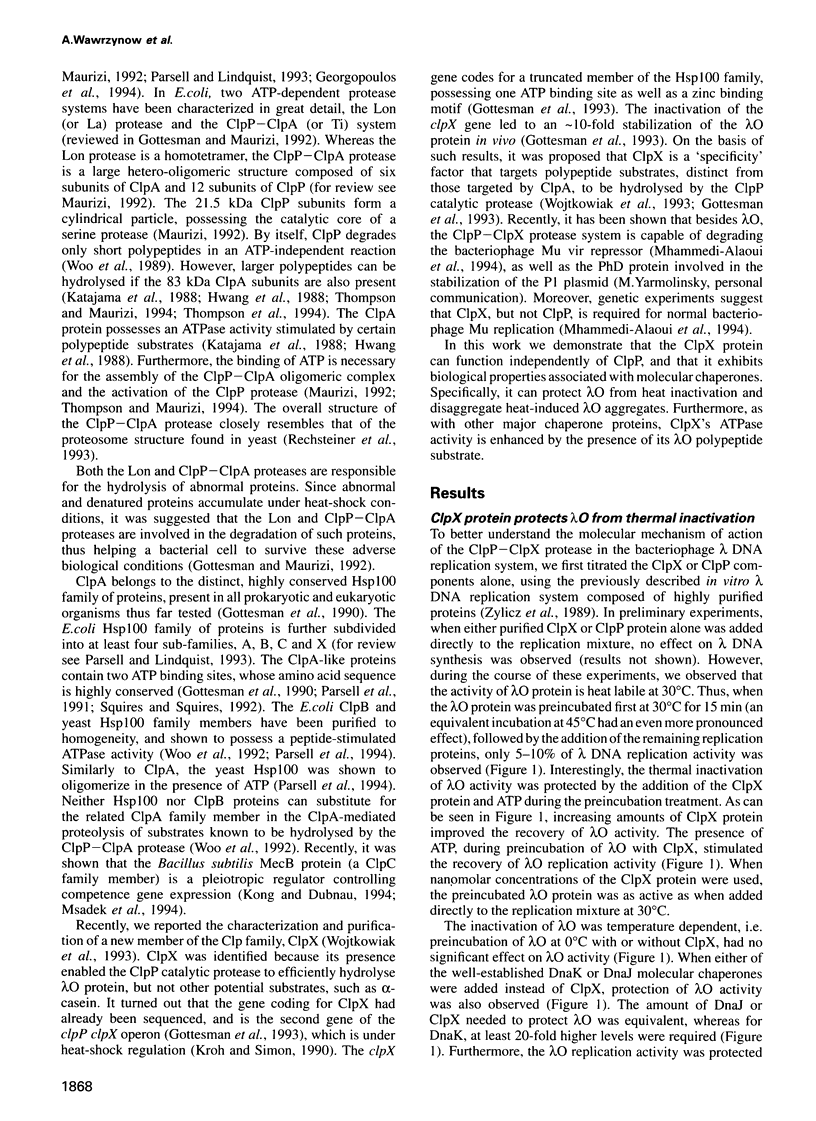
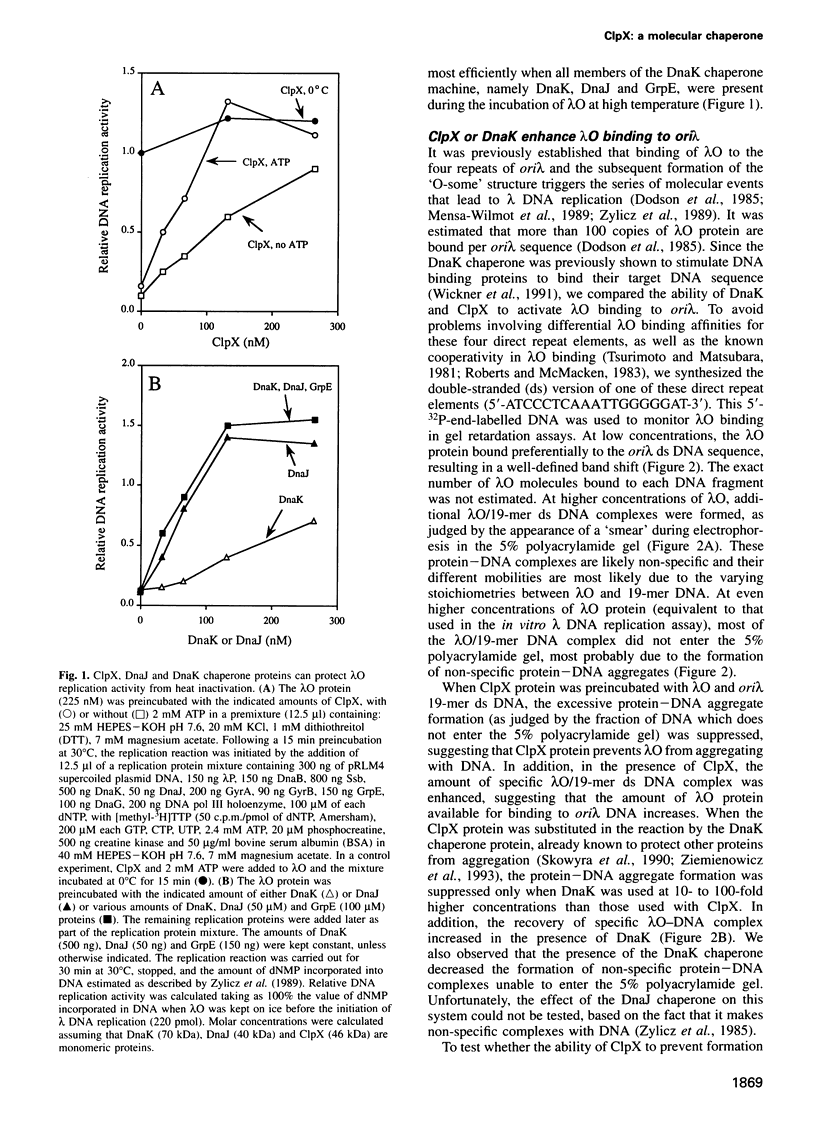
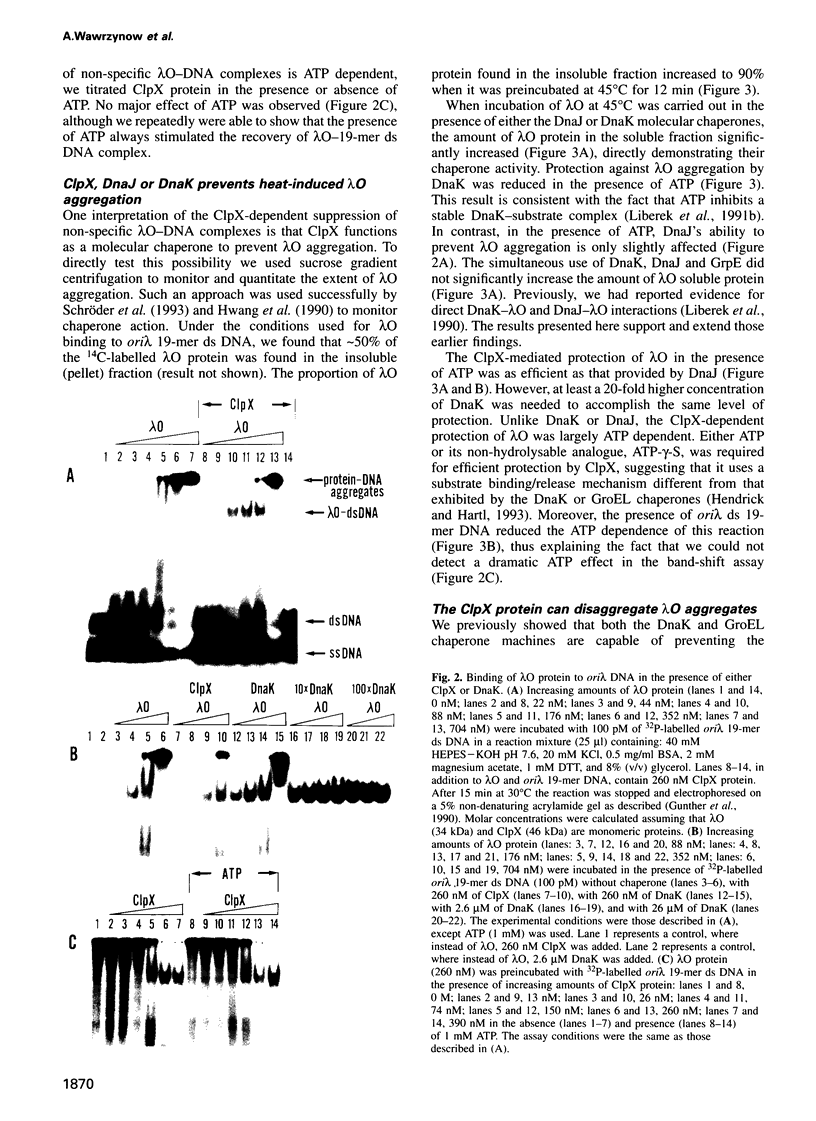
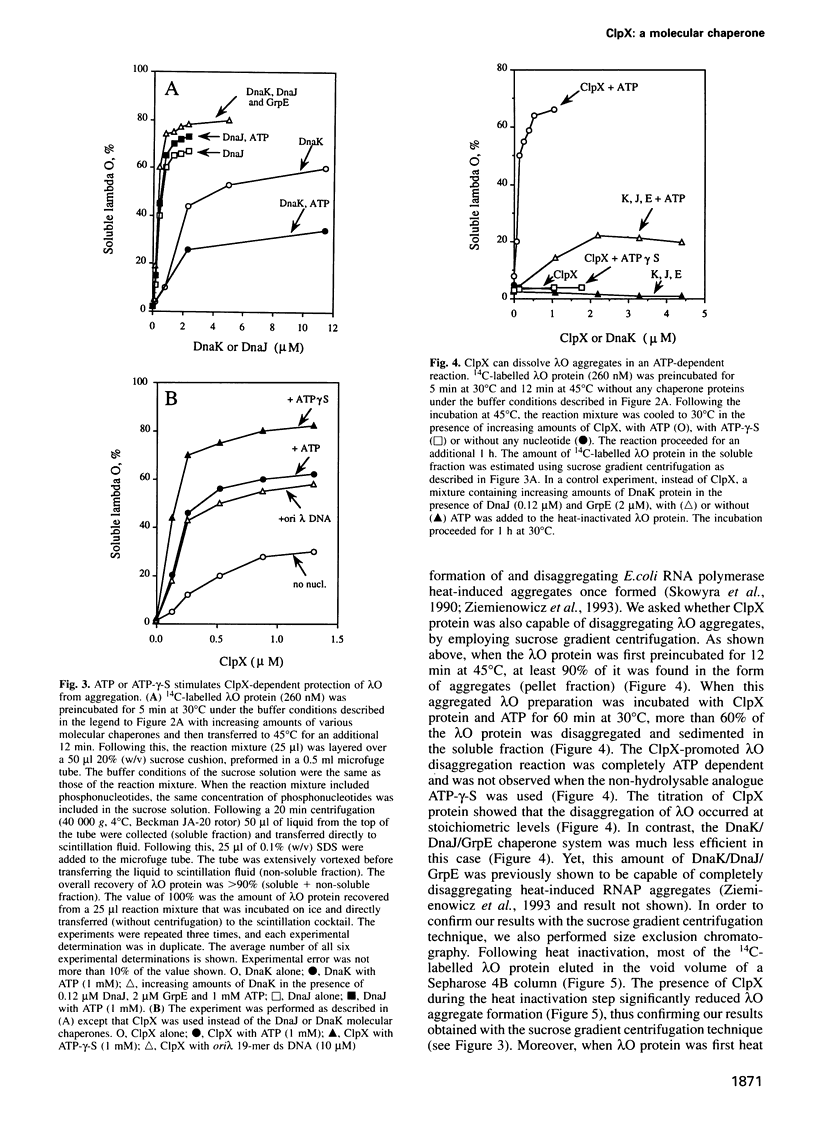
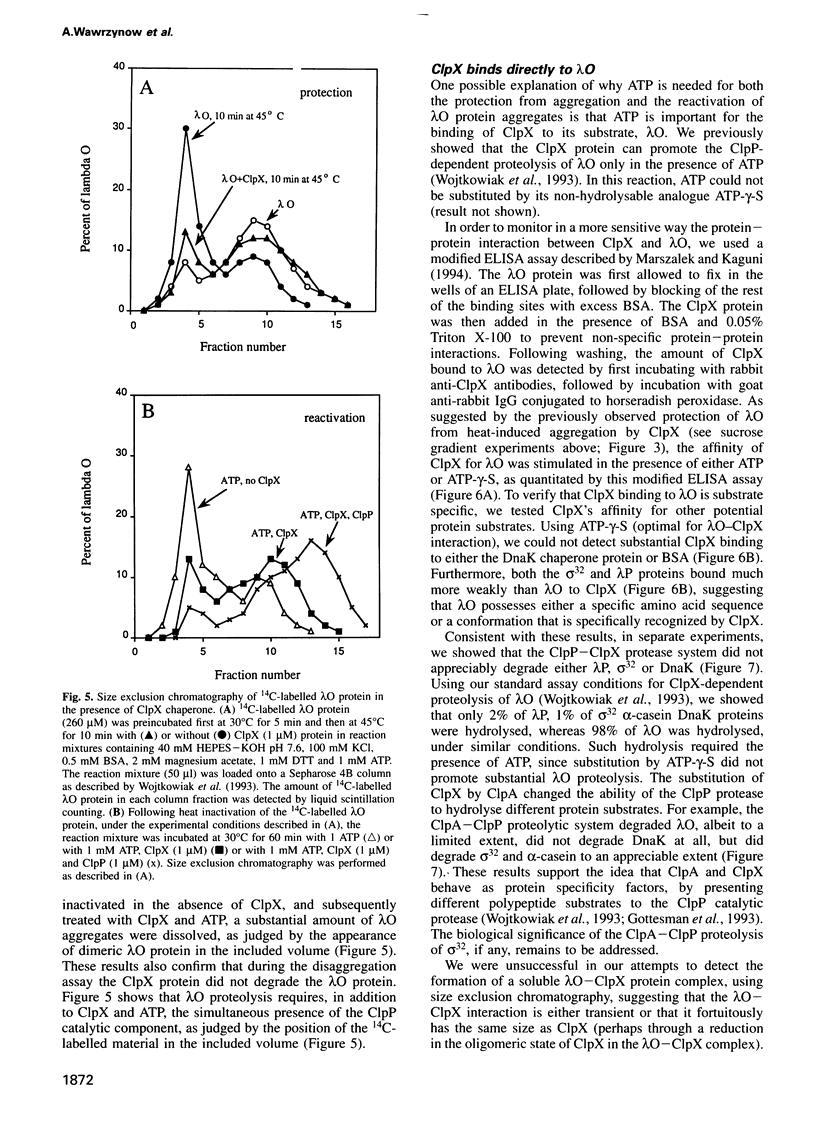
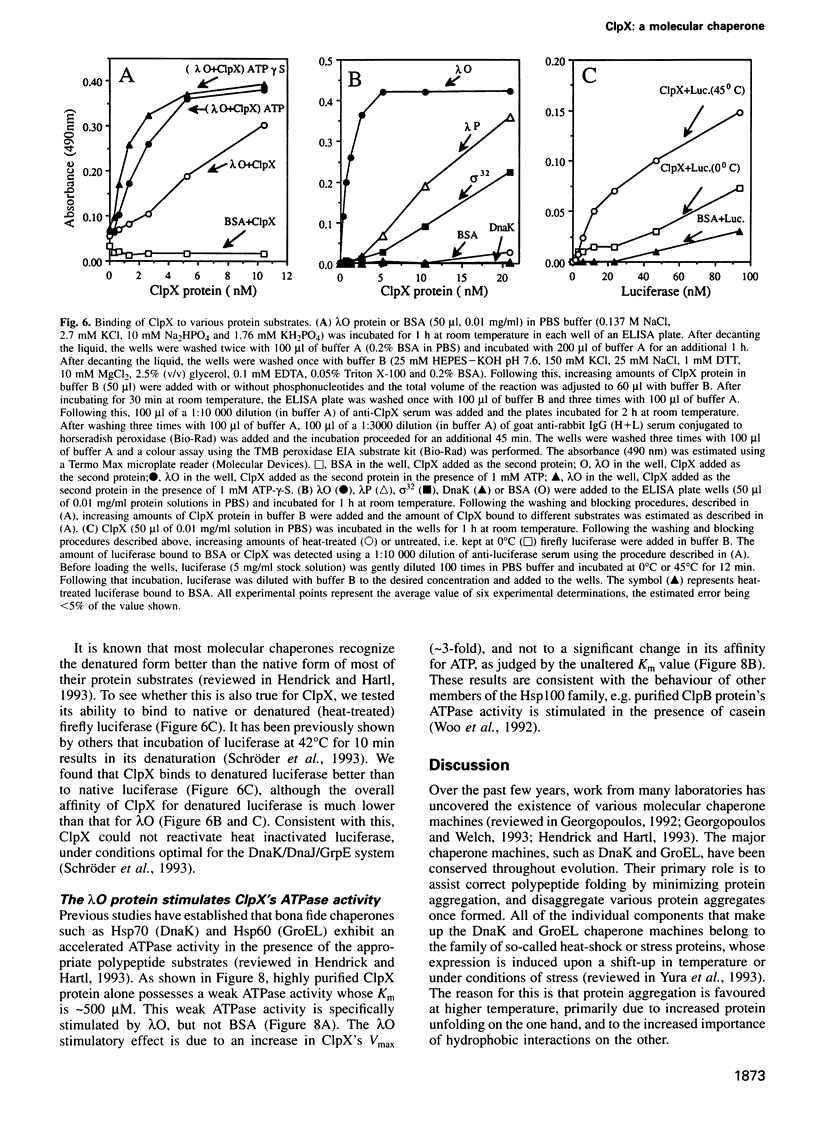
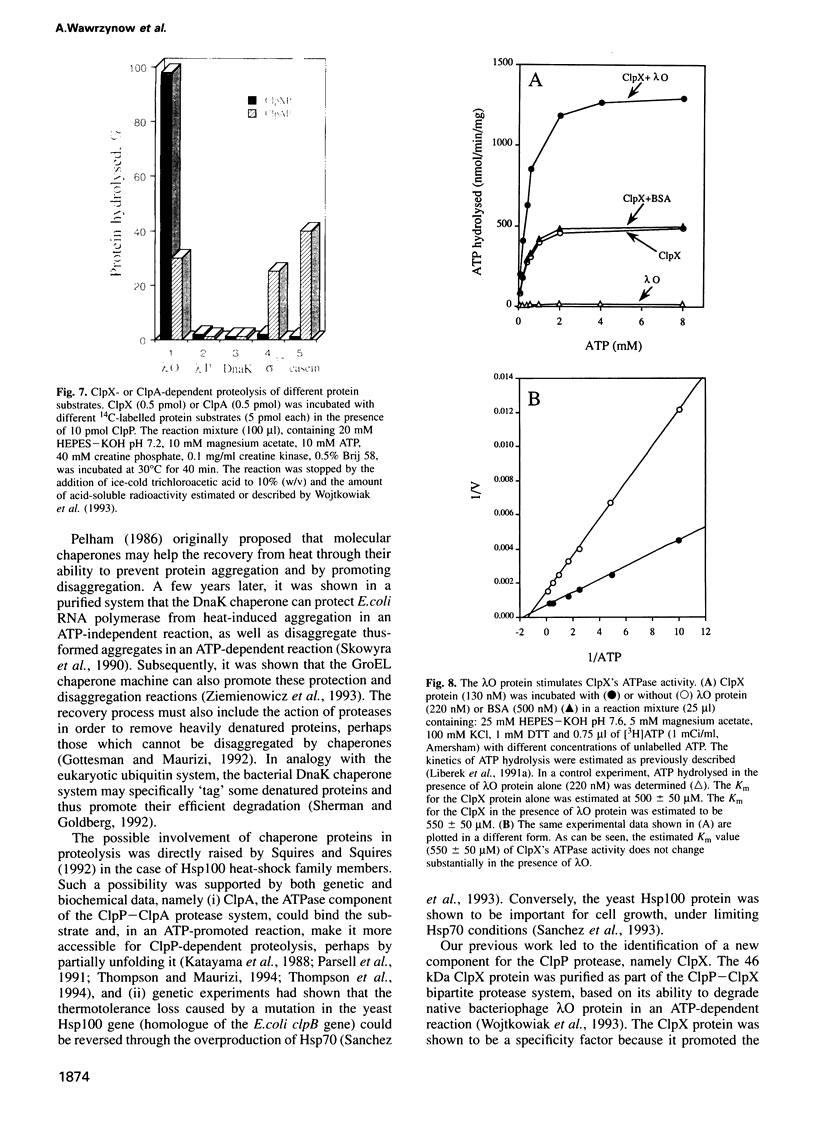
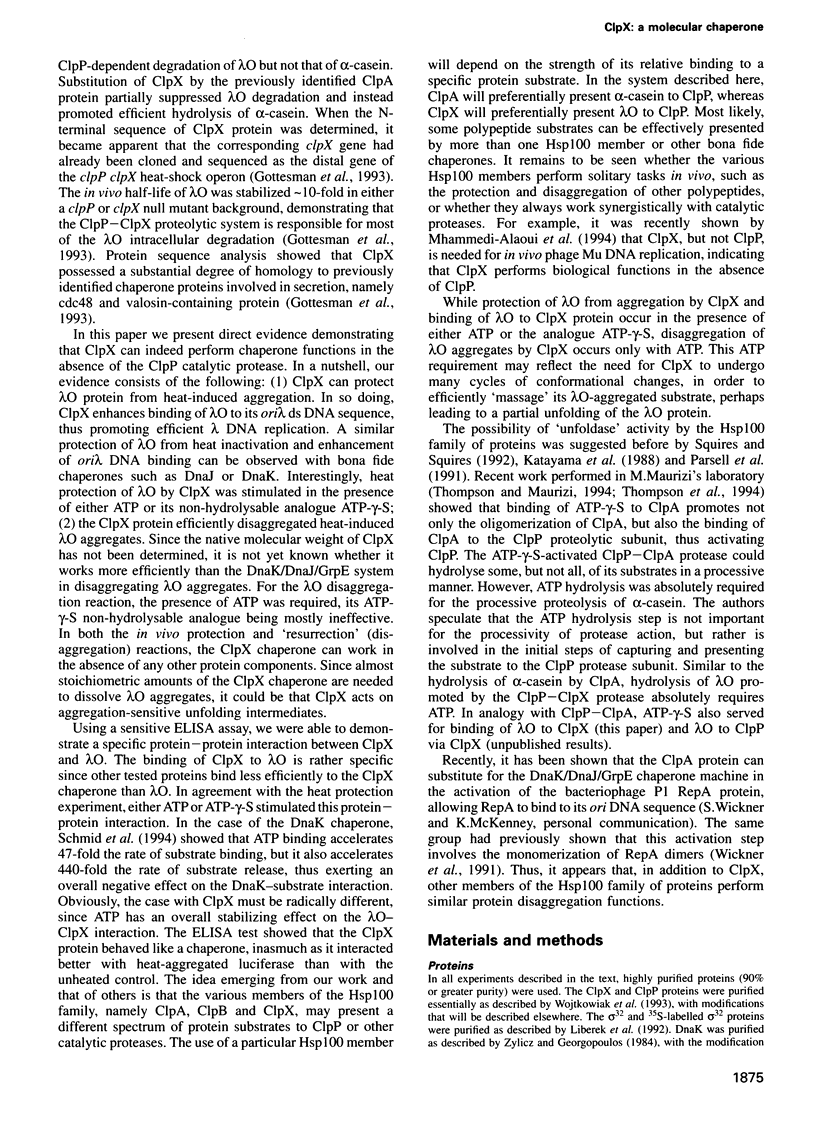
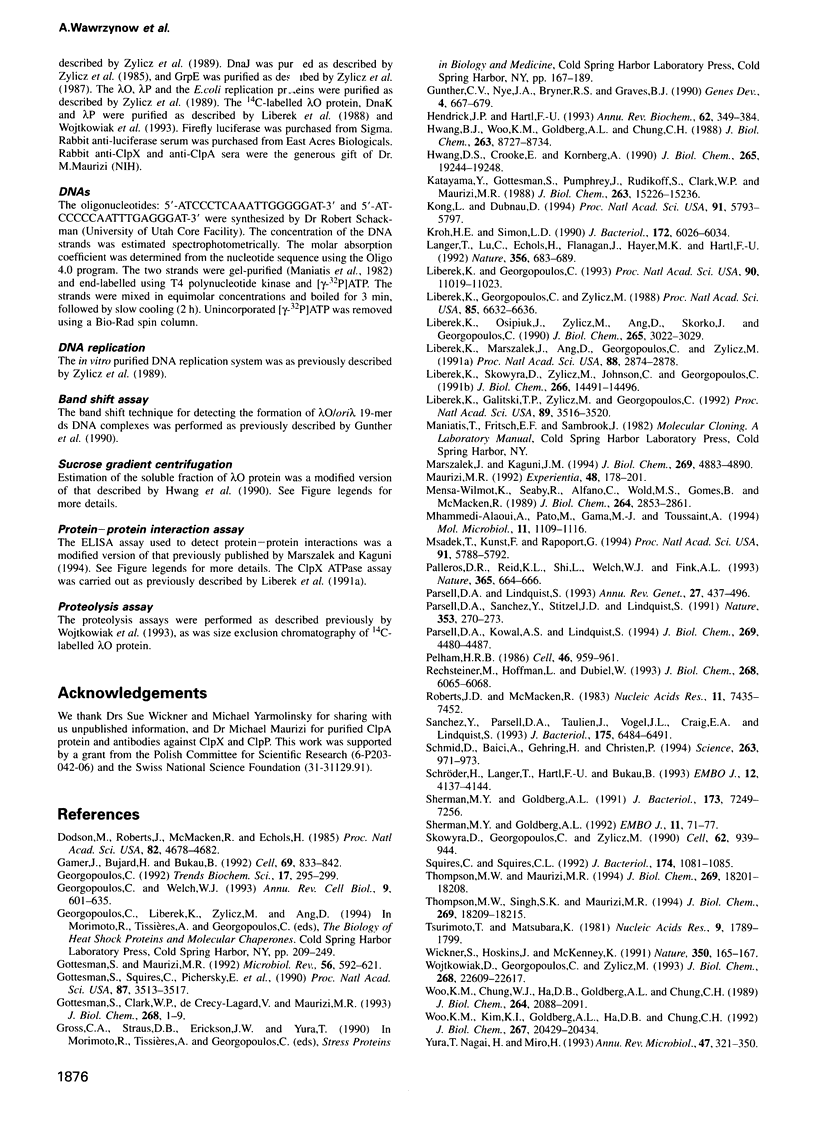
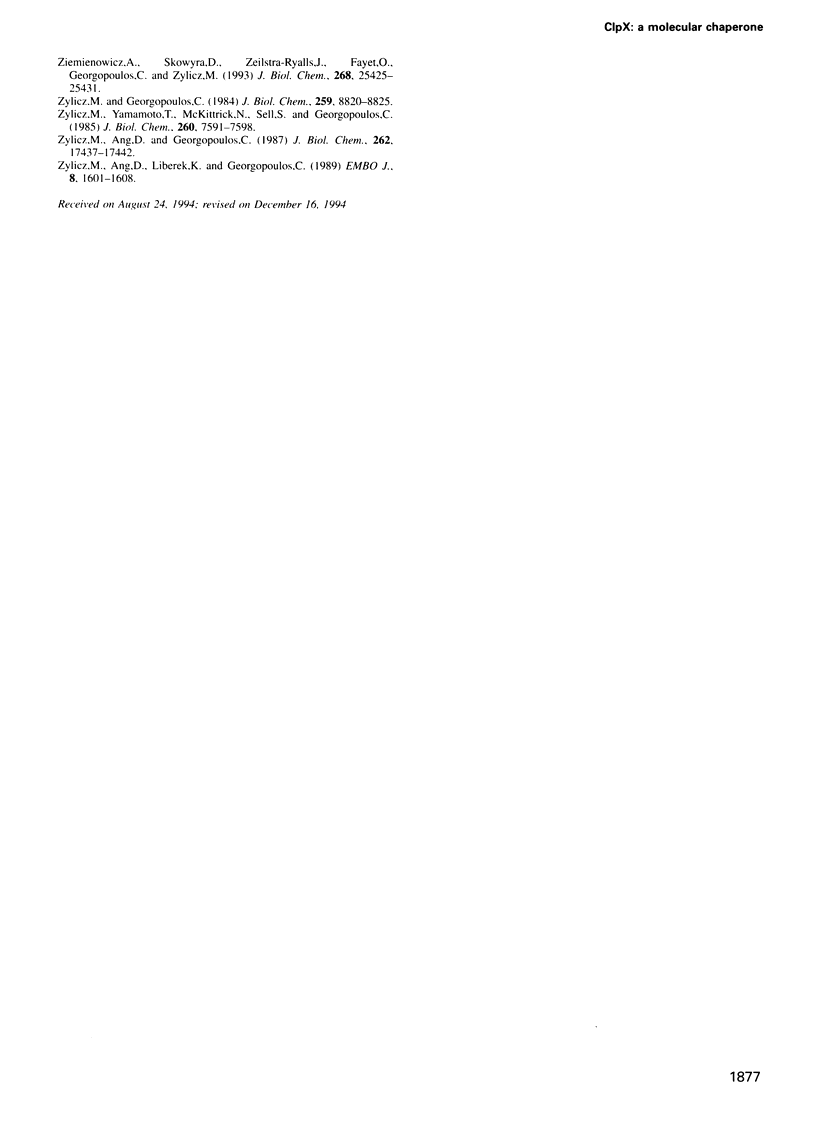
Images in this article
Selected References
These references are in PubMed. This may not be the complete list of references from this article.
- Dodson M., Roberts J., McMacken R., Echols H. Specialized nucleoprotein structures at the origin of replication of bacteriophage lambda: complexes with lambda O protein and with lambda O, lambda P, and Escherichia coli DnaB proteins. Proc Natl Acad Sci U S A. 1985 Jul;82(14):4678–4682. doi: 10.1073/pnas.82.14.4678. [DOI] [PMC free article] [PubMed] [Google Scholar]
- Gamer J., Bujard H., Bukau B. Physical interaction between heat shock proteins DnaK, DnaJ, and GrpE and the bacterial heat shock transcription factor sigma 32. Cell. 1992 May 29;69(5):833–842. doi: 10.1016/0092-8674(92)90294-m. [DOI] [PubMed] [Google Scholar]
- Georgopoulos C. The emergence of the chaperone machines. Trends Biochem Sci. 1992 Aug;17(8):295–299. doi: 10.1016/0968-0004(92)90439-g. [DOI] [PubMed] [Google Scholar]
- Georgopoulos C., Welch W. J. Role of the major heat shock proteins as molecular chaperones. Annu Rev Cell Biol. 1993;9:601–634. doi: 10.1146/annurev.cb.09.110193.003125. [DOI] [PubMed] [Google Scholar]
- Gottesman S., Maurizi M. R. Regulation by proteolysis: energy-dependent proteases and their targets. Microbiol Rev. 1992 Dec;56(4):592–621. doi: 10.1128/mr.56.4.592-621.1992. [DOI] [PMC free article] [PubMed] [Google Scholar]
- Gottesman S., Squires C., Pichersky E., Carrington M., Hobbs M., Mattick J. S., Dalrymple B., Kuramitsu H., Shiroza T., Foster T. Conservation of the regulatory subunit for the Clp ATP-dependent protease in prokaryotes and eukaryotes. Proc Natl Acad Sci U S A. 1990 May;87(9):3513–3517. doi: 10.1073/pnas.87.9.3513. [DOI] [PMC free article] [PubMed] [Google Scholar]
- Gunther C. V., Nye J. A., Bryner R. S., Graves B. J. Sequence-specific DNA binding of the proto-oncoprotein ets-1 defines a transcriptional activator sequence within the long terminal repeat of the Moloney murine sarcoma virus. Genes Dev. 1990 Apr;4(4):667–679. doi: 10.1101/gad.4.4.667. [DOI] [PubMed] [Google Scholar]
- Hendrick J. P., Hartl F. U. Molecular chaperone functions of heat-shock proteins. Annu Rev Biochem. 1993;62:349–384. doi: 10.1146/annurev.bi.62.070193.002025. [DOI] [PubMed] [Google Scholar]
- Hwang B. J., Woo K. M., Goldberg A. L., Chung C. H. Protease Ti, a new ATP-dependent protease in Escherichia coli, contains protein-activated ATPase and proteolytic functions in distinct subunits. J Biol Chem. 1988 Jun 25;263(18):8727–8734. [PubMed] [Google Scholar]
- Hwang D. S., Crooke E., Kornberg A. Aggregated dnaA protein is dissociated and activated for DNA replication by phospholipase or dnaK protein. J Biol Chem. 1990 Nov 5;265(31):19244–19248. [PubMed] [Google Scholar]
- Katayama Y., Gottesman S., Pumphrey J., Rudikoff S., Clark W. P., Maurizi M. R. The two-component, ATP-dependent Clp protease of Escherichia coli. Purification, cloning, and mutational analysis of the ATP-binding component. J Biol Chem. 1988 Oct 15;263(29):15226–15236. [PubMed] [Google Scholar]
- Kong L., Dubnau D. Regulation of competence-specific gene expression by Mec-mediated protein-protein interaction in Bacillus subtilis. Proc Natl Acad Sci U S A. 1994 Jun 21;91(13):5793–5797. doi: 10.1073/pnas.91.13.5793. [DOI] [PMC free article] [PubMed] [Google Scholar]
- Kroh H. E., Simon L. D. The ClpP component of Clp protease is the sigma 32-dependent heat shock protein F21.5. J Bacteriol. 1990 Oct;172(10):6026–6034. doi: 10.1128/jb.172.10.6026-6034.1990. [DOI] [PMC free article] [PubMed] [Google Scholar]
- Langer T., Lu C., Echols H., Flanagan J., Hayer M. K., Hartl F. U. Successive action of DnaK, DnaJ and GroEL along the pathway of chaperone-mediated protein folding. Nature. 1992 Apr 23;356(6371):683–689. doi: 10.1038/356683a0. [DOI] [PubMed] [Google Scholar]
- Liberek K., Galitski T. P., Zylicz M., Georgopoulos C. The DnaK chaperone modulates the heat shock response of Escherichia coli by binding to the sigma 32 transcription factor. Proc Natl Acad Sci U S A. 1992 Apr 15;89(8):3516–3520. doi: 10.1073/pnas.89.8.3516. [DOI] [PMC free article] [PubMed] [Google Scholar]
- Liberek K., Georgopoulos C. Autoregulation of the Escherichia coli heat shock response by the DnaK and DnaJ heat shock proteins. Proc Natl Acad Sci U S A. 1993 Dec 1;90(23):11019–11023. doi: 10.1073/pnas.90.23.11019. [DOI] [PMC free article] [PubMed] [Google Scholar]
- Liberek K., Georgopoulos C., Zylicz M. Role of the Escherichia coli DnaK and DnaJ heat shock proteins in the initiation of bacteriophage lambda DNA replication. Proc Natl Acad Sci U S A. 1988 Sep;85(18):6632–6636. doi: 10.1073/pnas.85.18.6632. [DOI] [PMC free article] [PubMed] [Google Scholar]
- Liberek K., Marszalek J., Ang D., Georgopoulos C., Zylicz M. Escherichia coli DnaJ and GrpE heat shock proteins jointly stimulate ATPase activity of DnaK. Proc Natl Acad Sci U S A. 1991 Apr 1;88(7):2874–2878. doi: 10.1073/pnas.88.7.2874. [DOI] [PMC free article] [PubMed] [Google Scholar]
- Liberek K., Osipiuk J., Zylicz M., Ang D., Skorko J., Georgopoulos C. Physical interactions between bacteriophage and Escherichia coli proteins required for initiation of lambda DNA replication. J Biol Chem. 1990 Feb 25;265(6):3022–3029. [PubMed] [Google Scholar]
- Liberek K., Skowyra D., Zylicz M., Johnson C., Georgopoulos C. The Escherichia coli DnaK chaperone, the 70-kDa heat shock protein eukaryotic equivalent, changes conformation upon ATP hydrolysis, thus triggering its dissociation from a bound target protein. J Biol Chem. 1991 Aug 5;266(22):14491–14496. [PubMed] [Google Scholar]
- Marszalek J., Kaguni J. M. DnaA protein directs the binding of DnaB protein in initiation of DNA replication in Escherichia coli. J Biol Chem. 1994 Feb 18;269(7):4883–4890. [PubMed] [Google Scholar]
- Maurizi M. R. Proteases and protein degradation in Escherichia coli. Experientia. 1992 Feb 15;48(2):178–201. doi: 10.1007/BF01923511. [DOI] [PubMed] [Google Scholar]
- Mensa-Wilmot K., Seaby R., Alfano C., Wold M. C., Gomes B., McMacken R. Reconstitution of a nine-protein system that initiates bacteriophage lambda DNA replication. J Biol Chem. 1989 Feb 15;264(5):2853–2861. [PubMed] [Google Scholar]
- Mhammedi-Alaoui A., Pato M., Gama M. J., Toussaint A. A new component of bacteriophage Mu replicative transposition machinery: the Escherichia coli ClpX protein. Mol Microbiol. 1994 Mar;11(6):1109–1116. doi: 10.1111/j.1365-2958.1994.tb00387.x. [DOI] [PubMed] [Google Scholar]
- Msadek T., Kunst F., Rapoport G. MecB of Bacillus subtilis, a member of the ClpC ATPase family, is a pleiotropic regulator controlling competence gene expression and growth at high temperature. Proc Natl Acad Sci U S A. 1994 Jun 21;91(13):5788–5792. doi: 10.1073/pnas.91.13.5788. [DOI] [PMC free article] [PubMed] [Google Scholar]
- Palleros D. R., Reid K. L., Shi L., Welch W. J., Fink A. L. ATP-induced protein-Hsp70 complex dissociation requires K+ but not ATP hydrolysis. Nature. 1993 Oct 14;365(6447):664–666. doi: 10.1038/365664a0. [DOI] [PubMed] [Google Scholar]
- Parsell D. A., Kowal A. S., Lindquist S. Saccharomyces cerevisiae Hsp104 protein. Purification and characterization of ATP-induced structural changes. J Biol Chem. 1994 Feb 11;269(6):4480–4487. [PubMed] [Google Scholar]
- Parsell D. A., Lindquist S. The function of heat-shock proteins in stress tolerance: degradation and reactivation of damaged proteins. Annu Rev Genet. 1993;27:437–496. doi: 10.1146/annurev.ge.27.120193.002253. [DOI] [PubMed] [Google Scholar]
- Parsell D. A., Sanchez Y., Stitzel J. D., Lindquist S. Hsp104 is a highly conserved protein with two essential nucleotide-binding sites. Nature. 1991 Sep 19;353(6341):270–273. doi: 10.1038/353270a0. [DOI] [PubMed] [Google Scholar]
- Pelham H. R. Speculations on the functions of the major heat shock and glucose-regulated proteins. Cell. 1986 Sep 26;46(7):959–961. doi: 10.1016/0092-8674(86)90693-8. [DOI] [PubMed] [Google Scholar]
- Rechsteiner M., Hoffman L., Dubiel W. The multicatalytic and 26 S proteases. J Biol Chem. 1993 Mar 25;268(9):6065–6068. [PubMed] [Google Scholar]
- Roberts J. D., McMacken R. The bacteriophage lambda O replication protein: isolation and characterization of the amplified initiator. Nucleic Acids Res. 1983 Nov 11;11(21):7435–7452. [PMC free article] [PubMed] [Google Scholar]
- Sanchez Y., Parsell D. A., Taulien J., Vogel J. L., Craig E. A., Lindquist S. Genetic evidence for a functional relationship between Hsp104 and Hsp70. J Bacteriol. 1993 Oct;175(20):6484–6491. doi: 10.1128/jb.175.20.6484-6491.1993. [DOI] [PMC free article] [PubMed] [Google Scholar]
- Schmid D., Baici A., Gehring H., Christen P. Kinetics of molecular chaperone action. Science. 1994 Feb 18;263(5149):971–973. doi: 10.1126/science.8310296. [DOI] [PubMed] [Google Scholar]
- Schröder H., Langer T., Hartl F. U., Bukau B. DnaK, DnaJ and GrpE form a cellular chaperone machinery capable of repairing heat-induced protein damage. EMBO J. 1993 Nov;12(11):4137–4144. doi: 10.1002/j.1460-2075.1993.tb06097.x. [DOI] [PMC free article] [PubMed] [Google Scholar]
- Sherman MYu, Goldberg A. L. Involvement of the chaperonin dnaK in the rapid degradation of a mutant protein in Escherichia coli. EMBO J. 1992 Jan;11(1):71–77. doi: 10.1002/j.1460-2075.1992.tb05029.x. [DOI] [PMC free article] [PubMed] [Google Scholar]
- Sherman M. Y., Goldberg A. L. Formation in vitro of complexes between an abnormal fusion protein and the heat shock proteins from Escherichia coli and yeast mitochondria. J Bacteriol. 1991 Nov;173(22):7249–7256. doi: 10.1128/jb.173.22.7249-7256.1991. [DOI] [PMC free article] [PubMed] [Google Scholar]
- Skowyra D., Georgopoulos C., Zylicz M. The E. coli dnaK gene product, the hsp70 homolog, can reactivate heat-inactivated RNA polymerase in an ATP hydrolysis-dependent manner. Cell. 1990 Sep 7;62(5):939–944. doi: 10.1016/0092-8674(90)90268-j. [DOI] [PubMed] [Google Scholar]
- Squires C., Squires C. L. The Clp proteins: proteolysis regulators or molecular chaperones? J Bacteriol. 1992 Feb;174(4):1081–1085. doi: 10.1128/jb.174.4.1081-1085.1992. [DOI] [PMC free article] [PubMed] [Google Scholar]
- Thompson M. W., Maurizi M. R. Activity and specificity of Escherichia coli ClpAP protease in cleaving model peptide substrates. J Biol Chem. 1994 Jul 8;269(27):18201–18208. [PubMed] [Google Scholar]
- Thompson M. W., Singh S. K., Maurizi M. R. Processive degradation of proteins by the ATP-dependent Clp protease from Escherichia coli. Requirement for the multiple array of active sites in ClpP but not ATP hydrolysis. J Biol Chem. 1994 Jul 8;269(27):18209–18215. [PubMed] [Google Scholar]
- Tsurimoto T., Matsubara K. Purified bacteriophage lambda O protein binds to four repeating sequences at the lambda replication origin. Nucleic Acids Res. 1981 Apr 24;9(8):1789–1799. doi: 10.1093/nar/9.8.1789. [DOI] [PMC free article] [PubMed] [Google Scholar]
- Wickner S., Hoskins J., McKenney K. Function of DnaJ and DnaK as chaperones in origin-specific DNA binding by RepA. Nature. 1991 Mar 14;350(6314):165–167. doi: 10.1038/350165a0. [DOI] [PubMed] [Google Scholar]
- Wojtkowiak D., Georgopoulos C., Zylicz M. Isolation and characterization of ClpX, a new ATP-dependent specificity component of the Clp protease of Escherichia coli. J Biol Chem. 1993 Oct 25;268(30):22609–22617. [PubMed] [Google Scholar]
- Woo K. M., Chung W. J., Ha D. B., Goldberg A. L., Chung C. H. Protease Ti from Escherichia coli requires ATP hydrolysis for protein breakdown but not for hydrolysis of small peptides. J Biol Chem. 1989 Feb 5;264(4):2088–2091. [PubMed] [Google Scholar]
- Woo K. M., Kim K. I., Goldberg A. L., Ha D. B., Chung C. H. The heat-shock protein ClpB in Escherichia coli is a protein-activated ATPase. J Biol Chem. 1992 Oct 5;267(28):20429–20434. [PubMed] [Google Scholar]
- Yura T., Nagai H., Mori H. Regulation of the heat-shock response in bacteria. Annu Rev Microbiol. 1993;47:321–350. doi: 10.1146/annurev.mi.47.100193.001541. [DOI] [PubMed] [Google Scholar]
- Ziemienowicz A., Skowyra D., Zeilstra-Ryalls J., Fayet O., Georgopoulos C., Zylicz M. Both the Escherichia coli chaperone systems, GroEL/GroES and DnaK/DnaJ/GrpE, can reactivate heat-treated RNA polymerase. Different mechanisms for the same activity. J Biol Chem. 1993 Dec 5;268(34):25425–25431. [PubMed] [Google Scholar]
- Zylicz M., Ang D., Georgopoulos C. The grpE protein of Escherichia coli. Purification and properties. J Biol Chem. 1987 Dec 25;262(36):17437–17442. [PubMed] [Google Scholar]
- Zylicz M., Ang D., Liberek K., Georgopoulos C. Initiation of lambda DNA replication with purified host- and bacteriophage-encoded proteins: the role of the dnaK, dnaJ and grpE heat shock proteins. EMBO J. 1989 May;8(5):1601–1608. doi: 10.1002/j.1460-2075.1989.tb03544.x. [DOI] [PMC free article] [PubMed] [Google Scholar]
- Zylicz M., Georgopoulos C. Purification and properties of the Escherichia coli dnaK replication protein. J Biol Chem. 1984 Jul 25;259(14):8820–8825. [PubMed] [Google Scholar]
- Zylicz M., Yamamoto T., McKittrick N., Sell S., Georgopoulos C. Purification and properties of the dnaJ replication protein of Escherichia coli. J Biol Chem. 1985 Jun 25;260(12):7591–7598. [PubMed] [Google Scholar]