Abstract
Small, single-module proteins that fold in a single cooperative step may be paradigms for understanding early events in protein-folding pathways generally. Recent experimental studies of the 64-residue chymotrypsin inhibitor 2 (CI2) support a nucleation mechanism for folding, as do some computer stimulations. CI2 has a nucleation site that develops only in the transition state for folding. The nucleus is composed of a set of adjacent residues (an alpha-helix), stabilized by long-range interactions that are formed as the rest of the protein collapses around it. A simple analysis of the optimization of the rate of protein folding predicts that rates are highest when the denatured state has little residual structure under physiological conditions and no intermediates accumulate. This implies that any potential nucleation site that is composed mainly of adjacent residues should be just weakly populated in the denatured state and become structured only in a high-energy intermediate or transition state when it is stabilized by interactions elsewhere in the protein. Hierarchical mechanisms of folding in which stable elements of structure accrete are unfavorable. The nucleation-condensation mechanism of CI2 fulfills the criteria for fast folding. On the other hand, stable intermediates do form in the folding of more complex proteins, and this may be an unavoidable consequence of increasing size and nucleation at more than one site.
Full text
PDF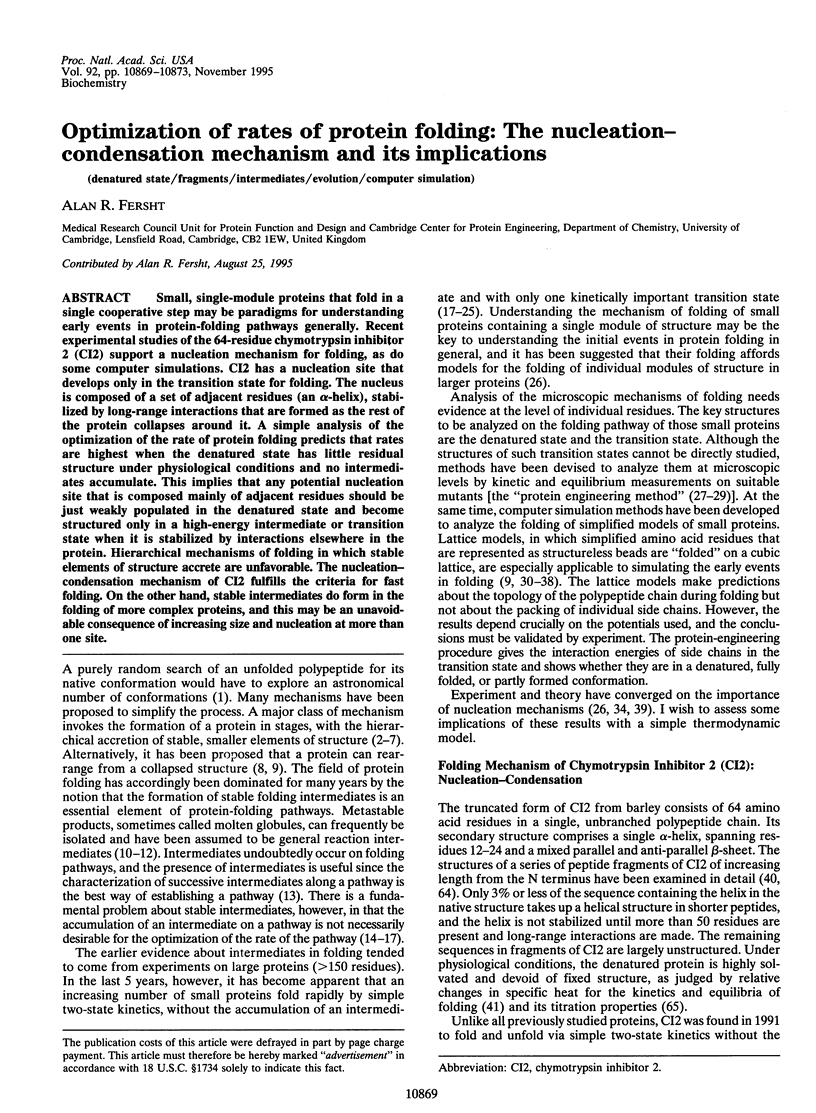
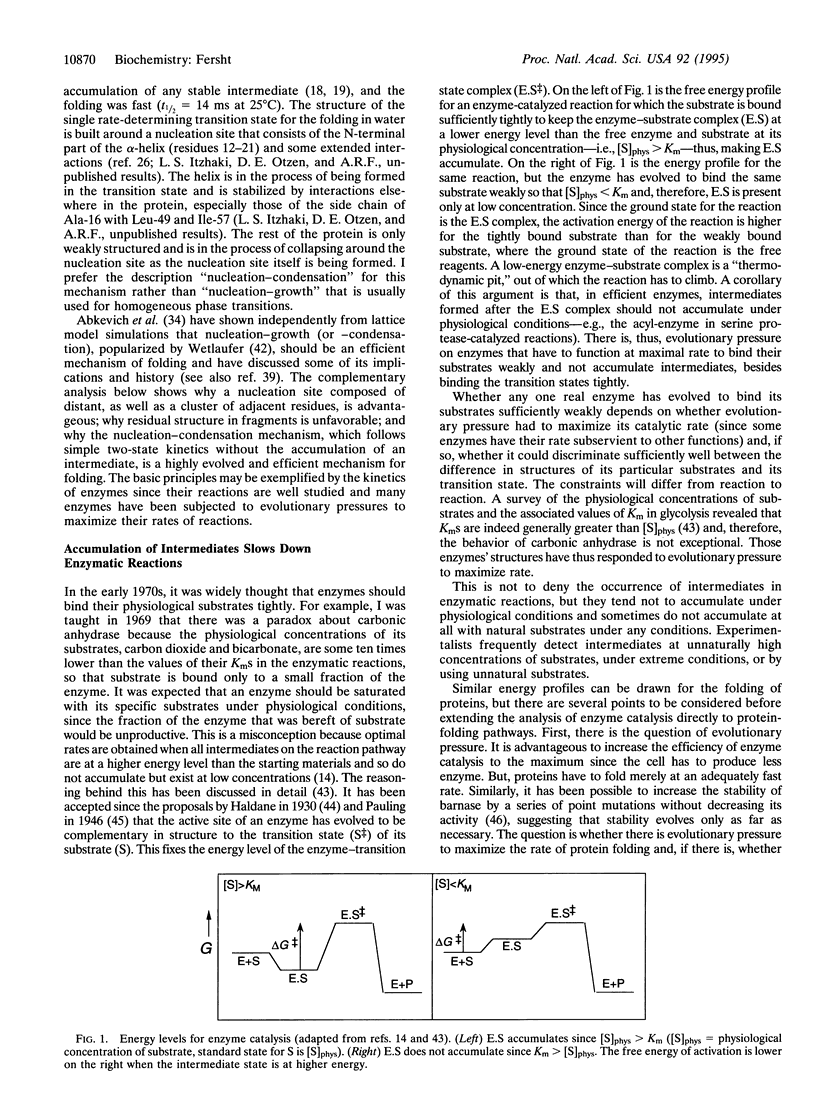
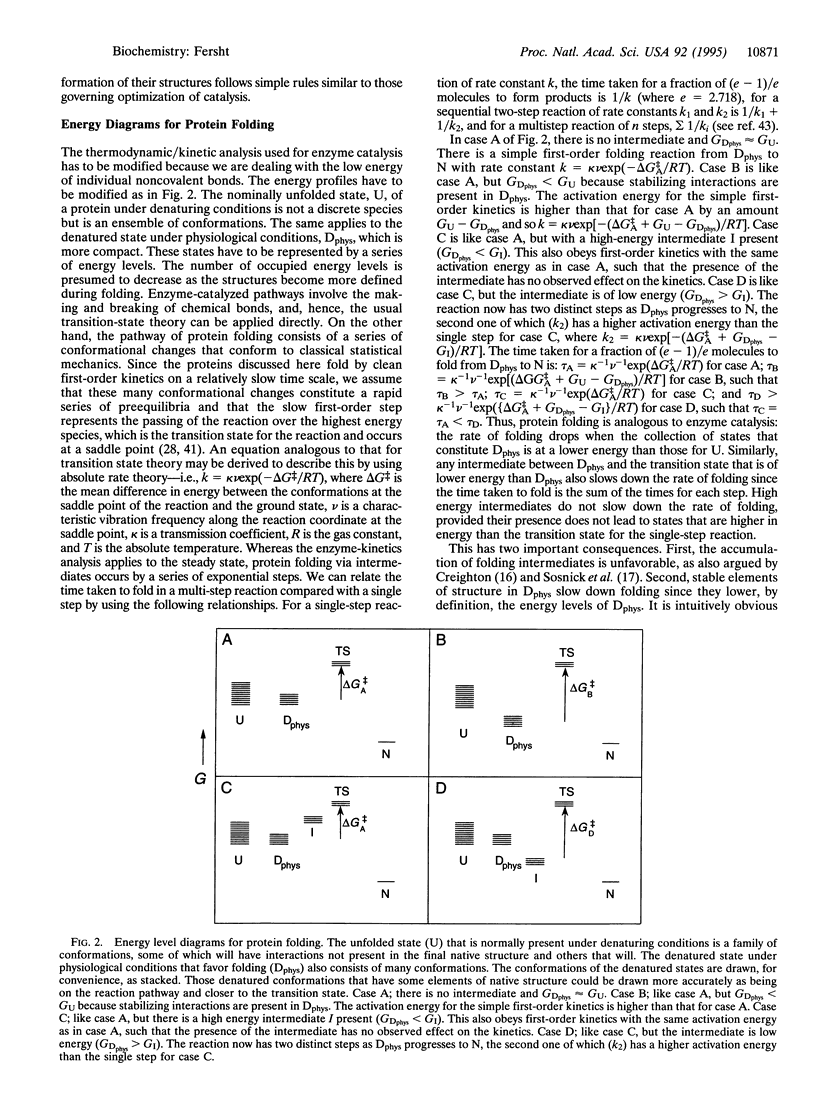
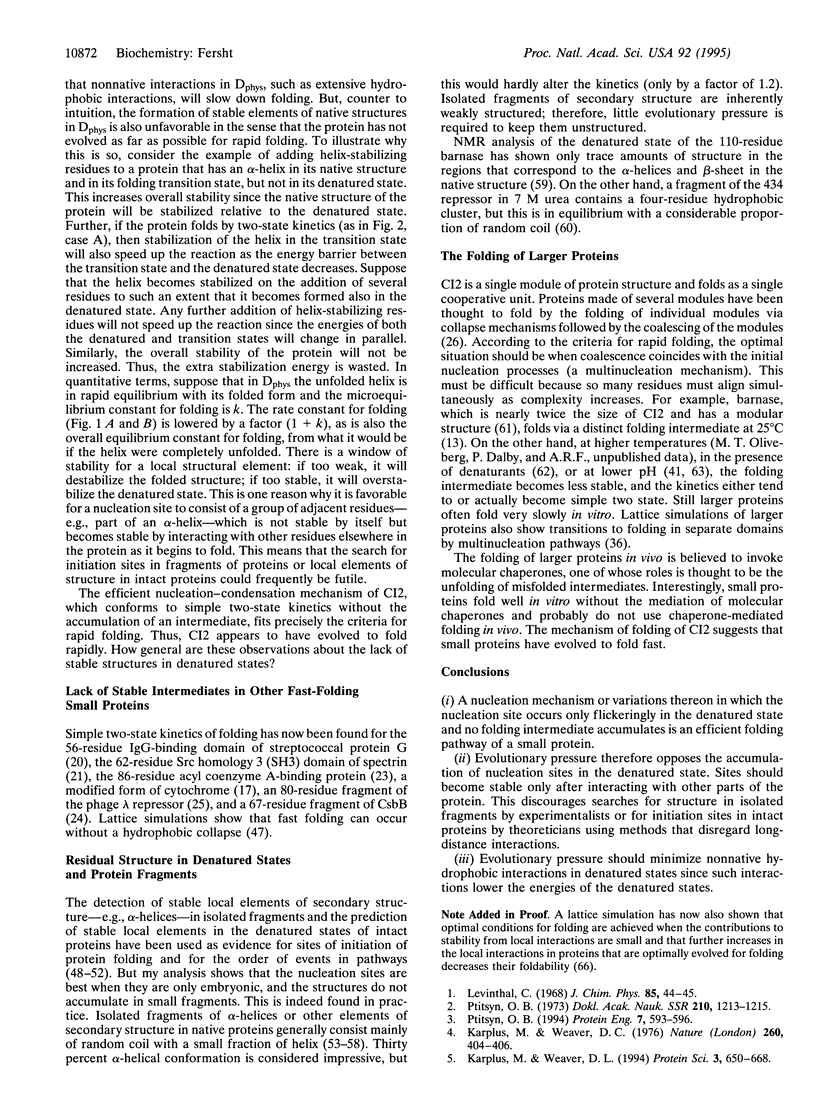
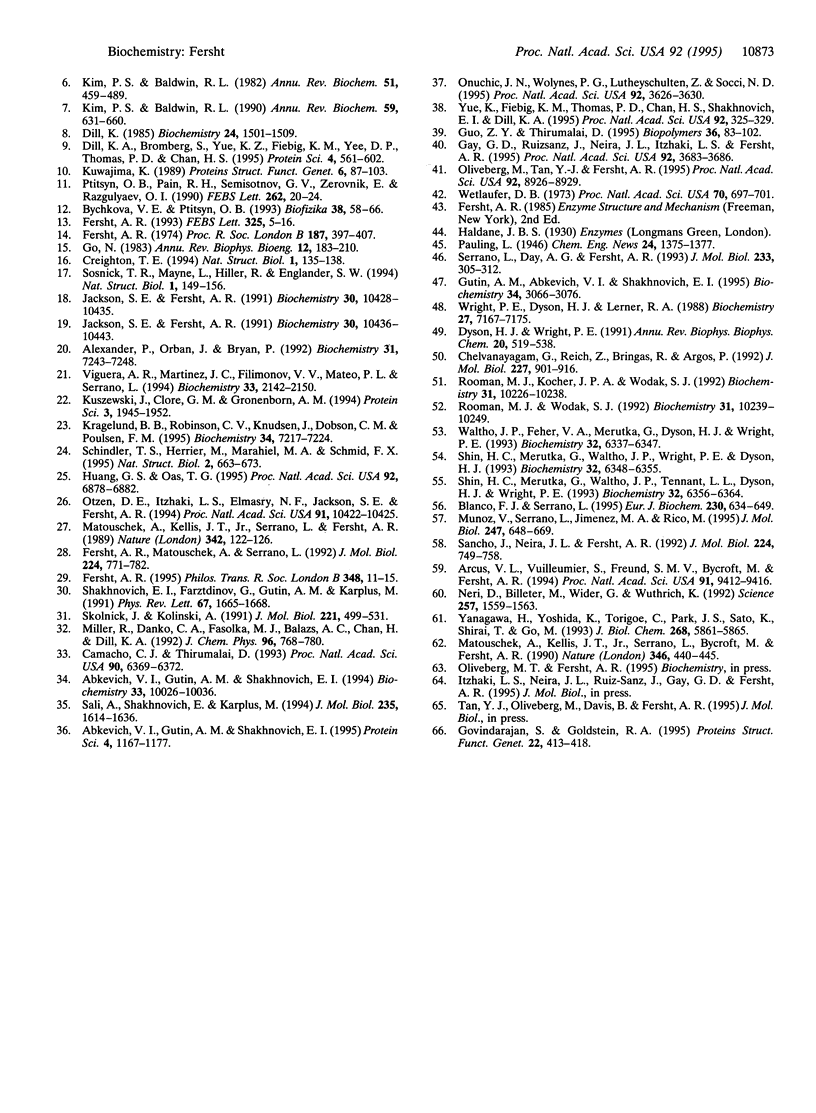
Selected References
These references are in PubMed. This may not be the complete list of references from this article.
- Abkevich V. I., Gutin A. M., Shakhnovich E. I. Domains in folding of model proteins. Protein Sci. 1995 Jun;4(6):1167–1177. doi: 10.1002/pro.5560040615. [DOI] [PMC free article] [PubMed] [Google Scholar]
- Abkevich V. I., Gutin A. M., Shakhnovich E. I. Specific nucleus as the transition state for protein folding: evidence from the lattice model. Biochemistry. 1994 Aug 23;33(33):10026–10036. doi: 10.1021/bi00199a029. [DOI] [PubMed] [Google Scholar]
- Alexander P., Orban J., Bryan P. Kinetic analysis of folding and unfolding the 56 amino acid IgG-binding domain of streptococcal protein G. Biochemistry. 1992 Aug 18;31(32):7243–7248. doi: 10.1021/bi00147a006. [DOI] [PubMed] [Google Scholar]
- Bychkova V. E., Ptitsyn O. B. Stostoianie rasplavlennoi globuly belkovykh molekul stanovitsia skoreepravilom, chem iskliucheniem. Biofizika. 1993 Jan-Feb;38(1):58–66. [PubMed] [Google Scholar]
- Camacho C. J., Thirumalai D. Kinetics and thermodynamics of folding in model proteins. Proc Natl Acad Sci U S A. 1993 Jul 1;90(13):6369–6372. doi: 10.1073/pnas.90.13.6369. [DOI] [PMC free article] [PubMed] [Google Scholar]
- Chelvanayagam G., Reich Z., Bringas R., Argos P. Prediction of protein folding pathways. J Mol Biol. 1992 Oct 5;227(3):901–916. doi: 10.1016/0022-2836(92)90230-h. [DOI] [PubMed] [Google Scholar]
- Creighton T. E. The energetic ups and downs of protein folding. Nat Struct Biol. 1994 Mar;1(3):135–138. doi: 10.1038/nsb0394-135. [DOI] [PubMed] [Google Scholar]
- De Prat Gay G., Ruiz-Sanz J., Neira J. L., Itzhaki L. S., Fersht A. R. Folding of a nascent polypeptide chain in vitro: cooperative formation of structure in a protein module. Proc Natl Acad Sci U S A. 1995 Apr 25;92(9):3683–3686. doi: 10.1073/pnas.92.9.3683. [DOI] [PMC free article] [PubMed] [Google Scholar]
- Dill K. A., Bromberg S., Yue K., Fiebig K. M., Yee D. P., Thomas P. D., Chan H. S. Principles of protein folding--a perspective from simple exact models. Protein Sci. 1995 Apr;4(4):561–602. doi: 10.1002/pro.5560040401. [DOI] [PMC free article] [PubMed] [Google Scholar]
- Dill K. A. Theory for the folding and stability of globular proteins. Biochemistry. 1985 Mar 12;24(6):1501–1509. doi: 10.1021/bi00327a032. [DOI] [PubMed] [Google Scholar]
- Dyson H. J., Wright P. E. Defining solution conformations of small linear peptides. Annu Rev Biophys Biophys Chem. 1991;20:519–538. doi: 10.1146/annurev.bb.20.060191.002511. [DOI] [PubMed] [Google Scholar]
- Fersht A. R. Catalysis, binding and enzyme-substrate complementarity. Proc R Soc Lond B Biol Sci. 1974 Nov 19;187(1089):397–407. doi: 10.1098/rspb.1974.0084. [DOI] [PubMed] [Google Scholar]
- Fersht A. R. Mapping the structures of transition states and intermediates in folding: delineation of pathways at high resolution. Philos Trans R Soc Lond B Biol Sci. 1995 Apr 29;348(1323):11–15. doi: 10.1098/rstb.1995.0040. [DOI] [PubMed] [Google Scholar]
- Fersht A. R., Matouschek A., Serrano L. The folding of an enzyme. I. Theory of protein engineering analysis of stability and pathway of protein folding. J Mol Biol. 1992 Apr 5;224(3):771–782. doi: 10.1016/0022-2836(92)90561-w. [DOI] [PubMed] [Google Scholar]
- Fersht A. R. The sixth Datta Lecture. Protein folding and stability: the pathway of folding of barnase. FEBS Lett. 1993 Jun 28;325(1-2):5–16. doi: 10.1016/0014-5793(93)81405-o. [DOI] [PubMed] [Google Scholar]
- Go N. Theoretical studies of protein folding. Annu Rev Biophys Bioeng. 1983;12:183–210. doi: 10.1146/annurev.bb.12.060183.001151. [DOI] [PubMed] [Google Scholar]
- Govindarajan S., Goldstein R. A. Optimal local propensities for model proteins. Proteins. 1995 Aug;22(4):413–418. doi: 10.1002/prot.340220411. [DOI] [PubMed] [Google Scholar]
- Gutin A. M., Abkevich V. I., Shakhnovich E. I. Is burst hydrophobic collapse necessary for protein folding? Biochemistry. 1995 Mar 7;34(9):3066–3076. doi: 10.1021/bi00009a038. [DOI] [PubMed] [Google Scholar]
- Huang G. S., Oas T. G. Submillisecond folding of monomeric lambda repressor. Proc Natl Acad Sci U S A. 1995 Jul 18;92(15):6878–6882. doi: 10.1073/pnas.92.15.6878. [DOI] [PMC free article] [PubMed] [Google Scholar]
- Jackson S. E., Fersht A. R. Folding of chymotrypsin inhibitor 2. 1. Evidence for a two-state transition. Biochemistry. 1991 Oct 29;30(43):10428–10435. doi: 10.1021/bi00107a010. [DOI] [PubMed] [Google Scholar]
- Jackson S. E., Fersht A. R. Folding of chymotrypsin inhibitor 2. 2. Influence of proline isomerization on the folding kinetics and thermodynamic characterization of the transition state of folding. Biochemistry. 1991 Oct 29;30(43):10436–10443. doi: 10.1021/bi00107a011. [DOI] [PubMed] [Google Scholar]
- Karplus M., Weaver D. L. Protein folding dynamics: the diffusion-collision model and experimental data. Protein Sci. 1994 Apr;3(4):650–668. doi: 10.1002/pro.5560030413. [DOI] [PMC free article] [PubMed] [Google Scholar]
- Karplus M., Weaver D. L. Protein-folding dynamics. Nature. 1976 Apr 1;260(5550):404–406. doi: 10.1038/260404a0. [DOI] [PubMed] [Google Scholar]
- Kim P. S., Baldwin R. L. Intermediates in the folding reactions of small proteins. Annu Rev Biochem. 1990;59:631–660. doi: 10.1146/annurev.bi.59.070190.003215. [DOI] [PubMed] [Google Scholar]
- Kim P. S., Baldwin R. L. Specific intermediates in the folding reactions of small proteins and the mechanism of protein folding. Annu Rev Biochem. 1982;51:459–489. doi: 10.1146/annurev.bi.51.070182.002331. [DOI] [PubMed] [Google Scholar]
- Kragelund B. B., Robinson C. V., Knudsen J., Dobson C. M., Poulsen F. M. Folding of a four-helix bundle: studies of acyl-coenzyme A binding protein. Biochemistry. 1995 May 30;34(21):7217–7224. doi: 10.1021/bi00021a037. [DOI] [PubMed] [Google Scholar]
- Kuszewski J., Clore G. M., Gronenborn A. M. Fast folding of a prototypic polypeptide: the immunoglobulin binding domain of streptococcal protein G. Protein Sci. 1994 Nov;3(11):1945–1952. doi: 10.1002/pro.5560031106. [DOI] [PMC free article] [PubMed] [Google Scholar]
- Kuwajima K. The molten globule state as a clue for understanding the folding and cooperativity of globular-protein structure. Proteins. 1989;6(2):87–103. doi: 10.1002/prot.340060202. [DOI] [PubMed] [Google Scholar]
- Matouschek A., Kellis J. T., Jr, Serrano L., Bycroft M., Fersht A. R. Transient folding intermediates characterized by protein engineering. Nature. 1990 Aug 2;346(6283):440–445. doi: 10.1038/346440a0. [DOI] [PubMed] [Google Scholar]
- Matouschek A., Kellis J. T., Jr, Serrano L., Fersht A. R. Mapping the transition state and pathway of protein folding by protein engineering. Nature. 1989 Jul 13;340(6229):122–126. doi: 10.1038/340122a0. [DOI] [PubMed] [Google Scholar]
- Muñoz V., Serrano L., Jiménez M. A., Rico M. Structural analysis of peptides encompassing all alpha-helices of three alpha/beta parallel proteins: Che-Y, flavodoxin and P21-ras: implications for alpha-helix stability and the folding of alpha/beta parallel proteins. J Mol Biol. 1995 Apr 7;247(4):648–669. doi: 10.1016/s0022-2836(05)80145-7. [DOI] [PubMed] [Google Scholar]
- Neri D., Billeter M., Wider G., Wüthrich K. NMR determination of residual structure in a urea-denatured protein, the 434-repressor. Science. 1992 Sep 11;257(5076):1559–1563. doi: 10.1126/science.1523410. [DOI] [PubMed] [Google Scholar]
- Oliveberg M., Tan Y. J., Fersht A. R. Negative activation enthalpies in the kinetics of protein folding. Proc Natl Acad Sci U S A. 1995 Sep 12;92(19):8926–8929. doi: 10.1073/pnas.92.19.8926. [DOI] [PMC free article] [PubMed] [Google Scholar]
- Onuchic J. N., Wolynes P. G., Luthey-Schulten Z., Socci N. D. Toward an outline of the topography of a realistic protein-folding funnel. Proc Natl Acad Sci U S A. 1995 Apr 11;92(8):3626–3630. doi: 10.1073/pnas.92.8.3626. [DOI] [PMC free article] [PubMed] [Google Scholar]
- Otzen D. E., Itzhaki L. S., elMasry N. F., Jackson S. E., Fersht A. R. Structure of the transition state for the folding/unfolding of the barley chymotrypsin inhibitor 2 and its implications for mechanisms of protein folding. Proc Natl Acad Sci U S A. 1994 Oct 25;91(22):10422–10425. doi: 10.1073/pnas.91.22.10422. [DOI] [PMC free article] [PubMed] [Google Scholar]
- Ptitsyn O. B. Kinetic and equilibrium intermediates in protein folding. Protein Eng. 1994 May;7(5):593–596. doi: 10.1093/protein/7.5.593. [DOI] [PubMed] [Google Scholar]
- Ptitsyn O. B., Pain R. H., Semisotnov G. V., Zerovnik E., Razgulyaev O. I. Evidence for a molten globule state as a general intermediate in protein folding. FEBS Lett. 1990 Mar 12;262(1):20–24. doi: 10.1016/0014-5793(90)80143-7. [DOI] [PubMed] [Google Scholar]
- Ptitsyn O. B. Stadiinyi mekhanizm samoorganizatsii belkovykh molekul. Dokl Akad Nauk SSSR. 1973 Jun 11;210(5):1213–1215. [PubMed] [Google Scholar]
- Rooman M. J., Kocher J. P., Wodak S. J. Extracting information on folding from the amino acid sequence: accurate predictions for protein regions with preferred conformation in the absence of tertiary interactions. Biochemistry. 1992 Oct 27;31(42):10226–10238. doi: 10.1021/bi00157a009. [DOI] [PubMed] [Google Scholar]
- Rooman M. J., Wodak S. J. Extracting information on folding from the amino acid sequence: consensus regions with preferred conformation in homologous proteins. Biochemistry. 1992 Oct 27;31(42):10239–10249. doi: 10.1021/bi00157a010. [DOI] [PubMed] [Google Scholar]
- Sali A., Shakhnovich E., Karplus M. Kinetics of protein folding. A lattice model study of the requirements for folding to the native state. J Mol Biol. 1994 Feb 4;235(5):1614–1636. doi: 10.1006/jmbi.1994.1110. [DOI] [PubMed] [Google Scholar]
- Sancho J., Neira J. L., Fersht A. R. An N-terminal fragment of barnase has residual helical structure similar to that in a refolding intermediate. J Mol Biol. 1992 Apr 5;224(3):749–758. doi: 10.1016/0022-2836(92)90559-3. [DOI] [PubMed] [Google Scholar]
- Schindler T., Herrler M., Marahiel M. A., Schmid F. X. Extremely rapid protein folding in the absence of intermediates. Nat Struct Biol. 1995 Aug;2(8):663–673. doi: 10.1038/nsb0895-663. [DOI] [PubMed] [Google Scholar]
- Serrano L., Day A. G., Fersht A. R. Step-wise mutation of barnase to binase. A procedure for engineering increased stability of proteins and an experimental analysis of the evolution of protein stability. J Mol Biol. 1993 Sep 20;233(2):305–312. doi: 10.1006/jmbi.1993.1508. [DOI] [PubMed] [Google Scholar]
- Shakhnovich E, Farztdinov G, Gutin AM, Karplus M. Protein folding bottlenecks: A lattice Monte Carlo simulation. Phys Rev Lett. 1991 Sep 16;67(12):1665–1668. doi: 10.1103/PhysRevLett.67.1665. [DOI] [PubMed] [Google Scholar]
- Shin H. C., Merutka G., Waltho J. P., Wright P. E., Dyson H. J. Peptide models of protein folding initiation sites. 2. The G-H turn region of myoglobin acts as a helix stop signal. Biochemistry. 1993 Jun 29;32(25):6348–6355. doi: 10.1021/bi00076a007. [DOI] [PubMed] [Google Scholar]
- Skolnick J., Kolinski A. Dynamic Monte Carlo simulations of a new lattice model of globular protein folding, structure and dynamics. J Mol Biol. 1991 Sep 20;221(2):499–531. doi: 10.1016/0022-2836(91)80070-b. [DOI] [PubMed] [Google Scholar]
- Sosnick T. R., Mayne L., Hiller R., Englander S. W. The barriers in protein folding. Nat Struct Biol. 1994 Mar;1(3):149–156. doi: 10.1038/nsb0394-149. [DOI] [PubMed] [Google Scholar]
- Viguera A. R., Martínez J. C., Filimonov V. V., Mateo P. L., Serrano L. Thermodynamic and kinetic analysis of the SH3 domain of spectrin shows a two-state folding transition. Biochemistry. 1994 Mar 1;33(8):2142–2150. doi: 10.1021/bi00174a022. [DOI] [PubMed] [Google Scholar]
- Waltho J. P., Feher V. A., Merutka G., Dyson H. J., Wright P. E. Peptide models of protein folding initiation sites. 1. Secondary structure formation by peptides corresponding to the G- and H-helices of myoglobin. Biochemistry. 1993 Jun 29;32(25):6337–6347. doi: 10.1021/bi00076a006. [DOI] [PubMed] [Google Scholar]
- Wetlaufer D. B. Nucleation, rapid folding, and globular intrachain regions in proteins. Proc Natl Acad Sci U S A. 1973 Mar;70(3):697–701. doi: 10.1073/pnas.70.3.697. [DOI] [PMC free article] [PubMed] [Google Scholar]
- Wright P. E., Dyson H. J., Lerner R. A. Conformation of peptide fragments of proteins in aqueous solution: implications for initiation of protein folding. Biochemistry. 1988 Sep 20;27(19):7167–7175. doi: 10.1021/bi00419a001. [DOI] [PubMed] [Google Scholar]
- Yanagawa H., Yoshida K., Torigoe C., Park J. S., Sato K., Shirai T., Go M. Protein anatomy: functional roles of barnase module. J Biol Chem. 1993 Mar 15;268(8):5861–5865. [PubMed] [Google Scholar]
- Yue K., Fiebig K. M., Thomas P. D., Chan H. S., Shakhnovich E. I., Dill K. A. A test of lattice protein folding algorithms. Proc Natl Acad Sci U S A. 1995 Jan 3;92(1):325–329. doi: 10.1073/pnas.92.1.325. [DOI] [PMC free article] [PubMed] [Google Scholar]