Abstract
Synapsin I has been proposed to be involved in the modulation of neurotransmitter release by controlling the availability of synaptic vesicles for exocytosis. To further understand the role of synapsin I in the function of adult nerve terminals, we studied synapsin I-deficient mice generated by homologous recombination. The organization of synaptic vesicles at presynaptic terminals of synapsin I-deficient mice was markedly altered: densely packed vesicles were only present in a narrow rim at active zones, whereas the majority of vesicles were dispersed throughout the terminal area. This was in contrast to the organized vesicle clusters present in terminals of wild-type animals. Release of glutamate from nerve endings, induced by K+,4-aminopyridine, or a Ca2+ ionophore, was markedly decreased in synapsin I mutant mice. The recovery of synaptic transmission after depletion of neurotransmitter by high-frequency stimulation was greatly delayed. Finally, synapsin I-deficient mice exhibited a strikingly increased response to electrical stimulation, as measured by electrographic and behavioral seizures. These results provide strong support for the hypothesis that synapsin I plays a key role in the regulation of nerve terminal function in mature synapses.
Full text
PDF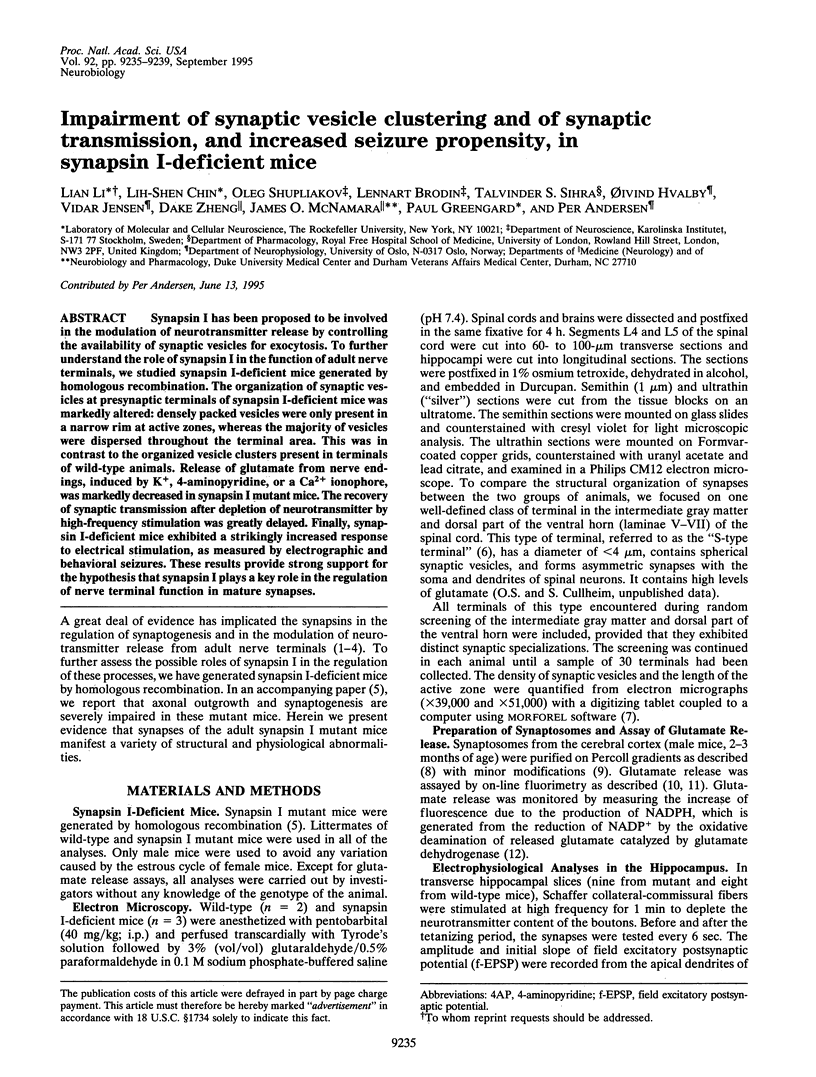
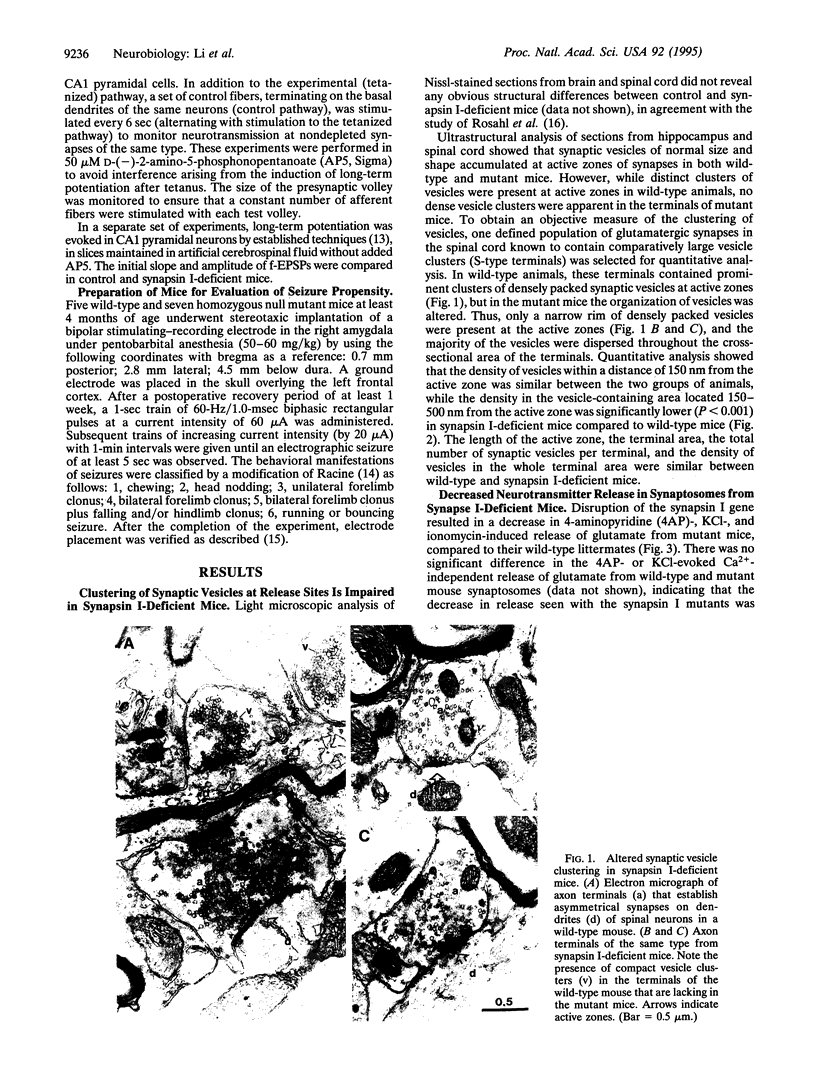
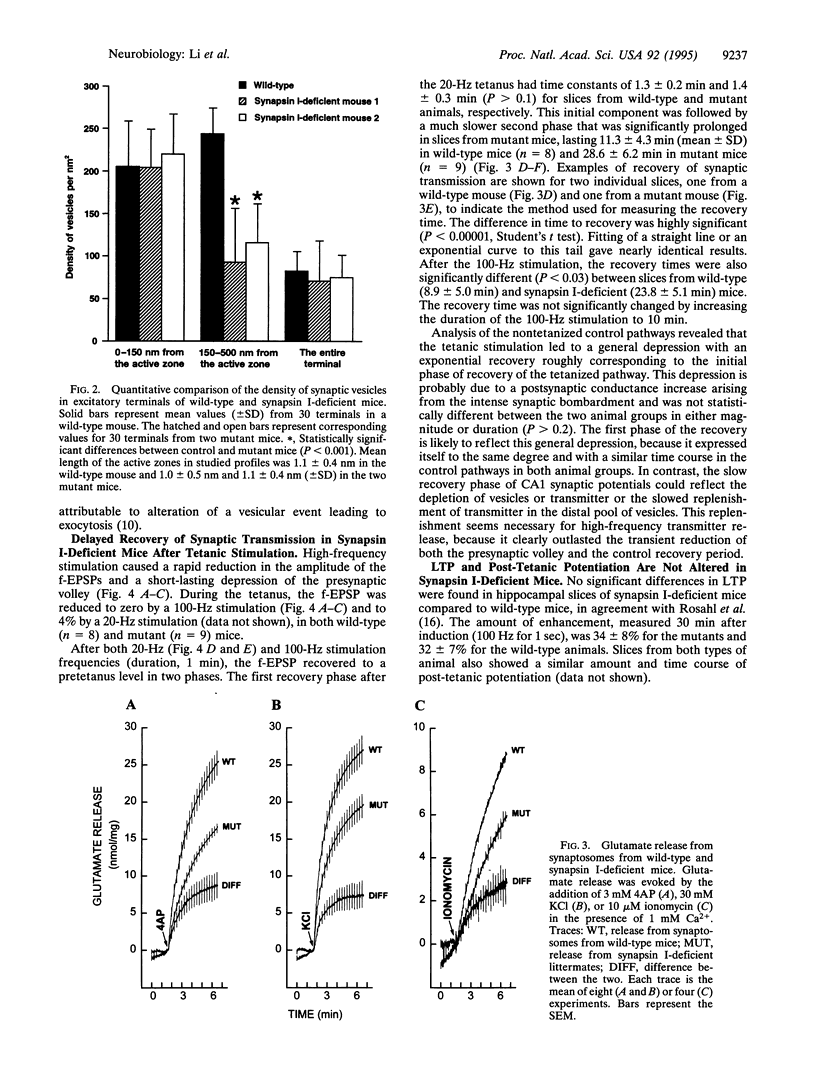
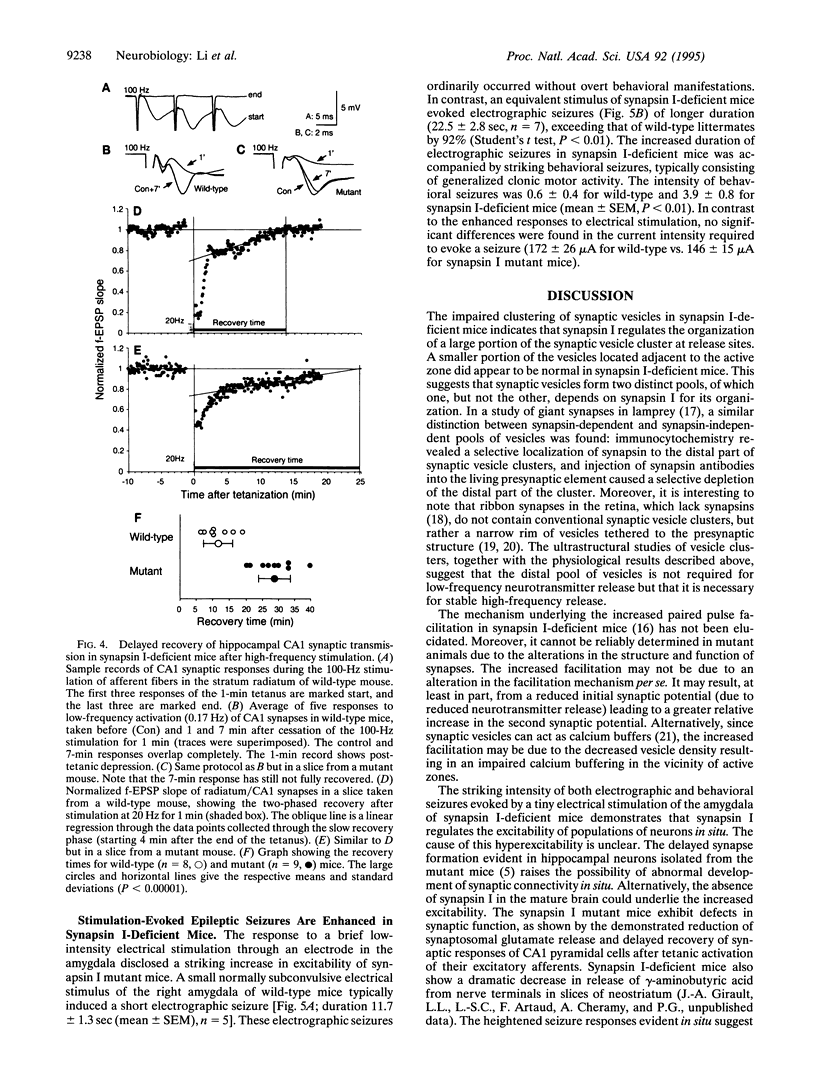
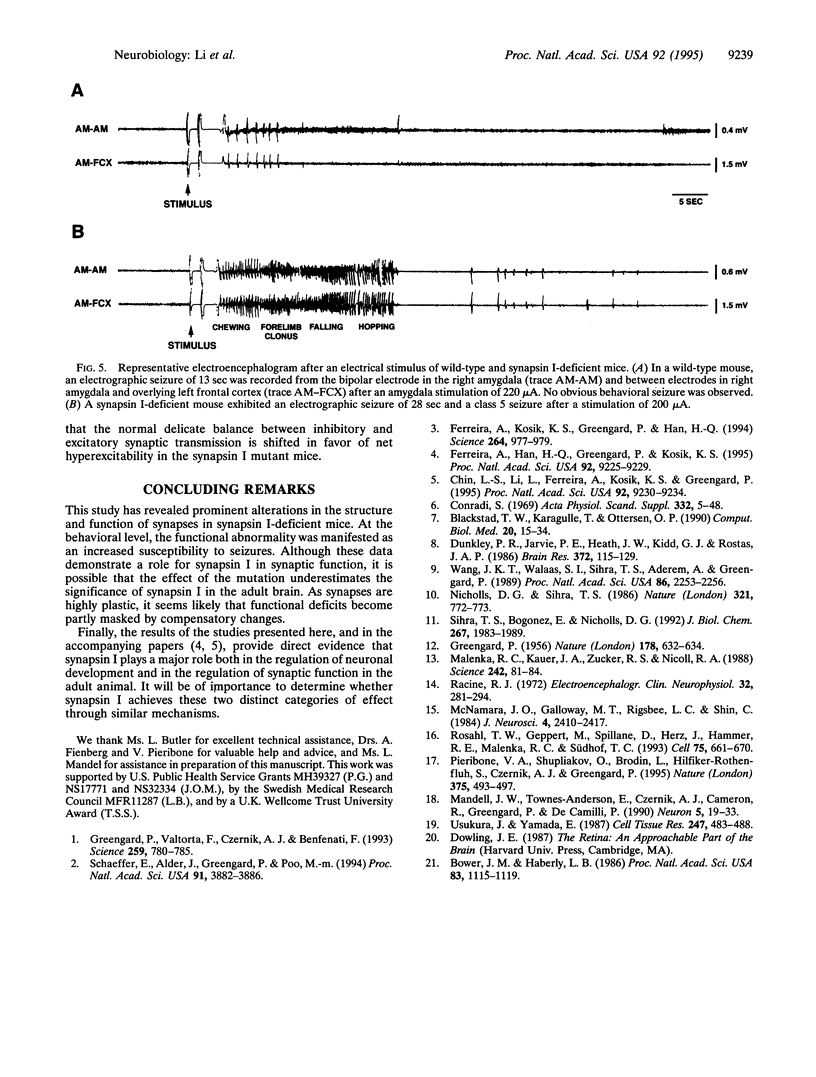
Images in this article
Selected References
These references are in PubMed. This may not be the complete list of references from this article.
- Blackstad T. W., Karagülle T., Ottersen O. P. MORFOREL, a computer program for two-dimensional analysis of micrographs of biological specimens, with emphasis on immunogold preparations. Comput Biol Med. 1990;20(1):15–34. doi: 10.1016/0010-4825(90)90041-m. [DOI] [PubMed] [Google Scholar]
- Bower J. M., Haberly L. B. Facilitating and nonfacilitating synapses on pyramidal cells: a correlation between physiology and morphology. Proc Natl Acad Sci U S A. 1986 Feb;83(4):1115–1119. doi: 10.1073/pnas.83.4.1115. [DOI] [PMC free article] [PubMed] [Google Scholar]
- Chin L. S., Li L., Ferreira A., Kosik K. S., Greengard P. Impairment of axonal development and of synaptogenesis in hippocampal neurons of synapsin I-deficient mice. Proc Natl Acad Sci U S A. 1995 Sep 26;92(20):9230–9234. doi: 10.1073/pnas.92.20.9230. [DOI] [PMC free article] [PubMed] [Google Scholar]
- Conradi S. Ultrastructure and distribution of neuronal and glial elements on the motoneuron surface in the lumbosacral spinal cord of the adult cat. Acta Physiol Scand Suppl. 1969;332:5–48. [PubMed] [Google Scholar]
- Dunkley P. R., Jarvie P. E., Heath J. W., Kidd G. J., Rostas J. A. A rapid method for isolation of synaptosomes on Percoll gradients. Brain Res. 1986 Apr 30;372(1):115–129. doi: 10.1016/0006-8993(86)91464-2. [DOI] [PubMed] [Google Scholar]
- Ferreira A., Han H. Q., Greengard P., Kosik K. S. Suppression of synapsin II inhibits the formation and maintenance of synapses in hippocampal culture. Proc Natl Acad Sci U S A. 1995 Sep 26;92(20):9225–9229. doi: 10.1073/pnas.92.20.9225. [DOI] [PMC free article] [PubMed] [Google Scholar]
- Ferreira A., Kosik K. S., Greengard P., Han H. Q. Aberrant neurites and synaptic vesicle protein deficiency in synapsin II-depleted neurons. Science. 1994 May 13;264(5161):977–979. doi: 10.1126/science.8178158. [DOI] [PubMed] [Google Scholar]
- GREENGARD P. Determination of intermediary metabolites by enzymic fluorimetry. Nature. 1956 Sep 22;178(4534):632–634. doi: 10.1038/178632a0. [DOI] [PubMed] [Google Scholar]
- Greengard P., Valtorta F., Czernik A. J., Benfenati F. Synaptic vesicle phosphoproteins and regulation of synaptic function. Science. 1993 Feb 5;259(5096):780–785. doi: 10.1126/science.8430330. [DOI] [PubMed] [Google Scholar]
- Malenka R. C., Kauer J. A., Zucker R. S., Nicoll R. A. Postsynaptic calcium is sufficient for potentiation of hippocampal synaptic transmission. Science. 1988 Oct 7;242(4875):81–84. doi: 10.1126/science.2845577. [DOI] [PubMed] [Google Scholar]
- Mandell J. W., Townes-Anderson E., Czernik A. J., Cameron R., Greengard P., De Camilli P. Synapsins in the vertebrate retina: absence from ribbon synapses and heterogeneous distribution among conventional synapses. Neuron. 1990 Jul;5(1):19–33. doi: 10.1016/0896-6273(90)90030-j. [DOI] [PubMed] [Google Scholar]
- McNamara J. O., Galloway M. T., Rigsbee L. C., Shin C. Evidence implicating substantia nigra in regulation of kindled seizure threshold. J Neurosci. 1984 Sep;4(9):2410–2417. doi: 10.1523/JNEUROSCI.04-09-02410.1984. [DOI] [PMC free article] [PubMed] [Google Scholar]
- Nicholls D. G., Sihra T. S. Synaptosomes possess an exocytotic pool of glutamate. Nature. 1986 Jun 19;321(6072):772–773. doi: 10.1038/321772a0. [DOI] [PubMed] [Google Scholar]
- Pieribone V. A., Shupliakov O., Brodin L., Hilfiker-Rothenfluh S., Czernik A. J., Greengard P. Distinct pools of synaptic vesicles in neurotransmitter release. Nature. 1995 Jun 8;375(6531):493–497. doi: 10.1038/375493a0. [DOI] [PubMed] [Google Scholar]
- Racine R. J. Modification of seizure activity by electrical stimulation. II. Motor seizure. Electroencephalogr Clin Neurophysiol. 1972 Mar;32(3):281–294. doi: 10.1016/0013-4694(72)90177-0. [DOI] [PubMed] [Google Scholar]
- Rosahl T. W., Geppert M., Spillane D., Herz J., Hammer R. E., Malenka R. C., Südhof T. C. Short-term synaptic plasticity is altered in mice lacking synapsin I. Cell. 1993 Nov 19;75(4):661–670. doi: 10.1016/0092-8674(93)90487-b. [DOI] [PubMed] [Google Scholar]
- Schaeffer E., Alder J., Greengard P., Poo M. M. Synapsin IIa accelerates functional development of neuromuscular synapses. Proc Natl Acad Sci U S A. 1994 Apr 26;91(9):3882–3886. doi: 10.1073/pnas.91.9.3882. [DOI] [PMC free article] [PubMed] [Google Scholar]
- Sihra T. S., Bogonez E., Nicholls D. G. Localized Ca2+ entry preferentially effects protein dephosphorylation, phosphorylation, and glutamate release. J Biol Chem. 1992 Jan 25;267(3):1983–1989. [PubMed] [Google Scholar]
- Usukura J., Yamada E. Ultrastructure of the synaptic ribbons in photoreceptor cells of Rana catesbeiana revealed by freeze-etching and freeze-substitution. Cell Tissue Res. 1987 Mar;247(3):483–488. doi: 10.1007/BF00215740. [DOI] [PubMed] [Google Scholar]
- Wang J. K., Walaas S. I., Sihra T. S., Aderem A., Greengard P. Phosphorylation and associated translocation of the 87-kDa protein, a major protein kinase C substrate, in isolated nerve terminals. Proc Natl Acad Sci U S A. 1989 Apr;86(7):2253–2256. doi: 10.1073/pnas.86.7.2253. [DOI] [PMC free article] [PubMed] [Google Scholar]