Abstract
In earlier studies it was shown that the mammalian translation system is highly organized in vivo and that the intermediates in the process, aminoacyl-tRNAs, are channeled--i.e., they are directly transferred from the aminoacyl-tRNA synthetases to the elongation factor to the ribosomes without dissociating into the cellular fluid. Here, we examine whether spent tRNAs leaving the ribosome enter the fluid phase or are transferred directly to their cognate aminoacyl-tRNA synthetases to complete a channeled tRNA cycle. Using a permeabilized CHO cell system that closely mimics living cells, we find that there is no leakage of endogenous tRNA during many cycles of translation, and protein synthesis remains linear during this period, even though free aminoacyl-tRNA is known to rapidly equilibrate between the inside and outside of these cells. We also find that exogenous tRNA and periodate-oxidized tRNA have no effect on protein synthesis in this system, indicating that they do not enter the translation machinery, despite the fact that exogenous tRNA rapidly distributes throughout the cells. Furthermore, most of the cellular aminoacyl-tRNA synthetases function only with endogenous tRNAs, although a portion can use exogenous tRNA molecules. However, aminoacylation of these exogenous tRNAs is strongly inhibited by oxidized tRNA; this inhibitor has no effect on endogenous aminoacylation. On the basis of these and the earlier observations, we conclude that endogenous tRNA is never free of the protein synthetic machinery at any stage of the translation process and, consequently, that there is a channeled tRNA cycle during protein synthesis in mammalian cells.
Full text
PDF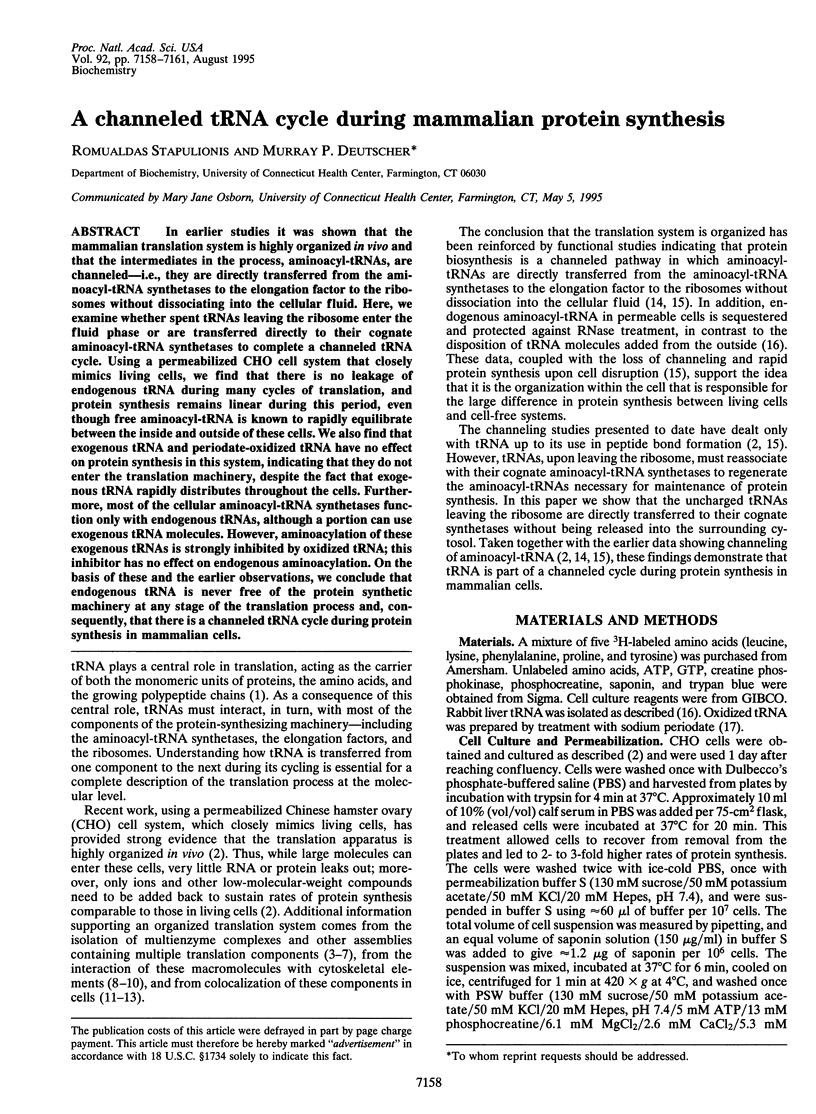
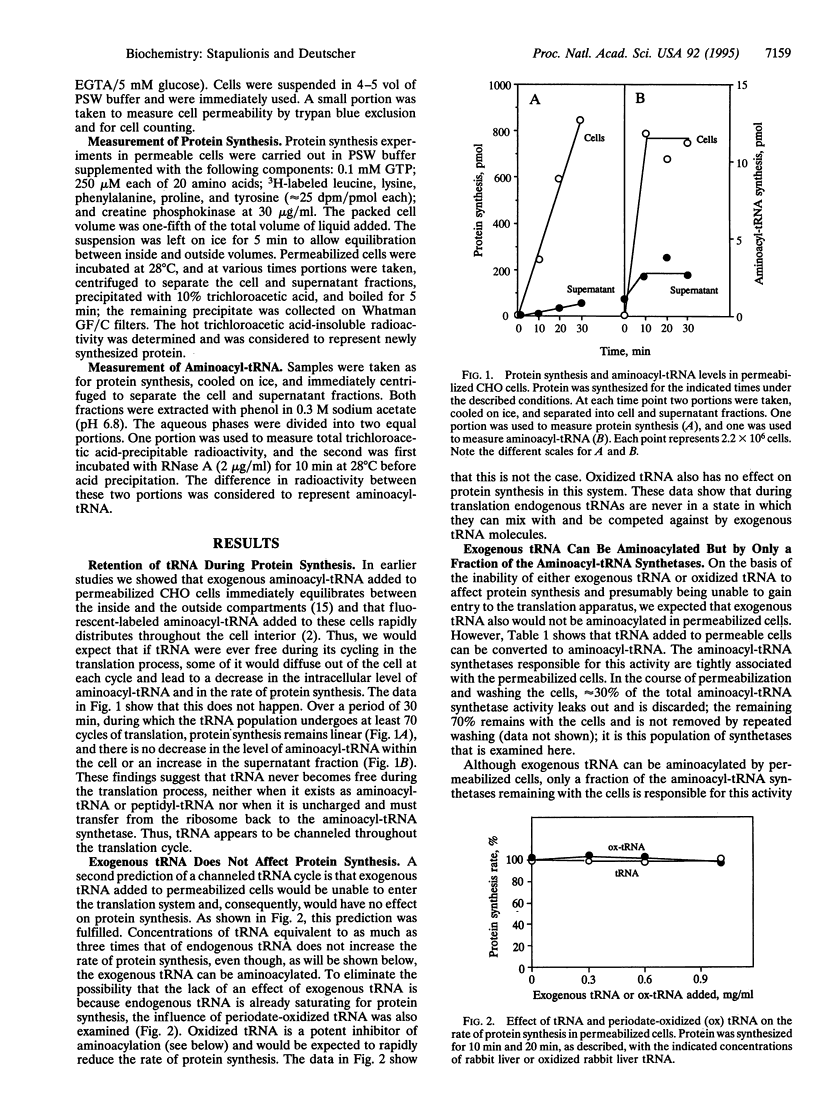
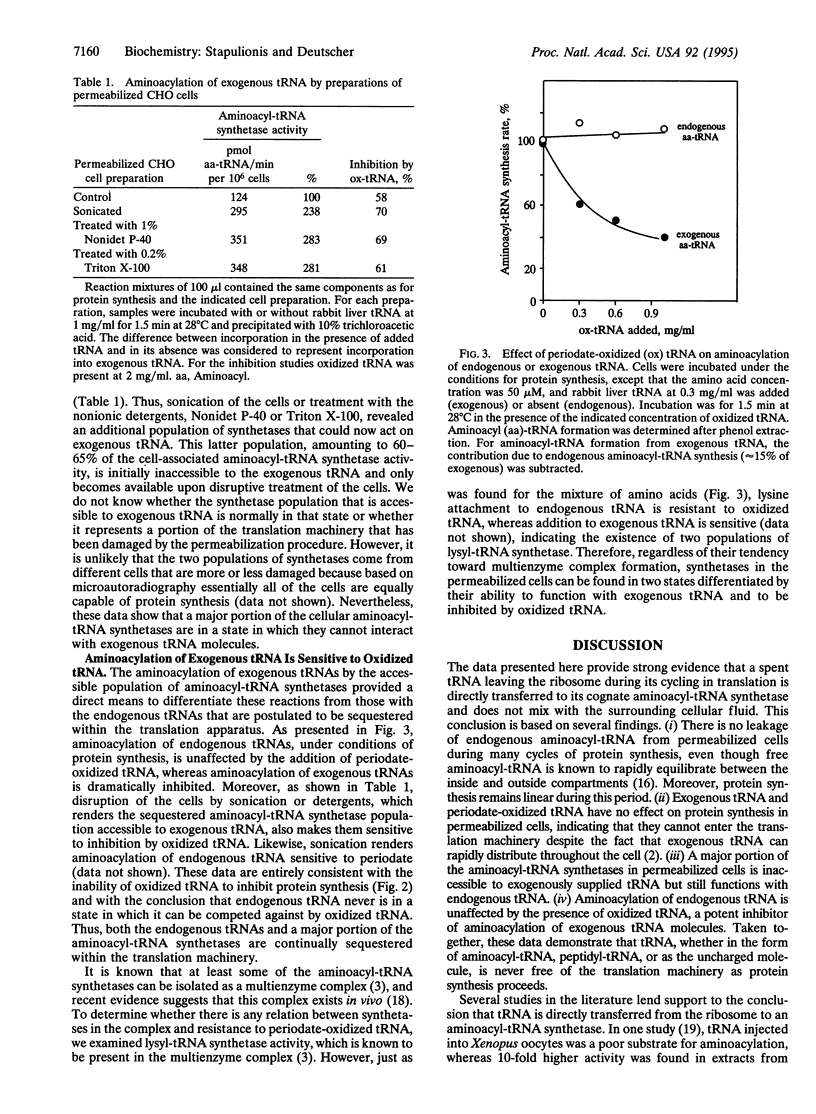
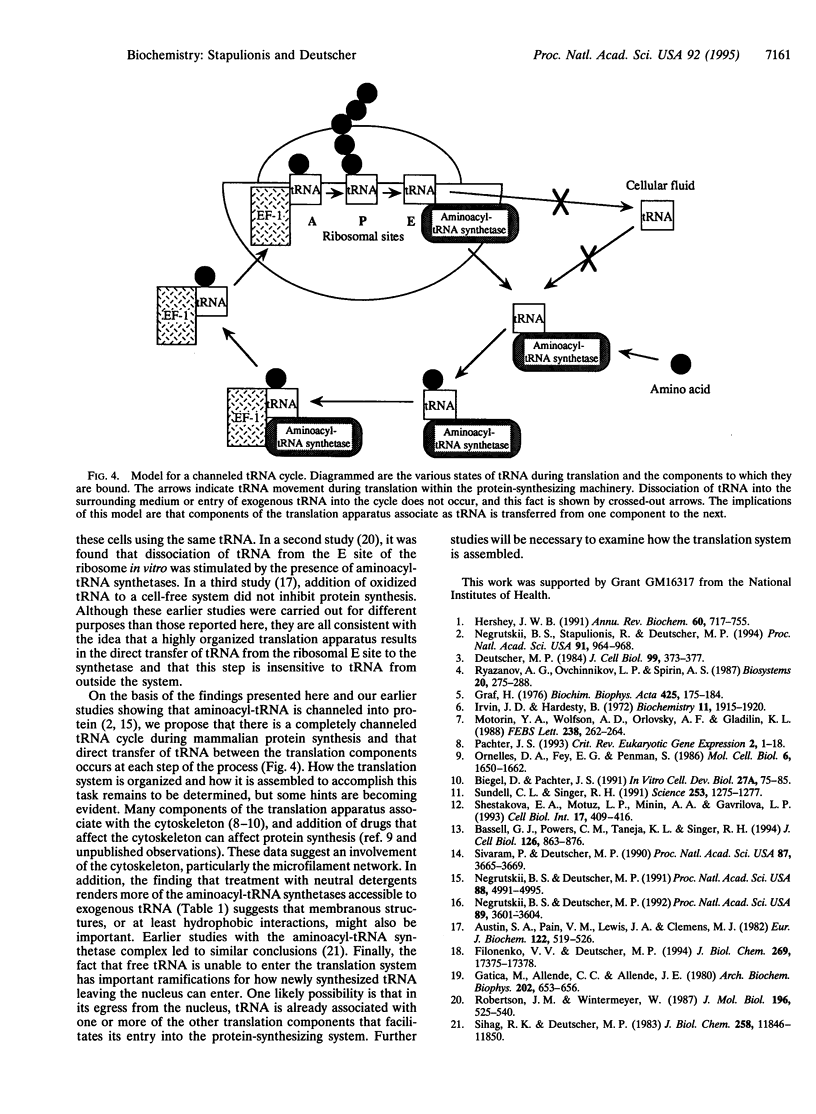
Selected References
These references are in PubMed. This may not be the complete list of references from this article.
- Austin S. A., Pain V. M., Lewis J. A., Clemens M. J. Investigation of the role of uncharged tRNA in the regulation of polypeptide chain initiation by amino acid starvation in cultured mammalian cells; a reappraisal. Eur J Biochem. 1982 Mar 1;122(3):519–526. doi: 10.1111/j.1432-1033.1982.tb06468.x. [DOI] [PubMed] [Google Scholar]
- Bassell G. J., Powers C. M., Taneja K. L., Singer R. H. Single mRNAs visualized by ultrastructural in situ hybridization are principally localized at actin filament intersections in fibroblasts. J Cell Biol. 1994 Aug;126(4):863–876. doi: 10.1083/jcb.126.4.863. [DOI] [PMC free article] [PubMed] [Google Scholar]
- Biegel D., Pachter J. S. "In situ" translation: use of the cytoskeletal framework to direct cell-free protein synthesis. In Vitro Cell Dev Biol. 1991 Jan;27(1):75–85. doi: 10.1007/BF02630897. [DOI] [PubMed] [Google Scholar]
- Deutscher M. P. The eucaryotic aminoacyl-tRNA synthetase complex: suggestions for its structure and function. J Cell Biol. 1984 Aug;99(2):373–377. doi: 10.1083/jcb.99.2.373. [DOI] [PMC free article] [PubMed] [Google Scholar]
- Filonenko V. V., Deutscher M. P. Evidence for similar structural organization of the multienzyme aminoacyl-tRNA synthetase complex in vivo and in vitro. J Biol Chem. 1994 Jul 1;269(26):17375–17378. [PubMed] [Google Scholar]
- Gatica M., Allende C. C., Allende J. E. Evidence for in vivo compartmentation of phenylalanyl-tRNA ligase in amphibian oocytes. Arch Biochem Biophys. 1980 Jul;202(2):653–656. doi: 10.1016/0003-9861(80)90474-9. [DOI] [PubMed] [Google Scholar]
- Graf H. Intraction of aminoacyl-tRNA synthetases with ribosomes and ribosomal subunits. Biochim Biophys Acta. 1976 Mar 4;425(2):175–184. doi: 10.1016/0005-2787(76)90023-x. [DOI] [PubMed] [Google Scholar]
- Hershey J. W. Translational control in mammalian cells. Annu Rev Biochem. 1991;60:717–755. doi: 10.1146/annurev.bi.60.070191.003441. [DOI] [PubMed] [Google Scholar]
- Irvin J. D., Hardesty B. Binding of aminoacyl transfer ribonucleic acid synthetases to ribosomes from rabbit reticulocytes. Biochemistry. 1972 May 9;11(10):1915–1920. doi: 10.1021/bi00760a028. [DOI] [PubMed] [Google Scholar]
- Motorin YuA, Wolfson A. D., Orlovsky A. F., Gladilin K. L. Mammalian valyl-tRNA synthetase forms a complex with the first elongation factor. FEBS Lett. 1988 Oct 10;238(2):262–264. doi: 10.1016/0014-5793(88)80492-7. [DOI] [PubMed] [Google Scholar]
- Negrutskii B. S., Deutscher M. P. A sequestered pool of aminoacyl-tRNA in mammalian cells. Proc Natl Acad Sci U S A. 1992 Apr 15;89(8):3601–3604. doi: 10.1073/pnas.89.8.3601. [DOI] [PMC free article] [PubMed] [Google Scholar]
- Negrutskii B. S., Deutscher M. P. Channeling of aminoacyl-tRNA for protein synthesis in vivo. Proc Natl Acad Sci U S A. 1991 Jun 1;88(11):4991–4995. doi: 10.1073/pnas.88.11.4991. [DOI] [PMC free article] [PubMed] [Google Scholar]
- Negrutskii B. S., Stapulionis R., Deutscher M. P. Supramolecular organization of the mammalian translation system. Proc Natl Acad Sci U S A. 1994 Feb 1;91(3):964–968. doi: 10.1073/pnas.91.3.964. [DOI] [PMC free article] [PubMed] [Google Scholar]
- Ornelles D. A., Fey E. G., Penman S. Cytochalasin releases mRNA from the cytoskeletal framework and inhibits protein synthesis. Mol Cell Biol. 1986 May;6(5):1650–1662. doi: 10.1128/mcb.6.5.1650. [DOI] [PMC free article] [PubMed] [Google Scholar]
- Pachter J. S. Association of mRNA with the cytoskeletal framework: its role in the regulation of gene expression. Crit Rev Eukaryot Gene Expr. 1992;2(1):1–18. [PubMed] [Google Scholar]
- Robertson J. M., Wintermeyer W. Mechanism of ribosomal translocation. tRNA binds transiently to an exit site before leaving the ribosome during translocation. J Mol Biol. 1987 Aug 5;196(3):525–540. doi: 10.1016/0022-2836(87)90030-1. [DOI] [PubMed] [Google Scholar]
- Ryazanov A. G., Ovchinnikov L. P., Spirin A. S. Development of structural organization of protein-synthesizing machinery from prokaryotes to eukaryotes. Biosystems. 1987;20(3):275–288. doi: 10.1016/0303-2647(87)90035-9. [DOI] [PubMed] [Google Scholar]
- Shestakova E. A., Motuz L. P., Minin A. A., Gavrilova L. P. Study of localization of the protein-synthesizing machinery along actin filament bundles. Cell Biol Int. 1993 Apr;17(4):409–416. doi: 10.1006/cbir.1993.1079. [DOI] [PubMed] [Google Scholar]
- Sihag R. K., Deutscher M. P. Perturbation of the aminoacyl-tRNA synthetase complex by salts and detergents. Importance of hydrophobic interactions and possible involvement of lipids. J Biol Chem. 1983 Oct 10;258(19):11846–11850. [PubMed] [Google Scholar]
- Sivaram P., Deutscher M. P. Existence of two forms of rat liver arginyl-tRNA synthetase suggests channeling of aminoacyl-tRNA for protein synthesis. Proc Natl Acad Sci U S A. 1990 May;87(10):3665–3669. doi: 10.1073/pnas.87.10.3665. [DOI] [PMC free article] [PubMed] [Google Scholar]
- Sundell C. L., Singer R. H. Requirement of microfilaments in sorting of actin messenger RNA. Science. 1991 Sep 13;253(5025):1275–1277. doi: 10.1126/science.1891715. [DOI] [PubMed] [Google Scholar]