Abstract
Squalene synthetase (farnesyltransferase; farnesyl diphosphate:farnesyl-diphosphate farnesyltransferase, EC 2.5.1.21), the enzyme in the cholesterol biosynthetic pathway that converts farnesyl pyrophosphate into squalene, is subject to regulation in cultured human fibroblasts. When cholesterol-carrying low density lipoprotein (LDL) was removed from the serum of the culture medium, squalene synthetase activity increased 8-fold over 24 hr. When LDL was added back to the medium, squalene synthetase was slowly suppressed, 50% and 90% reduction occurring in 15 and 48 hr, respectively. Suppression of squalene synthetase required uptake of LDL via the LDL receptor; hence, it did not occur in mutant fibroblasts from a patient with homozygous familial hypercholesterolemia that lack receptors. The addition of a mixture of 25-hydroxycholesterol and cholesterol suppressed squalene synthetase equally well in normal and mutant fibroblasts. Coupled with previous data, the current findings indicate that cholesterol derived from LDL regulates at least two enzymes in the cholesterol synthetic pathway in fibroblasts: (i) its primary action is to rapidly suppress 3-hydroxy-3-methylglutaryl coenzyme A reductase [mevalonate:NADP+, oxidoreductase (CoA-acylating), EC 1.1.1.34], which reduces mevalonate production by 95% within 8 hr, and (ii) its secondary action is to slowly suppress squalene synthetase. The LDL-mediated suppression of squalene synthetase does not regulate de novo cholesterol synthesis; it occurs after 3-hydroxy-3-methylglutaryl coenzyme A reductase is already suppressed. Rather, we hypothesize that it may function to allow the pool size of farnesyl pyrophosphate to be maintained in the presence of LDL so that low levels of mevalonate can be shunted preferentially into nonsterol products, such as ubiquinone-10 and dolichol. This mechanism may explain the earlier observation that the synthesis of ubiquinone-10 in fibroblasts proceeds at a normal rate in the presence of LDL despite a 95% decrease in mevalonate production.
Keywords: cholesterol biosynthesis, 3-hydroxy-3-methylglutaryl coenzyme A reductase, farnesyl pyrophosphate, cell surface receptors, familial hypercholesterolemia
Full text
PDF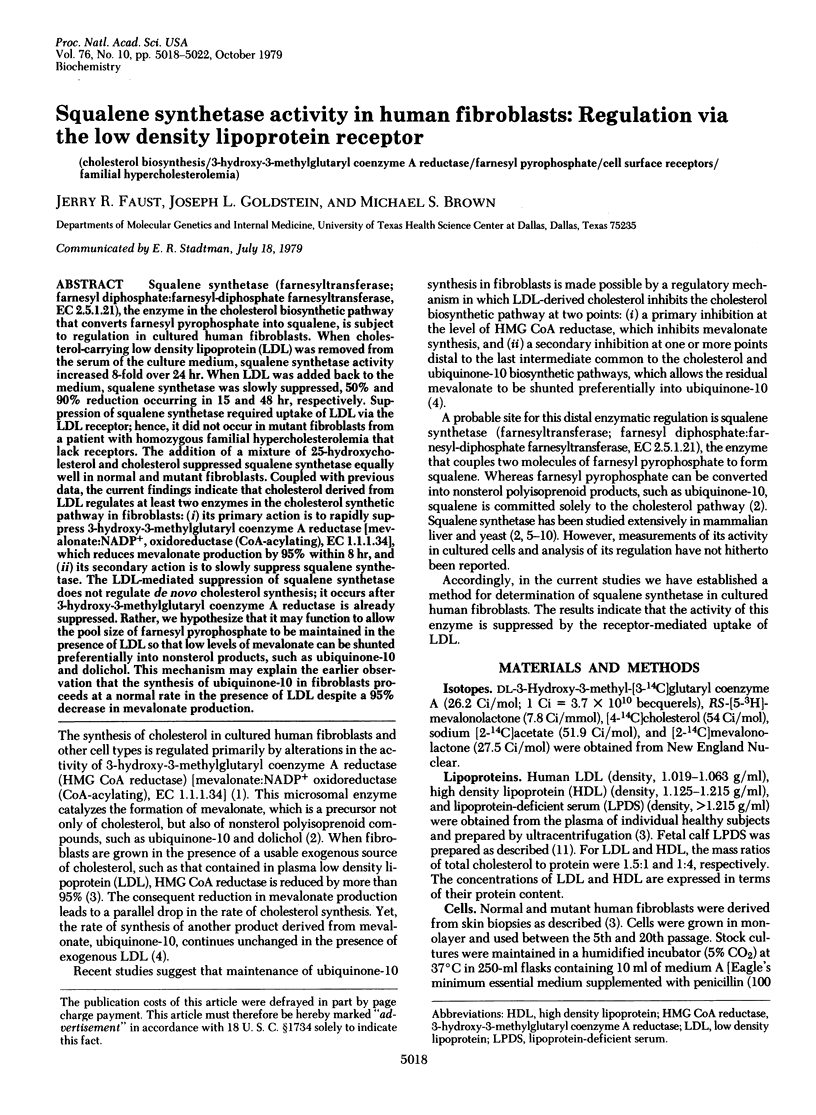
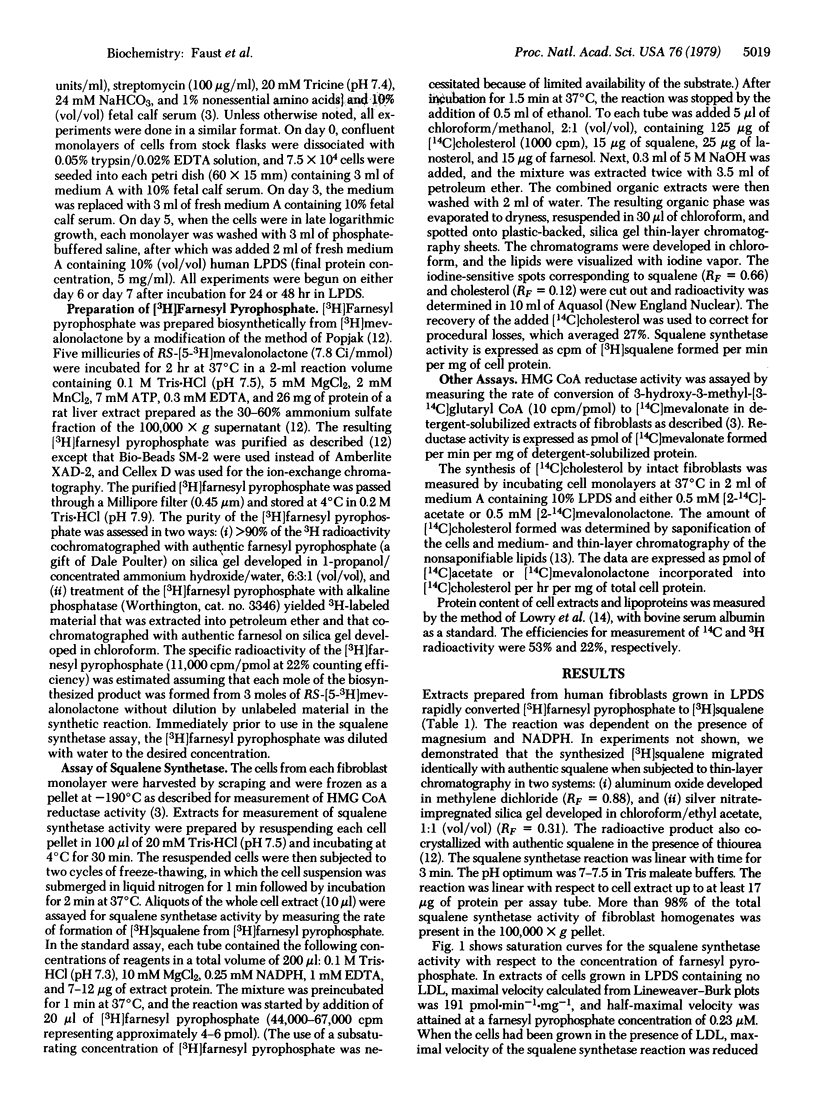
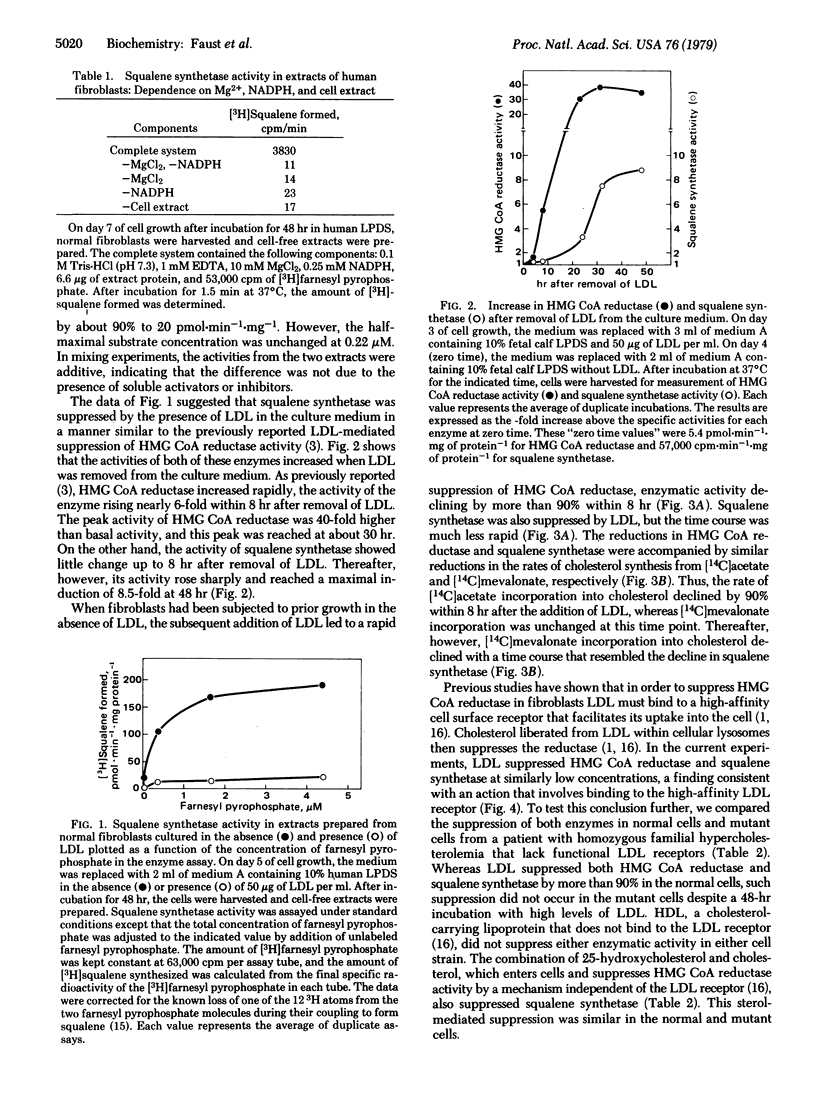
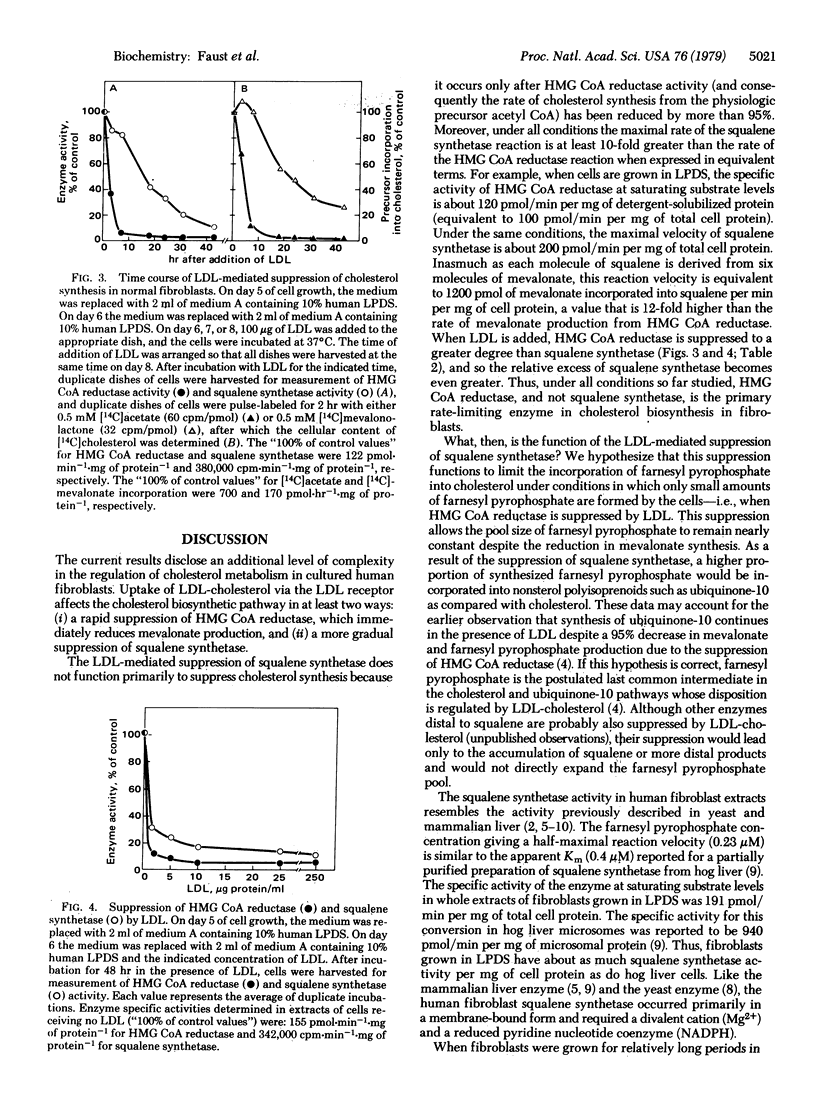
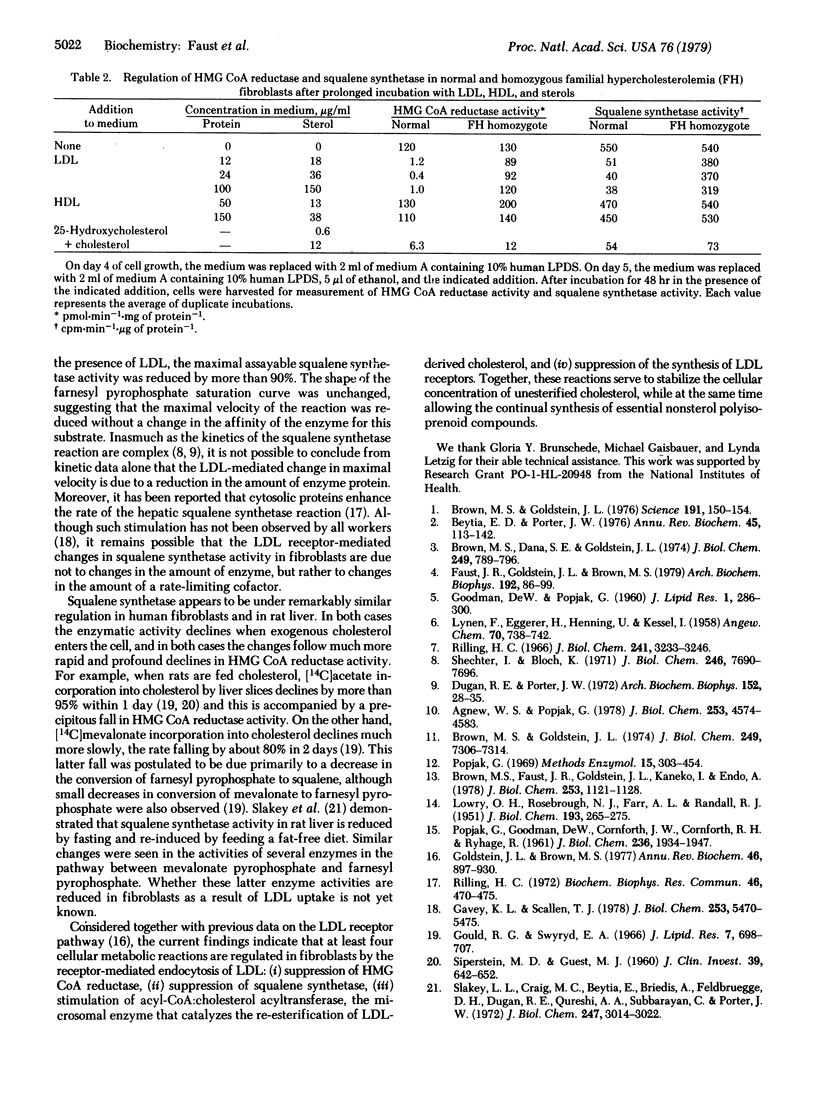
Selected References
These references are in PubMed. This may not be the complete list of references from this article.
- Agnew W. S., Popják G. Squalene synthetase. Solubilization from yeast microsomes of a phospholipid-requiring enzyme. J Biol Chem. 1978 Jul 10;253(13):4574–4583. [PubMed] [Google Scholar]
- Beytía E. D., Porter J. W. Biochemistry of polyisoprenoid biosynthesis. Annu Rev Biochem. 1976;45:113–142. doi: 10.1146/annurev.bi.45.070176.000553. [DOI] [PubMed] [Google Scholar]
- Brown M. S., Dana S. E., Goldstein J. L. Regulation of 3-hydroxy-3-methylglutaryl coenzyme A reductase activity in cultured human fibroblasts. Comparison of cells from a normal subject and from a patient with homozygous familial hypercholesterolemia. J Biol Chem. 1974 Feb 10;249(3):789–796. [PubMed] [Google Scholar]
- Brown M. S., Faust J. R., Goldstein J. L., Kaneko I., Endo A. Induction of 3-hydroxy-3-methylglutaryl coenzyme A reductase activity in human fibroblasts incubated with compactin (ML-236B), a competitive inhibitor of the reductase. J Biol Chem. 1978 Feb 25;253(4):1121–1128. [PubMed] [Google Scholar]
- Brown M. S., Goldstein J. L. Receptor-mediated control of cholesterol metabolism. Science. 1976 Jan 16;191(4223):150–154. doi: 10.1126/science.174194. [DOI] [PubMed] [Google Scholar]
- Brown M. S., Goldstein J. L. Suppression of 3-hydroxy-3-methylglutaryl coenzyme A reductase activity and inhibition of growth of human fibroblasts by 7-ketocholesterol. J Biol Chem. 1974 Nov 25;249(22):7306–7314. [PubMed] [Google Scholar]
- Dugan R. E., Porter J. W. Hog liver squalene synthetase: the partial purification of the particulate enzyme and kinetic analysis of the reaction. Arch Biochem Biophys. 1972 Sep;152(1):28–35. doi: 10.1016/0003-9861(72)90189-0. [DOI] [PubMed] [Google Scholar]
- Faust J. R., Goldstein J. L., Brown M. S. Synthesis of ubiquinone and cholesterol in human fibroblasts: regulation of a branched pathway. Arch Biochem Biophys. 1979 Jan;192(1):86–99. doi: 10.1016/0003-9861(79)90074-2. [DOI] [PubMed] [Google Scholar]
- GOODMAN D. S., POPJAK G. Studies on the biosynthesis of cholesterol. XII. Synthesis of allyl pyrophosphates from mevalonate and their conversion into squalene with liver enzymes. J Lipid Res. 1960 Jul;1:286–300. [PubMed] [Google Scholar]
- Gavey K. L., Scallen T. J. Nonparticipation of 105,000 x g liver supernatant or sterol carrier protein in the enzymatic conversion of farnesyl pyrophosphate to squalene by rat liver microsomes. J Biol Chem. 1978 Aug 10;253(15):5470–5475. [PubMed] [Google Scholar]
- Goldstein J. L., Brown M. S. The low-density lipoprotein pathway and its relation to atherosclerosis. Annu Rev Biochem. 1977;46:897–930. doi: 10.1146/annurev.bi.46.070177.004341. [DOI] [PubMed] [Google Scholar]
- Gould R. G., Swyryd E. A. Sites of control of hepatic cholesterol biosynthesis. J Lipid Res. 1966 Sep;7(5):698–707. [PubMed] [Google Scholar]
- LOWRY O. H., ROSEBROUGH N. J., FARR A. L., RANDALL R. J. Protein measurement with the Folin phenol reagent. J Biol Chem. 1951 Nov;193(1):265–275. [PubMed] [Google Scholar]
- POPJAK G., GOODMAN W. S., CORNFORTH J. W., CORNFORTH R. H., RYHAGE R. Studies on the biosynthesis of cholesterol. XV. Mechanism of squalene biosynthesis from farnesyl pyrophosphate and from mevalonate. J Biol Chem. 1961 Jul;236:1934–1947. [PubMed] [Google Scholar]
- Rilling H. C. A new intermediate in the biosynthesis of squalene. J Biol Chem. 1966 Jul 10;241(13):3233–3236. [PubMed] [Google Scholar]
- Rilling H. C. The effect of sterol carrier protein on squalene synthesis. Biochem Biophys Res Commun. 1972 Jan 31;46(2):470–475. doi: 10.1016/s0006-291x(72)80162-1. [DOI] [PubMed] [Google Scholar]
- SIPERSTEIN M. D., GUEST M. J. Studies on the site of the feedback control of cholesterol synthesis. J Clin Invest. 1960 Apr;39:642–652. doi: 10.1172/JCI104079. [DOI] [PMC free article] [PubMed] [Google Scholar]
- Shechter I., Bloch K. Solubilization and purification of trans-farnesyl pyrophosphate-squalene synthetase. J Biol Chem. 1971 Dec 25;246(24):7690–7696. [PubMed] [Google Scholar]
- Slakey L. L., Craig M. C., Beytia E., Briedis A., Feldbruegge D. H., Dugan R. E., Qureshi A. A., Subbarayan C., Porter J. W. The effects of fasting, refeeding, and time of day on the levels of enzymes effecting the conversion of -hydroxy- -methylglutaryl-coenzyme A to squalene. J Biol Chem. 1972 May 25;247(10):3014–3022. [PubMed] [Google Scholar]