Abstract
Streptococcus mutans Ingbritt was grown anaerobically in a chemostat with a glucose limitation, as well as with an excess of glucose (amino acid limitation) at dilution rates (D) between 0.05 and 0.4 h−1 (mean generation time = 12 to 1.5 h). The glucose-limited culture produced cells having 1.5- to 6.0-fold greater glycolytic activity than the cells from the glucose-excess culture. The preferred substrate for these cells was glucose, with the glycolytic rate for sucrose being only slightly lower; the rate for fructose was half that of glucose. The glycolytic rate of the glucose-limited cells was maximum at D = 0.1 h−1, with a decline in rate as the growth rate approached D = 0.4 h−1. A comparison of the activity of phosphoenolpyruvate phosphotransferase system (PTS) in the two types of cells showed that the glucose-limited cells had 1.7- to 5.6-fold greater PTS activity for the three sugars than the glucose-excess-grown cells. Whereas little difference was seen between the three sugars with the latter cells, the glucose-PTS had the greatest activity with glucose-limited cells, with the maximum in cells grown at D = 0.1 h−1. Comparison of the rate of sugar uptake in the chemostat with the rate of PTS transport activity in the cells at each growth rate demonstrated that only under conditions of slow growth with a glucose limitation was the PTS system capable of supporting growth on glucose. Furthermore, PTS activity in cells grown with an excess of glucose was insignificant when compared with glucose uptake during growth in the chemostat. This evidence supports the observation that S. mutans possesses at least one other system, in addition to the PTS, for the transport of glucose into the cell. The organism was, however, devoid of glucose-proton symport transport activity.
Full text
PDF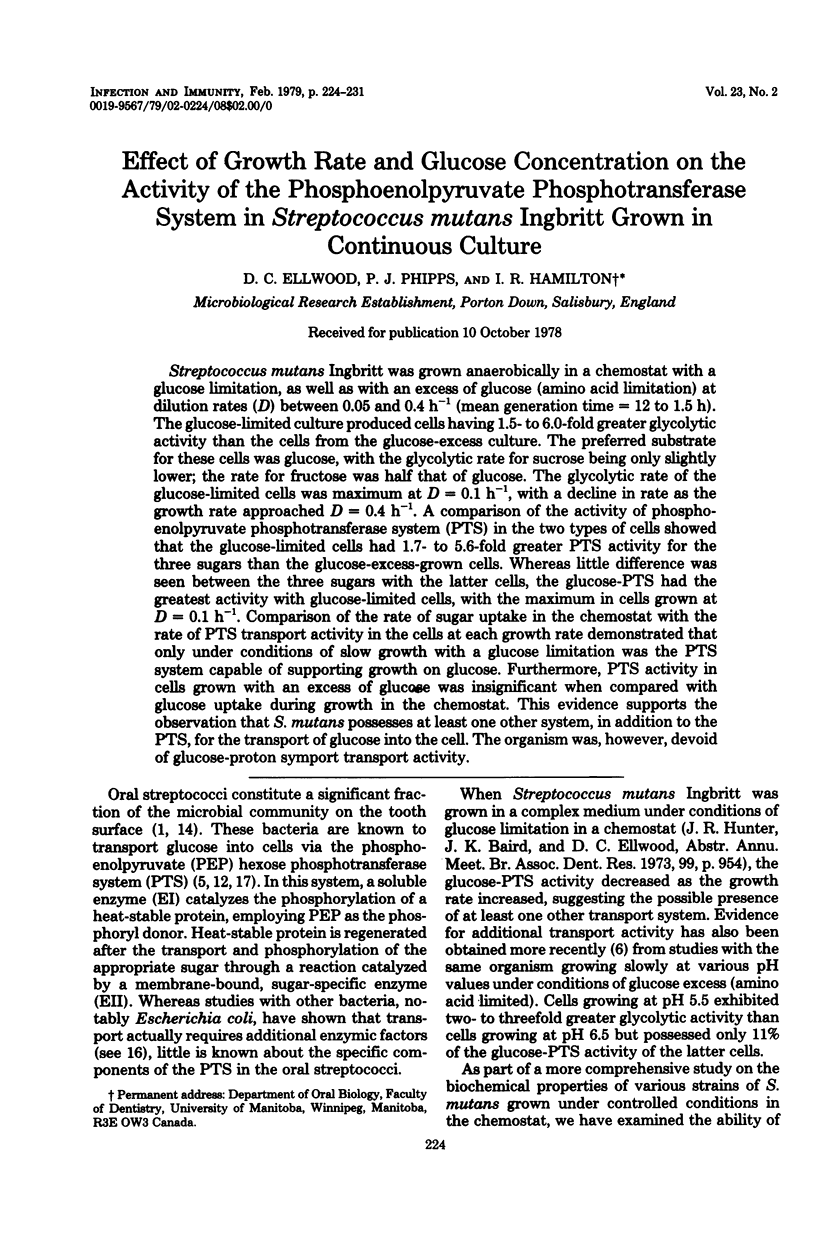
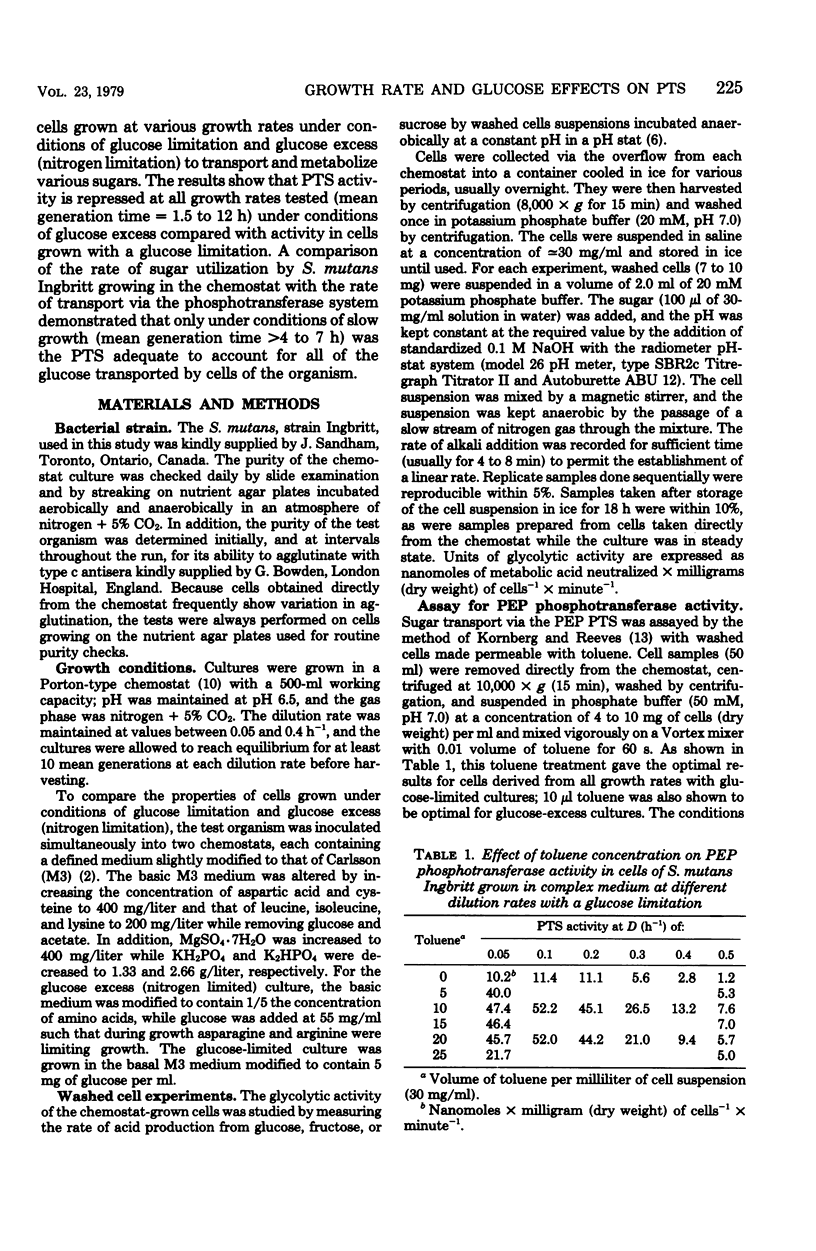
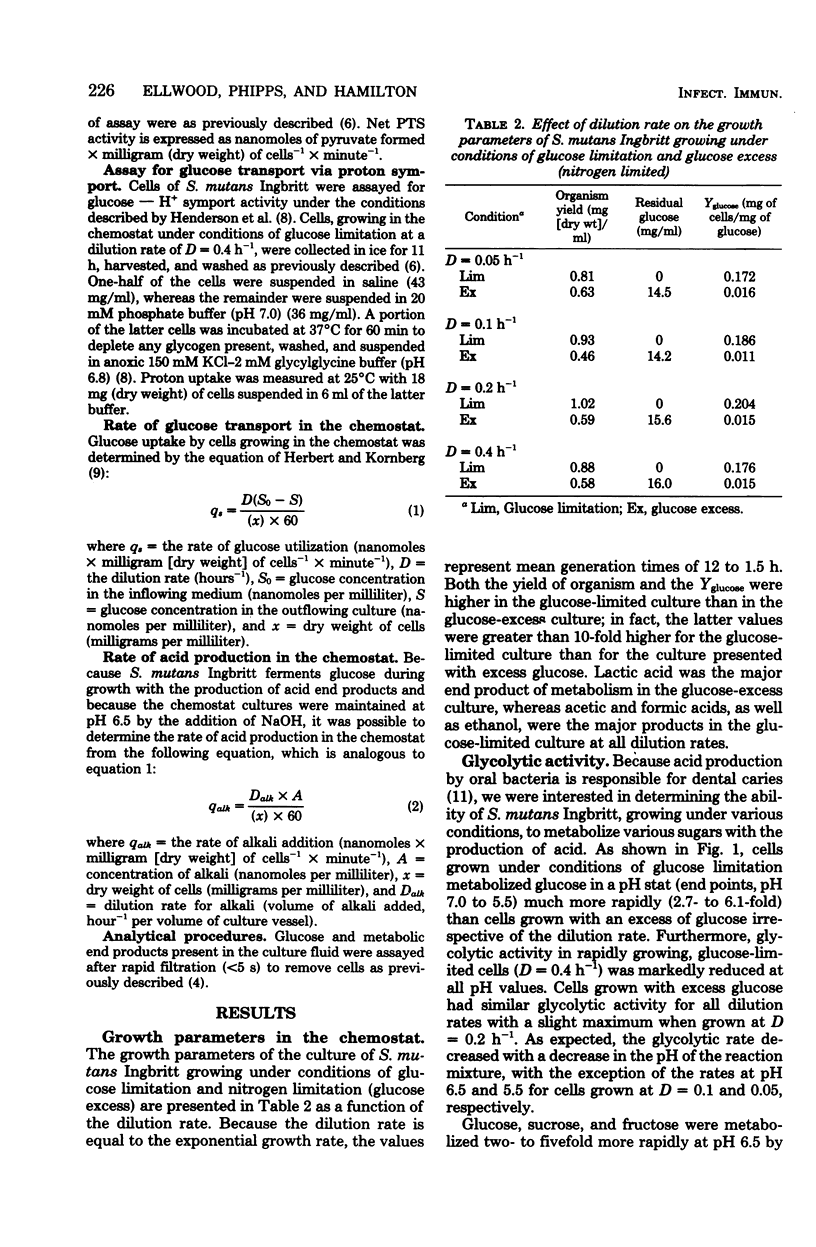
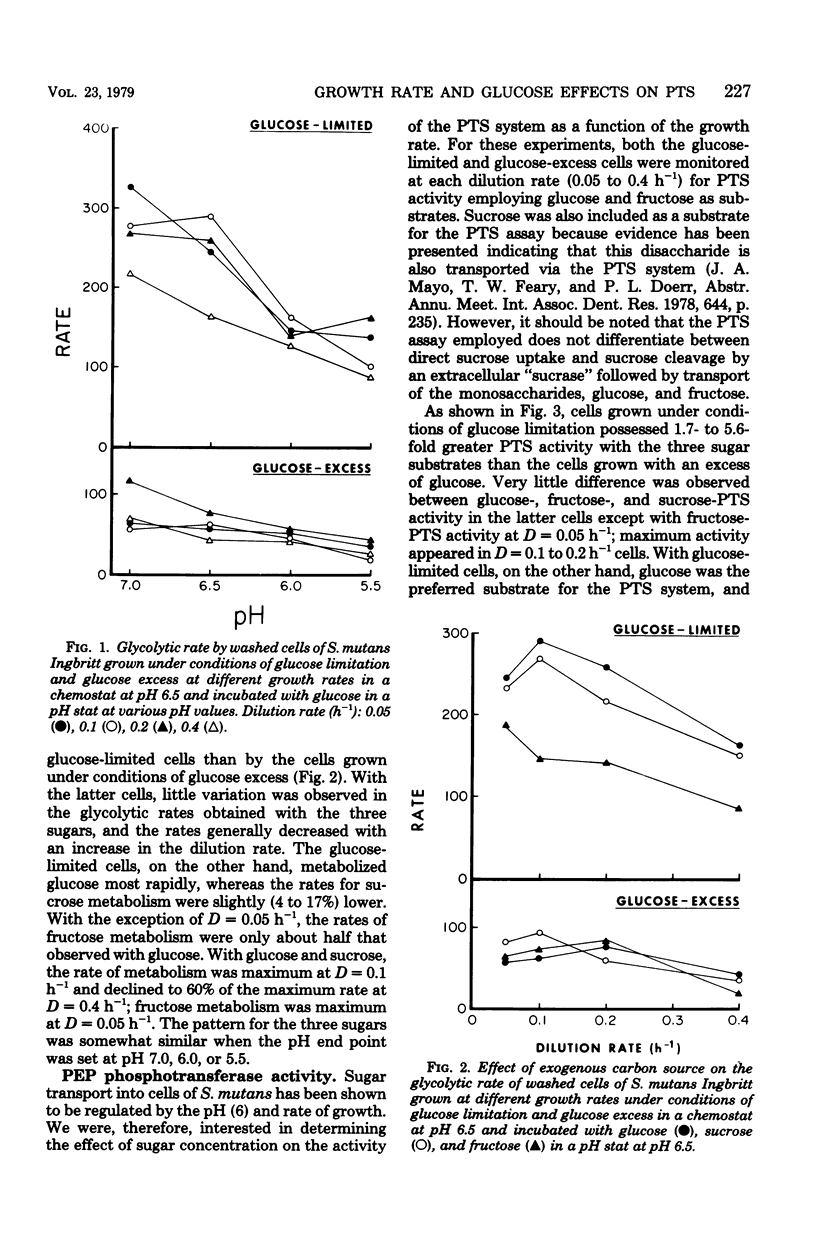
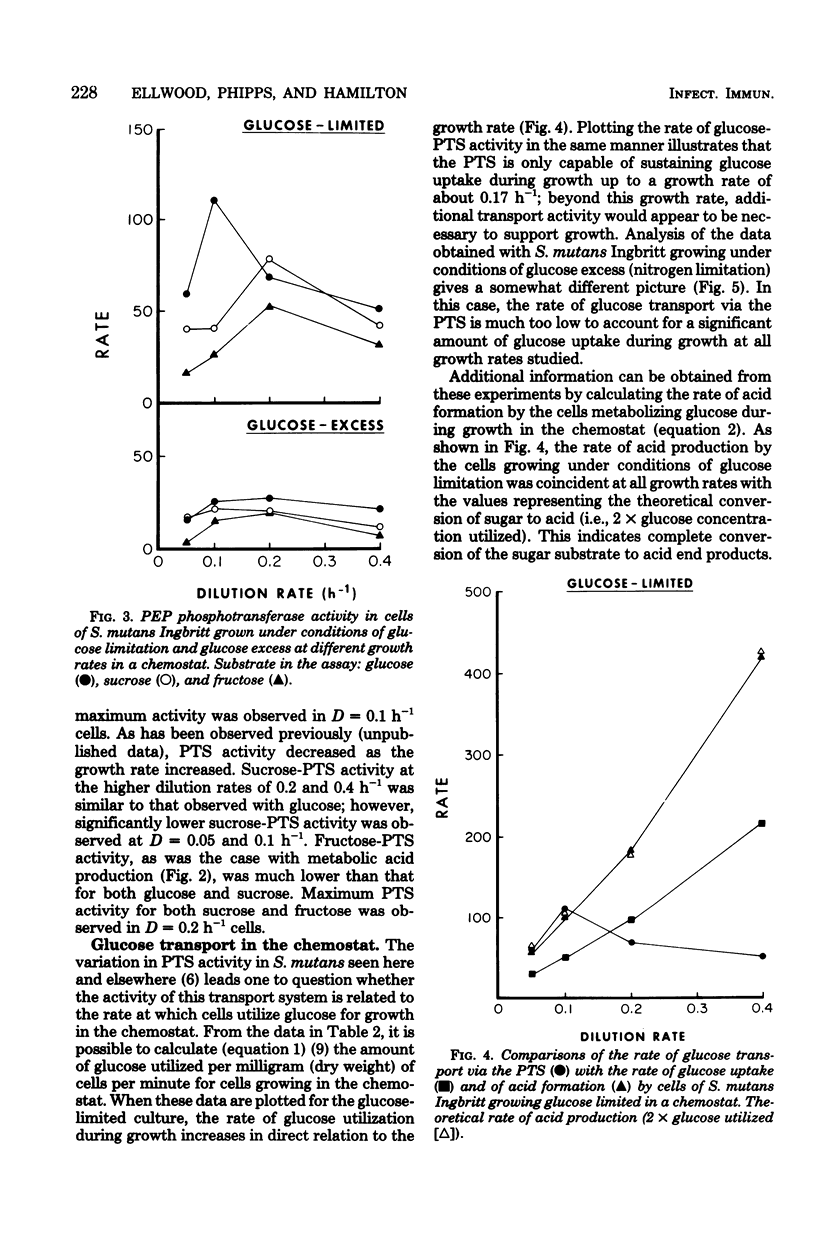
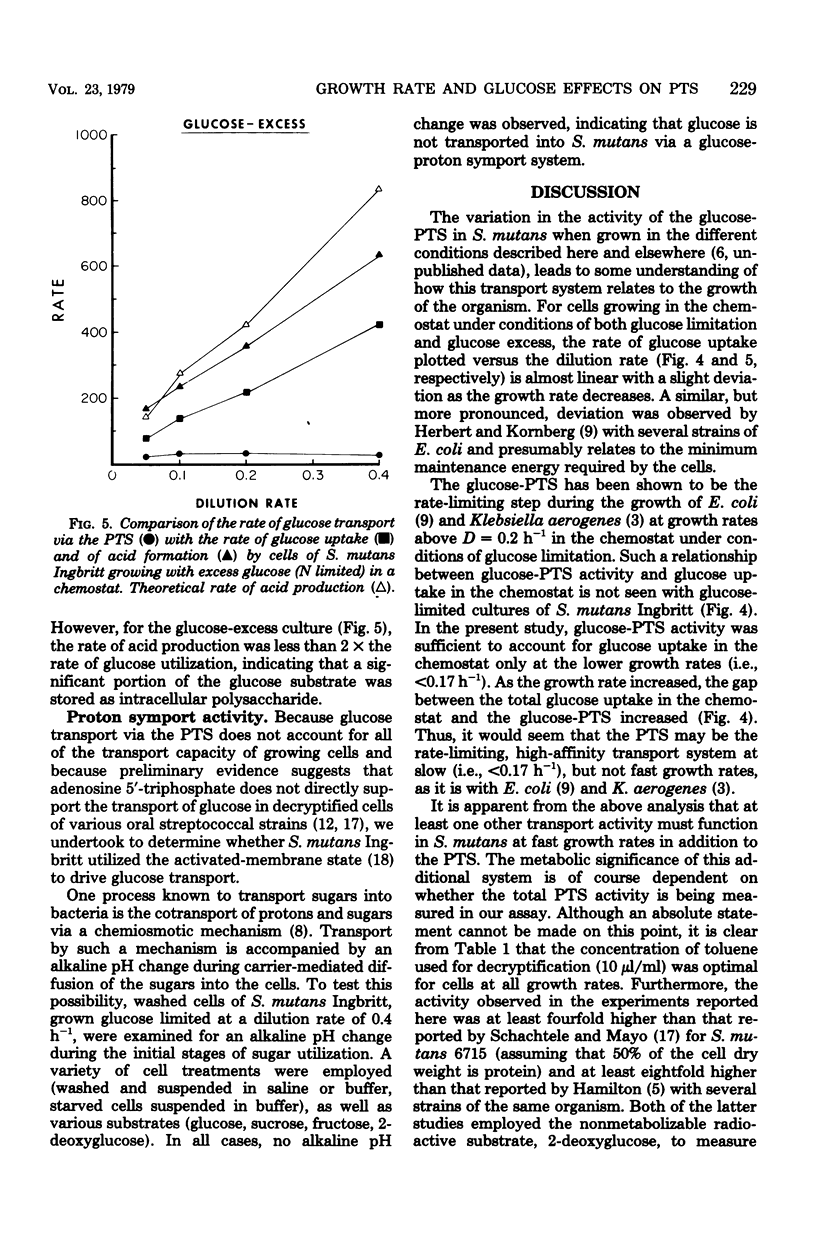
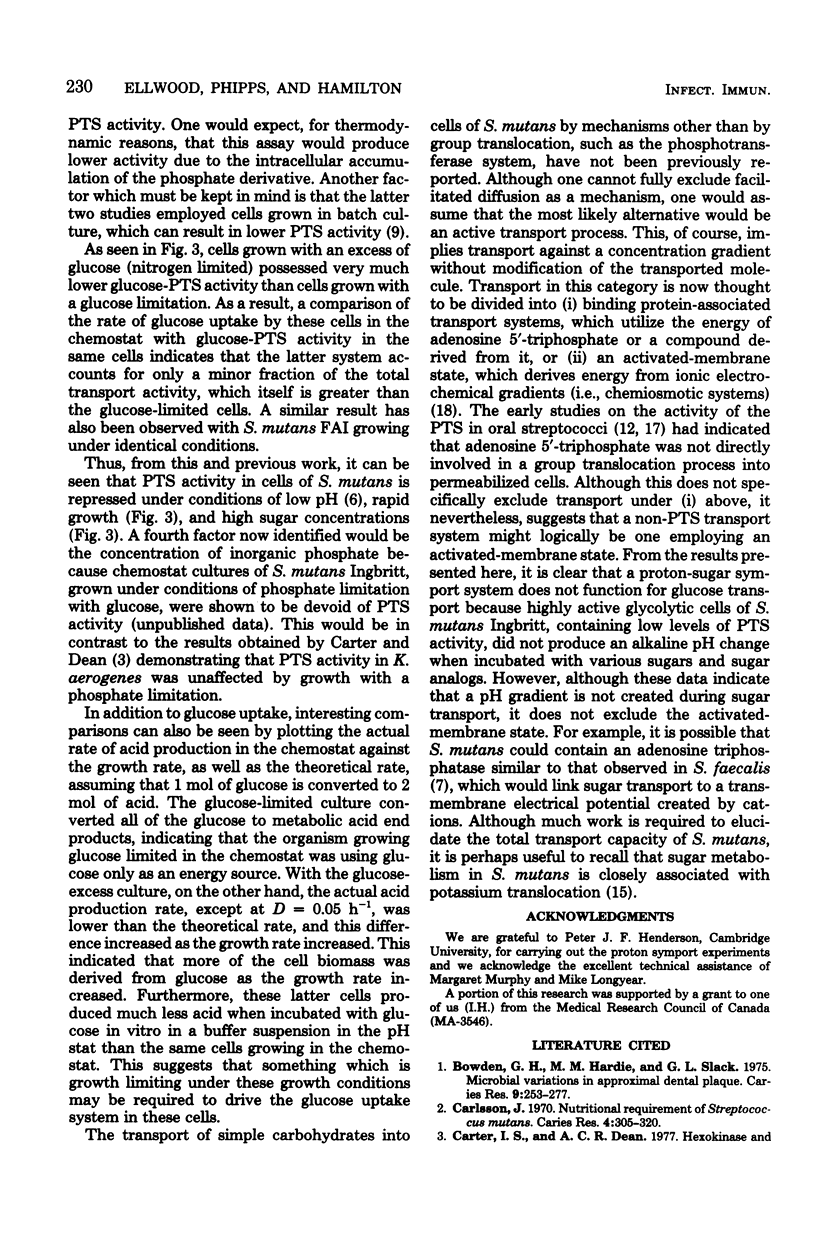
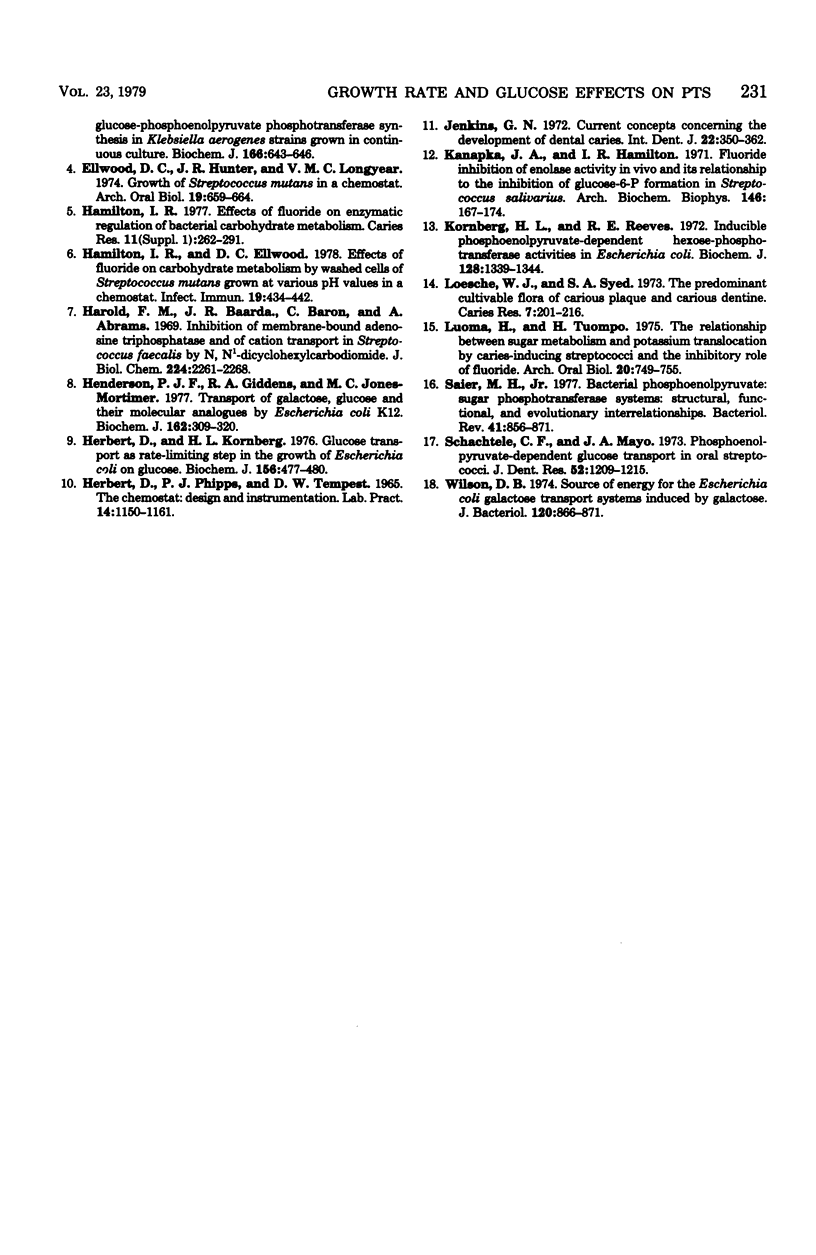
Selected References
These references are in PubMed. This may not be the complete list of references from this article.
- Bowden G. H., Hardie J. M., Slack G. L. Microbial variations in approximal dental plaque. Caries Res. 1975;9(4):253–277. doi: 10.1159/000260162. [DOI] [PubMed] [Google Scholar]
- Carlsson J. Nutritional requirements of Streptococcus mutans. Caries Res. 1970;4(4):305–320. doi: 10.1159/000259653. [DOI] [PubMed] [Google Scholar]
- Carter I. S., Dean A. C. Hexokinase and glucose-phosphoenolpyruvate phosphotransferase synthesis in Klebsiella aerogenes strains growing in continuous culture. Biochem J. 1977 Sep 15;166(3):643–646. doi: 10.1042/bj1660643. [DOI] [PMC free article] [PubMed] [Google Scholar]
- Ellwood D. C., Hunter J. R., Longyear V. M. Growth of Streptococcus mutans in a chemostat. Arch Oral Biol. 1974 Aug;19(8):659–664. doi: 10.1016/0003-9969(74)90134-4. [DOI] [PubMed] [Google Scholar]
- Hamilton I. R. Effects of fluoride on enzymatic regulation of bacterial carbohydrate metabolism. Caries Res. 1977;11 (Suppl 1):262–291. doi: 10.1159/000260304. [DOI] [PubMed] [Google Scholar]
- Hamilton I. R., Ellwood D. C. Effects of fluoride on carbohydrate metabolism by washed cells of Streptococcus mutans grown at various pH values in a chemostat. Infect Immun. 1978 Feb;19(2):434–442. doi: 10.1128/iai.19.2.434-442.1978. [DOI] [PMC free article] [PubMed] [Google Scholar]
- Harold F. M., Baarda J. R., Baron C., Abrams A. Inhibition of membrane-bound adenosine triphosphatase and of cation transport in Streptococcus faecalis by N,N'-dicyclohexylcarbodiimide. J Biol Chem. 1969 May 10;244(9):2261–2268. [PubMed] [Google Scholar]
- Henderson P. J., Giddens R. A., Jones-Mortimer M. C. Transport of galactose, glucose and their molecular analogues by Escherichia coli K12. Biochem J. 1977 Feb 15;162(2):309–320. doi: 10.1042/bj1620309. [DOI] [PMC free article] [PubMed] [Google Scholar]
- Herbert D., Kornberg H. L. Glucose transport as rate-limiting step in the growth of Escherichia coli on glucose. Biochem J. 1976 May 15;156(2):477–480. doi: 10.1042/bj1560477. [DOI] [PMC free article] [PubMed] [Google Scholar]
- Herbert D., Phipps P. J., Tempest D. W. The chemostat: design and instrumentation. Lab Pract. 1965 Oct;14(10):1150–1161. [PubMed] [Google Scholar]
- Jenkins G. N. Current concepts concerning the development of dental caries. Int Dent J. 1972 Sep;22(3):350–361. [PubMed] [Google Scholar]
- Kanapka J. A., Hamilton I. R. Fluoride inhibition of enolase activity in vivo and its relationship to the inhibition of glucose-6-P formation in Streptococcus salivarius. Arch Biochem Biophys. 1971 Sep;146(1):167–174. doi: 10.1016/s0003-9861(71)80053-x. [DOI] [PubMed] [Google Scholar]
- Kornberg H. L., Reeves R. E. Inducible phosphoenolpyruvate-dependent hexose phosphotransferase activities in Escherichia coli. Biochem J. 1972 Aug;128(5):1339–1344. doi: 10.1042/bj1281339. [DOI] [PMC free article] [PubMed] [Google Scholar]
- Loesche W. J., Syed S. A. The predominant cultivable flora of carious plaque and carious dentine. Caries Res. 1973;7(3):201–216. doi: 10.1159/000259844. [DOI] [PubMed] [Google Scholar]
- Luoma H., Tuompo H. The relationship between sugar metabolism and potassium translocation by caries-inducing streptococci and the inhibitory role of fluoride. Arch Oral Biol. 1975 Nov;20(11):749–755. doi: 10.1016/0003-9969(75)90047-3. [DOI] [PubMed] [Google Scholar]
- Saier M. H., Jr Bacterial phosphoenolpyruvate: sugar phosphotransferase systems: structural, functional, and evolutionary interrelationships. Bacteriol Rev. 1977 Dec;41(4):856–871. doi: 10.1128/br.41.4.856-871.1977. [DOI] [PMC free article] [PubMed] [Google Scholar]
- Schachtele C. F., Mayo J. A. Phosphoenolpyruvate-dependent glucose transport in oral streptococci. J Dent Res. 1973 Nov-Dec;52(6):1209–1215. doi: 10.1177/00220345730520060801. [DOI] [PubMed] [Google Scholar]
- Wilson D. B. Source of energy for the Escherichia coli galactose transport systems induced by galactose. J Bacteriol. 1974 Nov;120(2):866–871. doi: 10.1128/jb.120.2.866-871.1974. [DOI] [PMC free article] [PubMed] [Google Scholar]