Abstract
A phosphoenolpyruvate-dependent sucrose phosphotransferase system (PTS) has been demonstrated, by an enzyme-coupled reaction and product isolation, in decryptified cell suspensions of the cariogenic microorganism Streptococcus mutans NCTC 10449. The apparent sucrose PTS reaction for sucrose-adapted, sucrose-challenged cells displayed saturation kinetics with an apparent Km of 7.14 x 10(-5) M, which was distinct from the Km of the glucose PTS activity of glucose-adapted, glucose-challenged cells. Both the sucrose and the glucose PTS activities appear to be inducible and under separate genetic control. The sucrose PTS reaction demonstrated in decryptified cells had an absolute requirement for phosphoenolpyruvate. Only 2-phosphoglycerate, the immediate glycolytic precursor of phosphoenolpyruvate, was found to substitute for phosphoenolpyruvate in this reaction in the absence of fluoride. The sucrose PTS activity of sucrose-adapted cells was competitively inhibited by raffinose and lactose; these same sugars had no effect on the apparent glucose PTS activity. Fructose was the only carbohydrate tested other than sucrose which elicited an apparent PTS reaction in sucrose-adapted cells. The product of the sucrose PTS reaction was isolated and behaved chromatographically on a Dowex-1-X8 column like a monophosphate ester. Alkaline phosphatase treatment of the presumptive sucrose monophosphate liberated a component which behaved chromatographically like free sucrose. Subsequent acid hydrolysis of this component produced moieties which behaved chromatographically like glucose and fructose.
Full text
PDF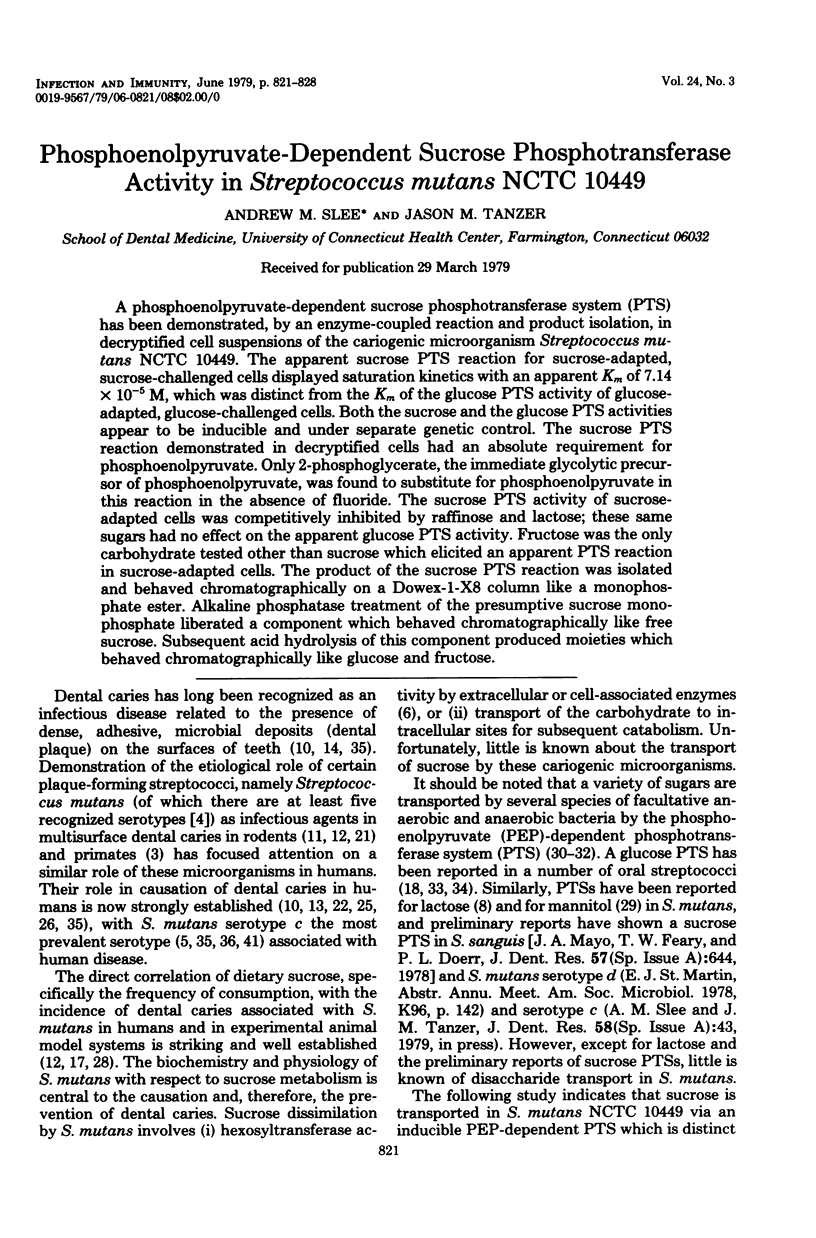
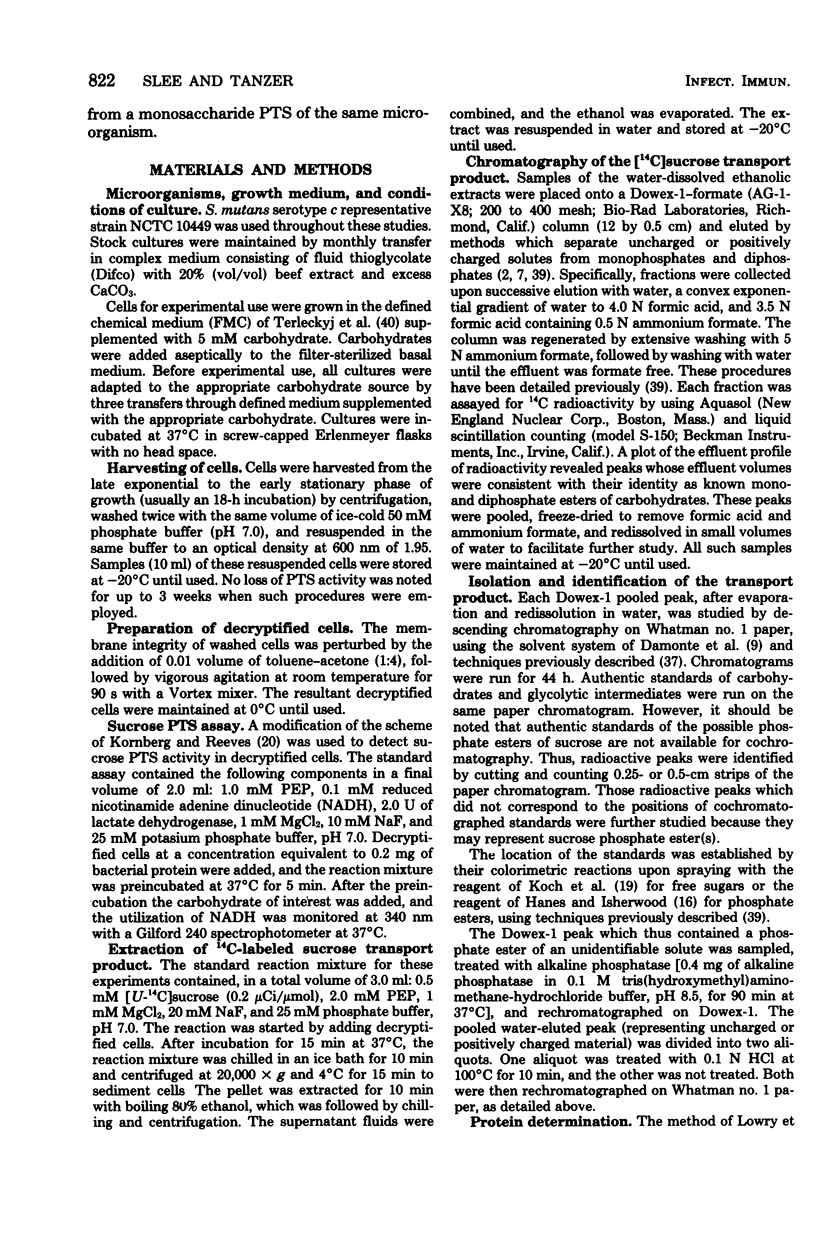
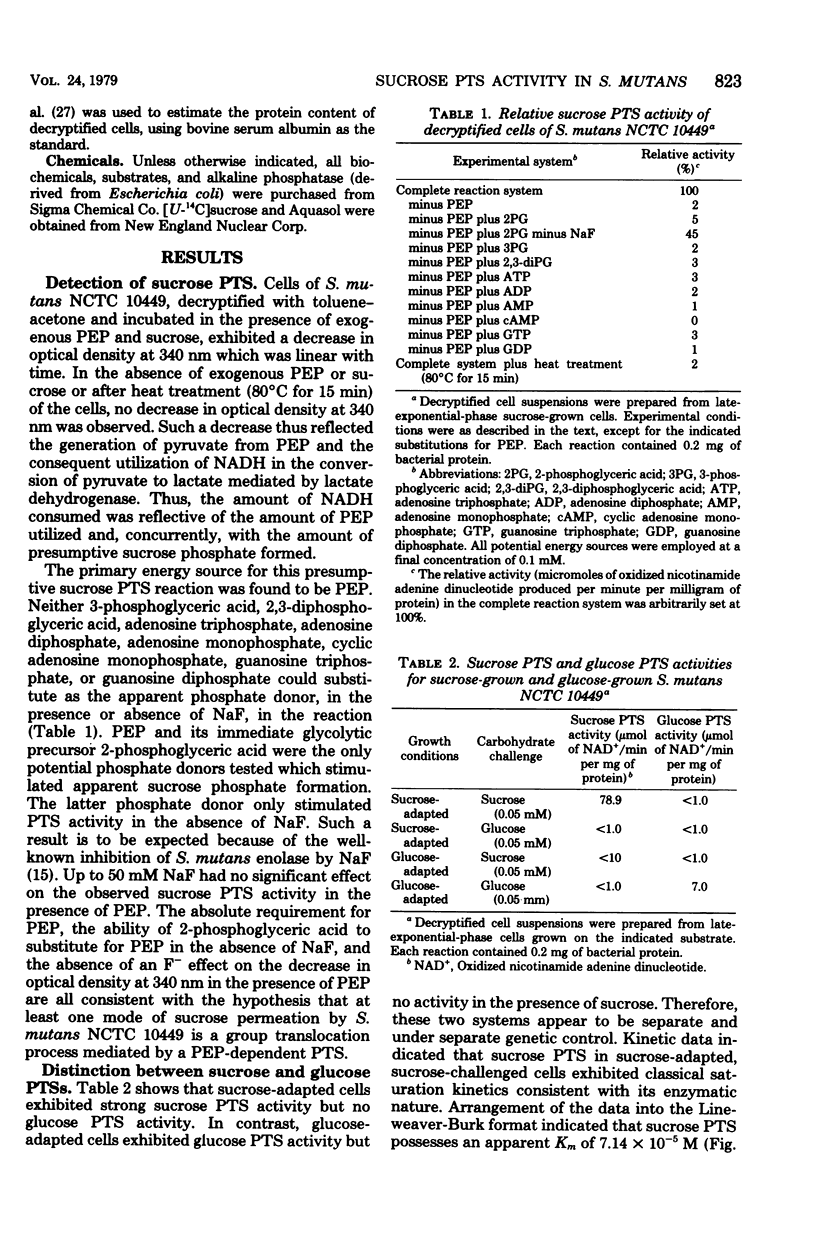
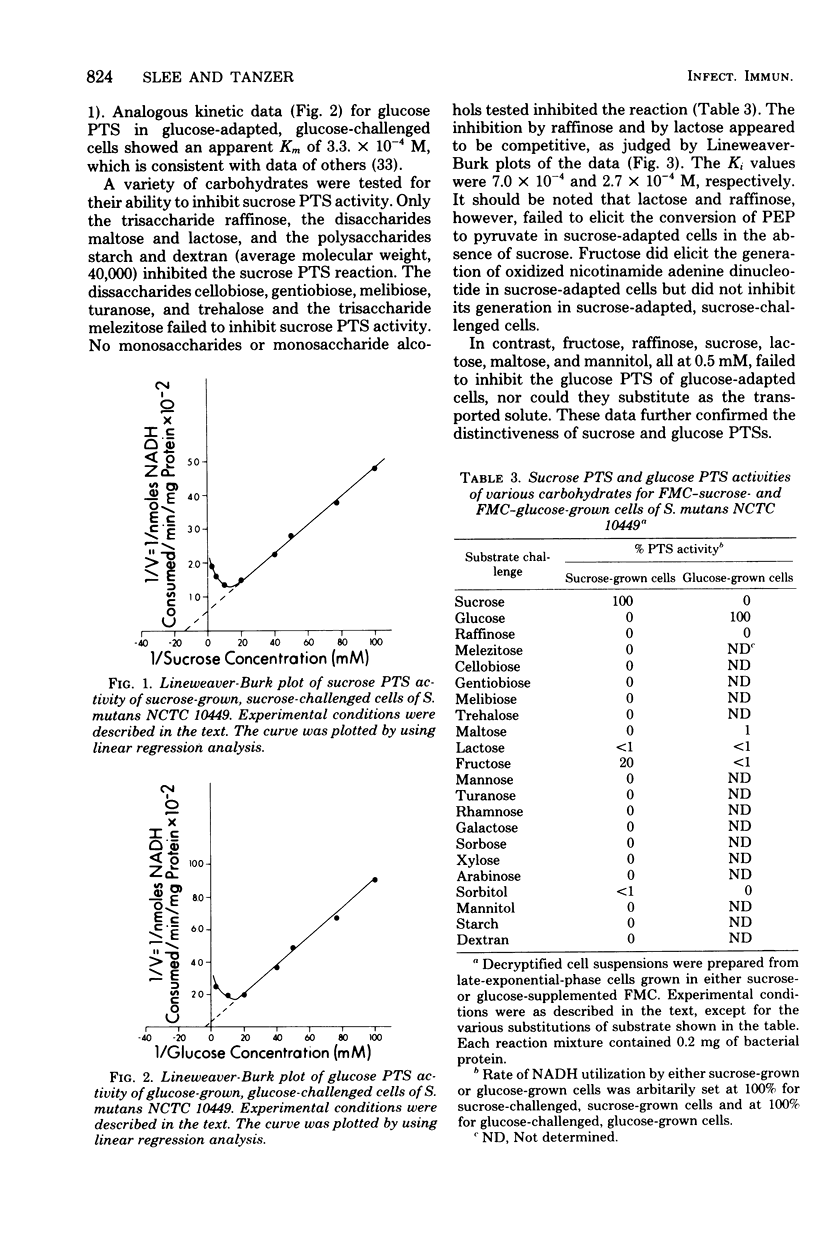
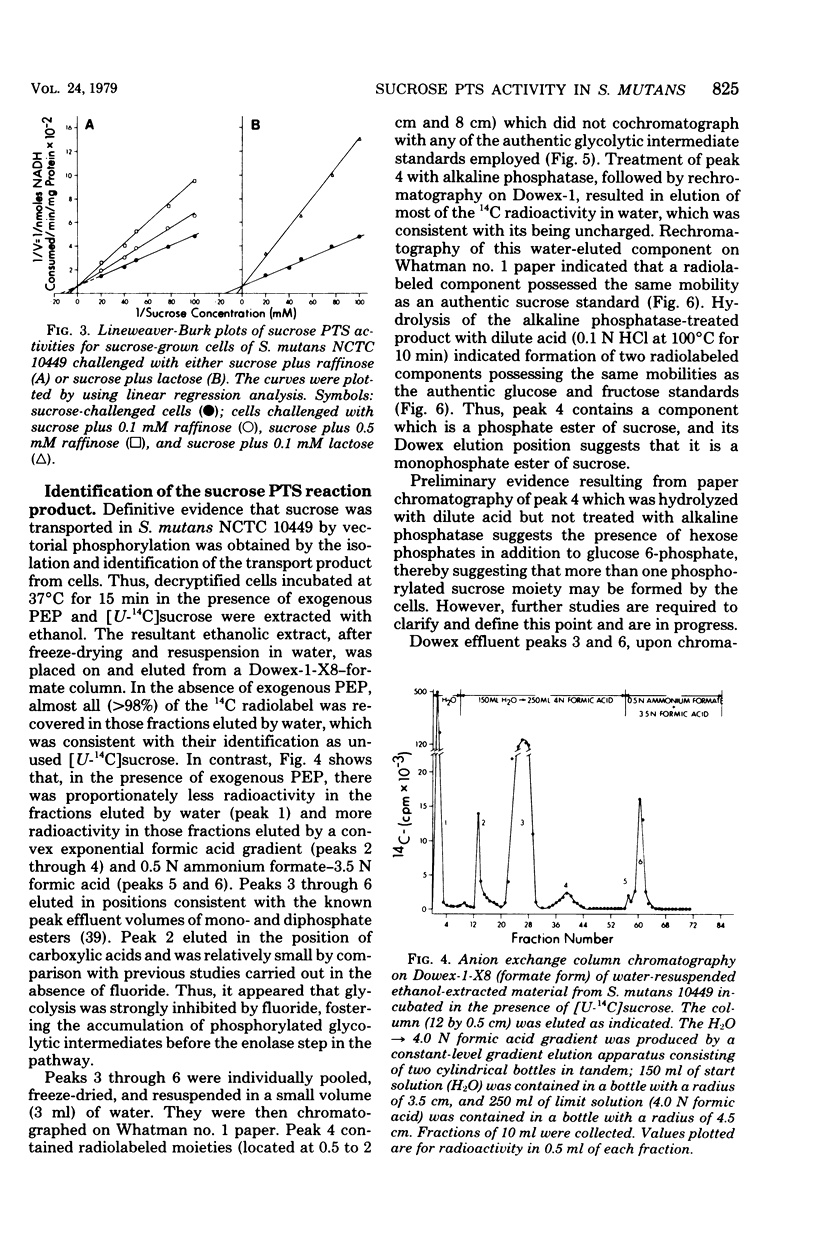
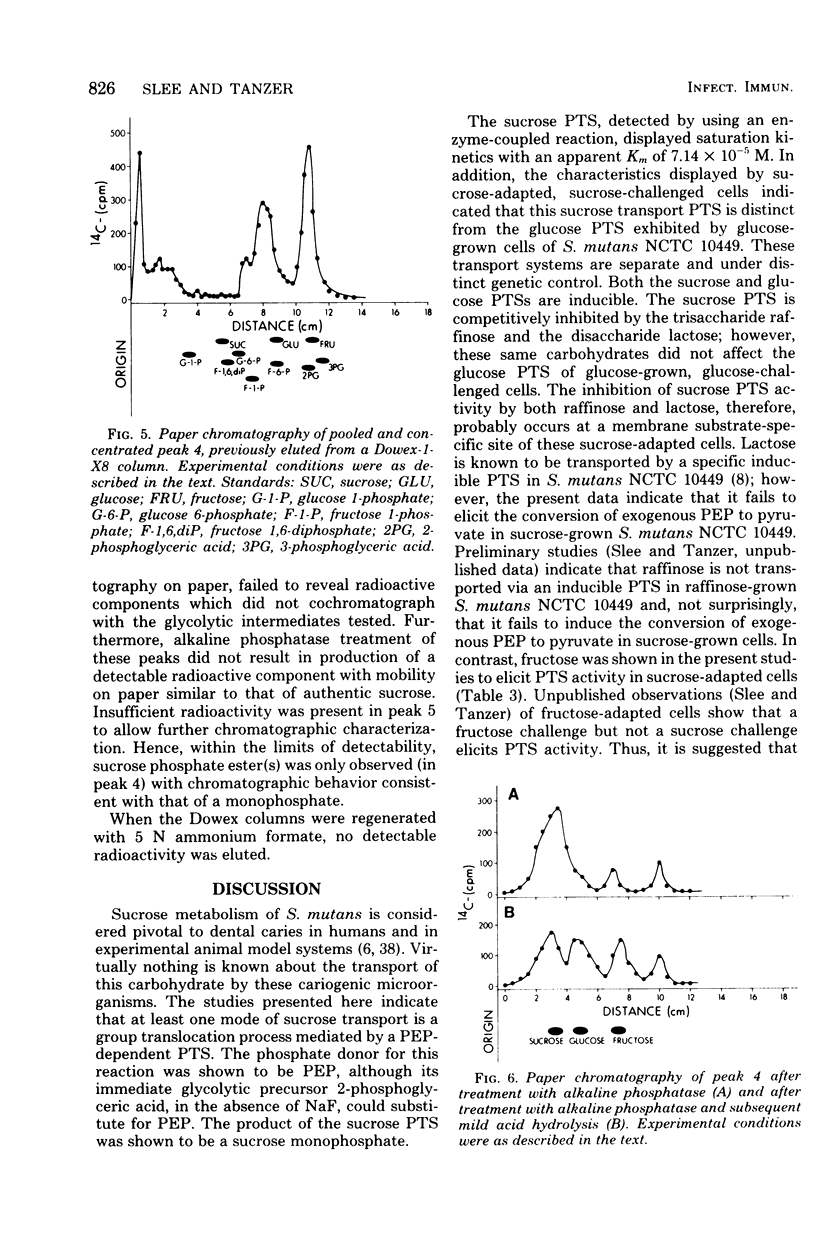
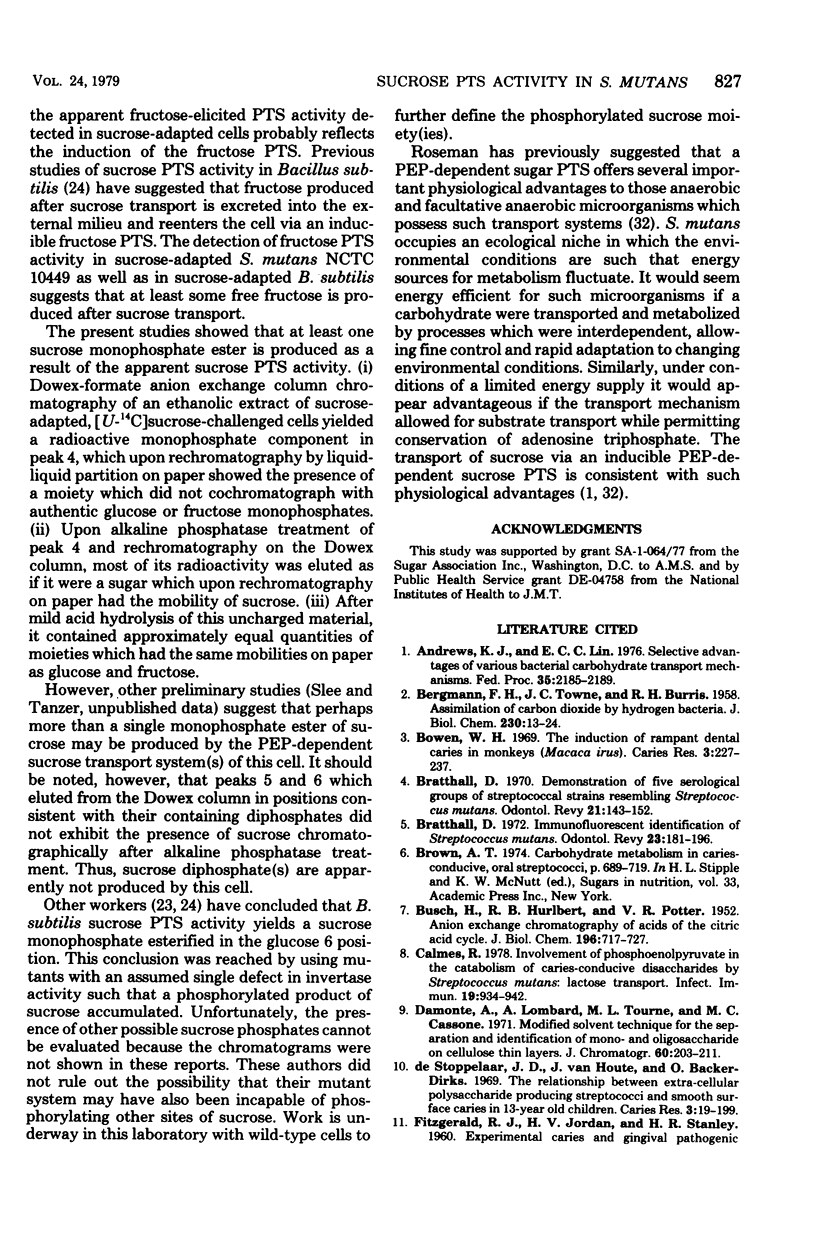
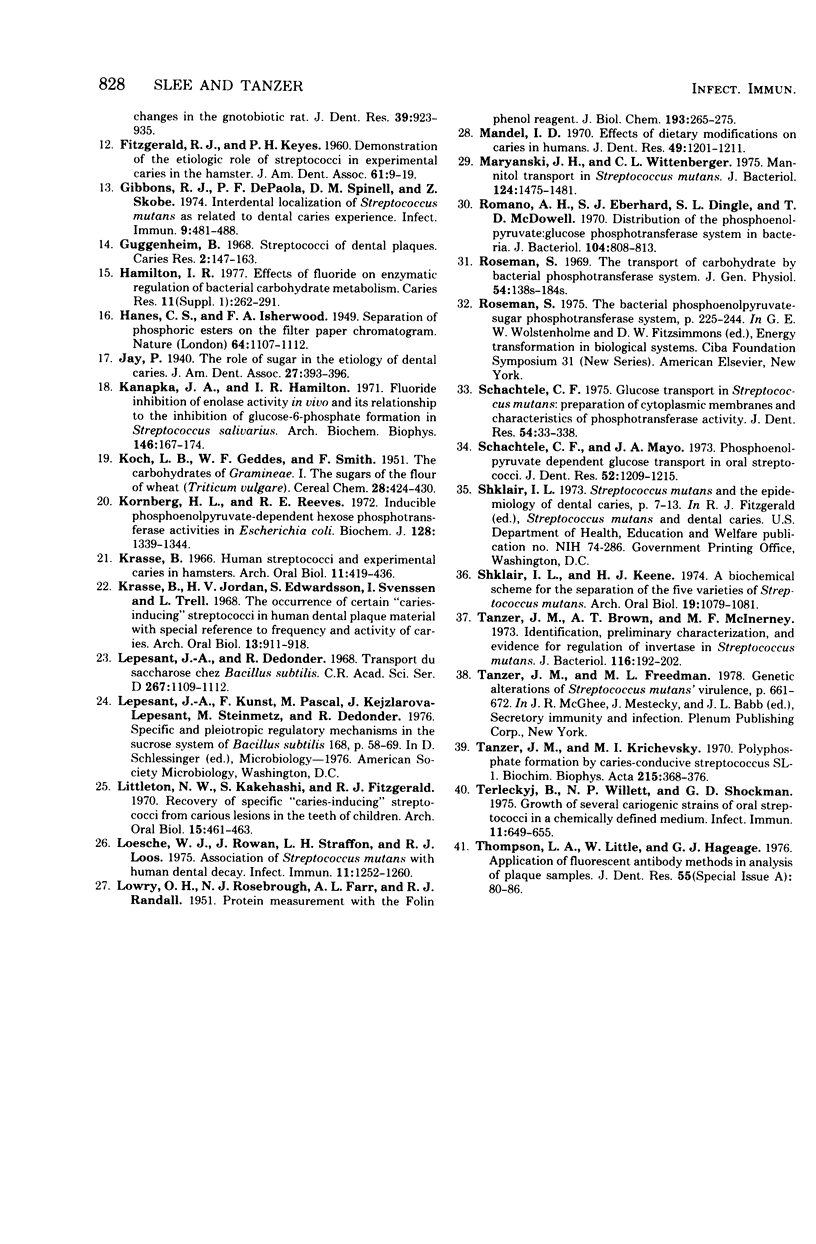
Selected References
These references are in PubMed. This may not be the complete list of references from this article.
- Andrews K. J., Lin E. C. Selective advantages of various bacterial carbohydrate transport mechanisms. Fed Proc. 1976 Aug;35(10):2185–2189. [PubMed] [Google Scholar]
- BERGMANN F. H., TOWNE J. C., BURRIS R. H. Assimilation of carbon dioxide by hydrogen bacteria. J Biol Chem. 1958 Jan;230(1):13–24. [PubMed] [Google Scholar]
- BUSCH H., HURLBERT R. B., POTTER V. R. Anion exchange chromatography of acids of the citric acid cycle. J Biol Chem. 1952 May;196(2):717–727. [PubMed] [Google Scholar]
- Bowen W. H. The induction of rampant dental caries in monkeys (Macaca irus). Caries Res. 1969;3(3):227–237. doi: 10.1159/000259597. [DOI] [PubMed] [Google Scholar]
- Bratthall D. Demonstration of five serological groups of streptococcal strains resembling Streptococcus mutans. Odontol Revy. 1970;21(2):143–152. [PubMed] [Google Scholar]
- Bratthall D. Immunofluorescent identification of Streptococcus mutans. Odontol Revy. 1972;23(2):181–196. [PubMed] [Google Scholar]
- Calmes R. Involvement of phosphoenolpyruvate in the catabolism of caries-conducive disaccharides by Streptococcus mutans: lactose transport. Infect Immun. 1978 Mar;19(3):934–942. doi: 10.1128/iai.19.3.934-942.1978. [DOI] [PMC free article] [PubMed] [Google Scholar]
- De Stoppelaar J. D., Van Houte J., Backer Dirks O. The relationship between extracellular polysaccharide-producing streptococci and smooth surface caries in 13-year-old children. Caries Res. 1969;3(2):190–199. doi: 10.1159/000259582. [DOI] [PubMed] [Google Scholar]
- FITZGERALD R. J., JORDAN H. V., STANLEY H. R. Experimental caries and gingival pathologic changes in the gnotobiotic rat. J Dent Res. 1960 Sep-Oct;39:923–935. doi: 10.1177/00220345600390052701. [DOI] [PubMed] [Google Scholar]
- FITZGERALD R. J., KEYES P. H. Demonstration of the etiologic role of streptococci in experimental caries in the hamster. J Am Dent Assoc. 1960 Jul;61:9–19. doi: 10.14219/jada.archive.1960.0138. [DOI] [PubMed] [Google Scholar]
- Gibbons R. J., Depaola P. F., Spinell D. M., Skobe Z. Interdental localization of Streptococcus mutans as related to dental caries experience. Infect Immun. 1974 Mar;9(3):481–488. doi: 10.1128/iai.9.3.481-488.1974. [DOI] [PMC free article] [PubMed] [Google Scholar]
- Guggenheim B. Streptococci of dental plaques. Caries Res. 1968;2(2):147–163. doi: 10.1159/000259553. [DOI] [PubMed] [Google Scholar]
- HANES C. S., ISHERWOOD F. A. Separation of the phosphoric esters on the filter paper chromatogram. Nature. 1949 Dec 31;164(4183):1107-12, illust. doi: 10.1038/1641107a0. [DOI] [PubMed] [Google Scholar]
- Hamilton I. R. Effects of fluoride on enzymatic regulation of bacterial carbohydrate metabolism. Caries Res. 1977;11 (Suppl 1):262–291. doi: 10.1159/000260304. [DOI] [PubMed] [Google Scholar]
- Kanapka J. A., Hamilton I. R. Fluoride inhibition of enolase activity in vivo and its relationship to the inhibition of glucose-6-P formation in Streptococcus salivarius. Arch Biochem Biophys. 1971 Sep;146(1):167–174. doi: 10.1016/s0003-9861(71)80053-x. [DOI] [PubMed] [Google Scholar]
- Kornberg H. L., Reeves R. E. Inducible phosphoenolpyruvate-dependent hexose phosphotransferase activities in Escherichia coli. Biochem J. 1972 Aug;128(5):1339–1344. doi: 10.1042/bj1281339. [DOI] [PMC free article] [PubMed] [Google Scholar]
- Krasse B. Human streptococci and experimental caries in hamsters. Arch Oral Biol. 1966 Apr;11(4):429–436. doi: 10.1016/0003-9969(66)90107-5. [DOI] [PubMed] [Google Scholar]
- Krasse B., Jordan H. V., Edwardsson S., Svensson I., Trell L. The occurrence of certain "caries-inducing" streptococci in human dental plaque material with special reference to frequency and activity of caries. Arch Oral Biol. 1968 Aug;13(8):911–918. doi: 10.1016/0003-9969(68)90006-x. [DOI] [PubMed] [Google Scholar]
- LOWRY O. H., ROSEBROUGH N. J., FARR A. L., RANDALL R. J. Protein measurement with the Folin phenol reagent. J Biol Chem. 1951 Nov;193(1):265–275. [PubMed] [Google Scholar]
- Lepesant J. A., Dedonder R. Transport du saccharose chez Bacillus subtilis. C R Acad Sci Hebd Seances Acad Sci D. 1968 Sep 23;267(13):1109–1112. [PubMed] [Google Scholar]
- Littleton N. W., Kakehashi S., Fitzgerald R. J. Recovery of specific "caries-inducing" streptococci from carious lesions in the teeth of children. Arch Oral Biol. 1970 May;15(5):461–463. doi: 10.1016/0003-9969(70)90073-7. [DOI] [PubMed] [Google Scholar]
- Loesche W. J., Rowan J., Straffon L. H., Loos P. J. Association of Streptococcus mutants with human dental decay. Infect Immun. 1975 Jun;11(6):1252–1260. doi: 10.1128/iai.11.6.1252-1260.1975. [DOI] [PMC free article] [PubMed] [Google Scholar]
- Mandel I. D. Effects of dietary modifications on caries in humans. J Dent Res. 1970 Nov-Dec;49(6):1201–1211. doi: 10.1177/00220345700490060501. [DOI] [PubMed] [Google Scholar]
- Maryanski J. H., Wittenberger C. L. Mannitol transport in Streptococcus mutans. J Bacteriol. 1975 Dec;124(3):1475–1481. doi: 10.1128/jb.124.3.1475-1481.1975. [DOI] [PMC free article] [PubMed] [Google Scholar]
- Romano A. H., Eberhard S. J., Dingle S. L., McDowell T. D. Distribution of the phosphoenolpyruvate: glucose phosphotransferase system in bacteria. J Bacteriol. 1970 Nov;104(2):808–813. doi: 10.1128/jb.104.2.808-813.1970. [DOI] [PMC free article] [PubMed] [Google Scholar]
- Roseman S. The bacterial phosphoenolpyruvate: sugar phosphotransferase system. Ciba Found Symp. 1975;(31):225–241. doi: 10.1002/9780470720134.ch13. [DOI] [PubMed] [Google Scholar]
- Schachtele C. F. Glucose transport in Streptococcus mutans: preparation of cytoplasmic membranes and characteristics of phosphotransferase activity. J Dent Res. 1975 Mar-Apr;54(2):330–338. [PubMed] [Google Scholar]
- Schachtele C. F., Mayo J. A. Phosphoenolpyruvate-dependent glucose transport in oral streptococci. J Dent Res. 1973 Nov-Dec;52(6):1209–1215. doi: 10.1177/00220345730520060801. [DOI] [PubMed] [Google Scholar]
- Shklair I. L., Keene H. J. A biochemical scheme for the separation of the five varieties of Streptococcus mutans. Arch Oral Biol. 1974 Nov;19(11):1079–1081. doi: 10.1016/0003-9969(74)90099-5. [DOI] [PubMed] [Google Scholar]
- Tanzer J. M., Brown A. T., McInerney M. F. Identification, preliminary characterization, and evidence for regulation of invertase in Streptococcus mutans. J Bacteriol. 1973 Oct;116(1):192–202. doi: 10.1128/jb.116.1.192-202.1973. [DOI] [PMC free article] [PubMed] [Google Scholar]
- Tanzer J. M., Freedman M. L. Genetic alterations of Streptococcus mutans' virulence. Adv Exp Med Biol. 1978;107:661–672. doi: 10.1007/978-1-4684-3369-2_75. [DOI] [PubMed] [Google Scholar]
- Tanzer J. M., Krichevsky M. I. Polyphosphate formation by caries-conducive streptococcus SL-I. Biochim Biophys Acta. 1970 Aug 14;215(2):368–376. doi: 10.1016/0304-4165(70)90036-x. [DOI] [PubMed] [Google Scholar]
- Terleckyj B., Willett N. P., Shockman G. D. Growth of several cariogenic strains of oral streptococci in a chemically defined medium. Infect Immun. 1975 Apr;11(4):649–655. doi: 10.1128/iai.11.4.649-655.1975. [DOI] [PMC free article] [PubMed] [Google Scholar]