Abstract
The traditional function of neurotransmitter-gated ion channels is to induce rapid changes in electrical activity. Channels that are Ca(2+)-permeable, such as N-methyl-D-aspartate receptors at depolarized membrane potentials, can have a broader repertoire of consequences, including changes in synaptic efficacy, developmental plasticity, and excitotoxicity. Neuronal nicotinic receptors for acetylcholine (nAChRs) are usually less Ca(2+)-permeable than N-methyl-D-aspartate receptors but have a significant Ca2+ permeability, which is greater at negative potentials. Here we report that in neuroendocrine cells, activation of nAChRs can trigger exocytosis at hyperpolarized potentials. We used whole-cell patch-clamp recordings to record currents and the capacitance detection technique to monitor exocytosis in isolated bovine chromaffin cells. Stimulation of nAChRs at hyperpolarized potentials (-60 or -90 mV) evokes a large current and a maximal capacitance increase corresponding to the fusion of approximately 200 large dense-core vesicles. The amount of exocytosis is controlled both by the Ca2+ influx through nAChRs and by a contribution from thapsigargin-sensitive Ca2+ sequestering stores. This is a form of neurotransmitter action in which activation of nAChRs triggers secretion through an additional coupling pathway that coexists with classical voltage-dependent Ca2+ entry.
Full text
PDF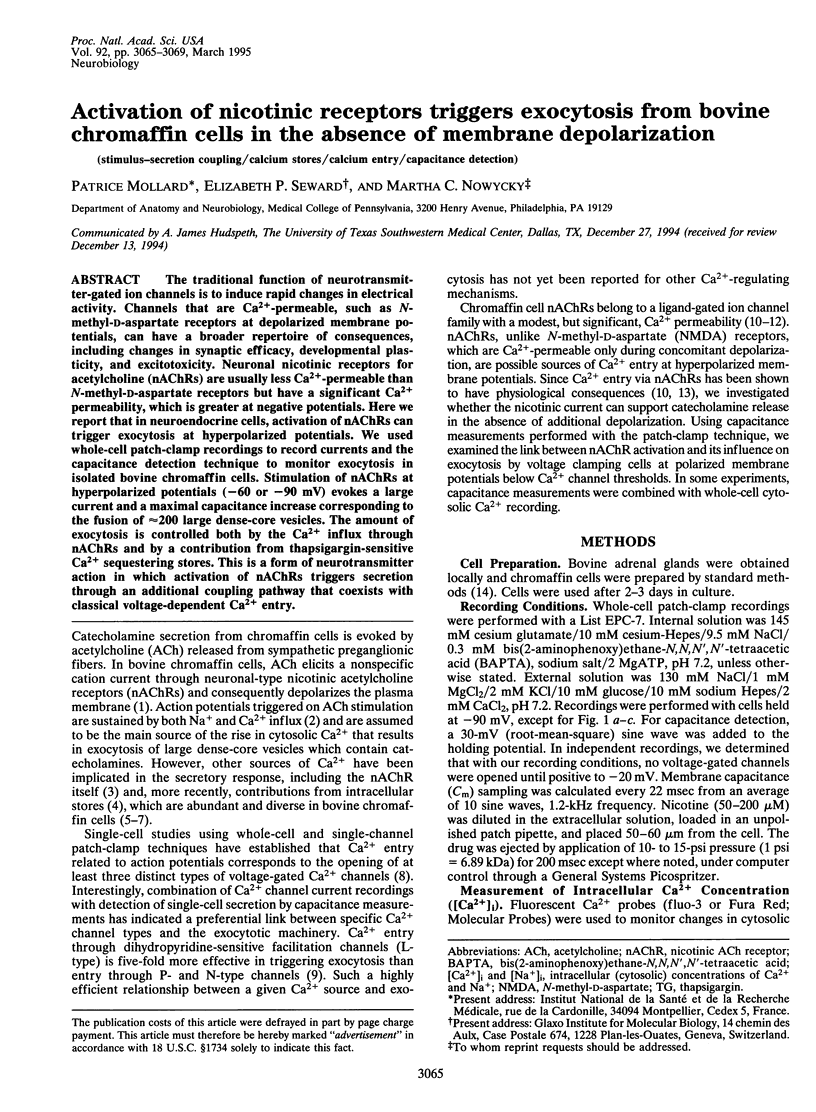
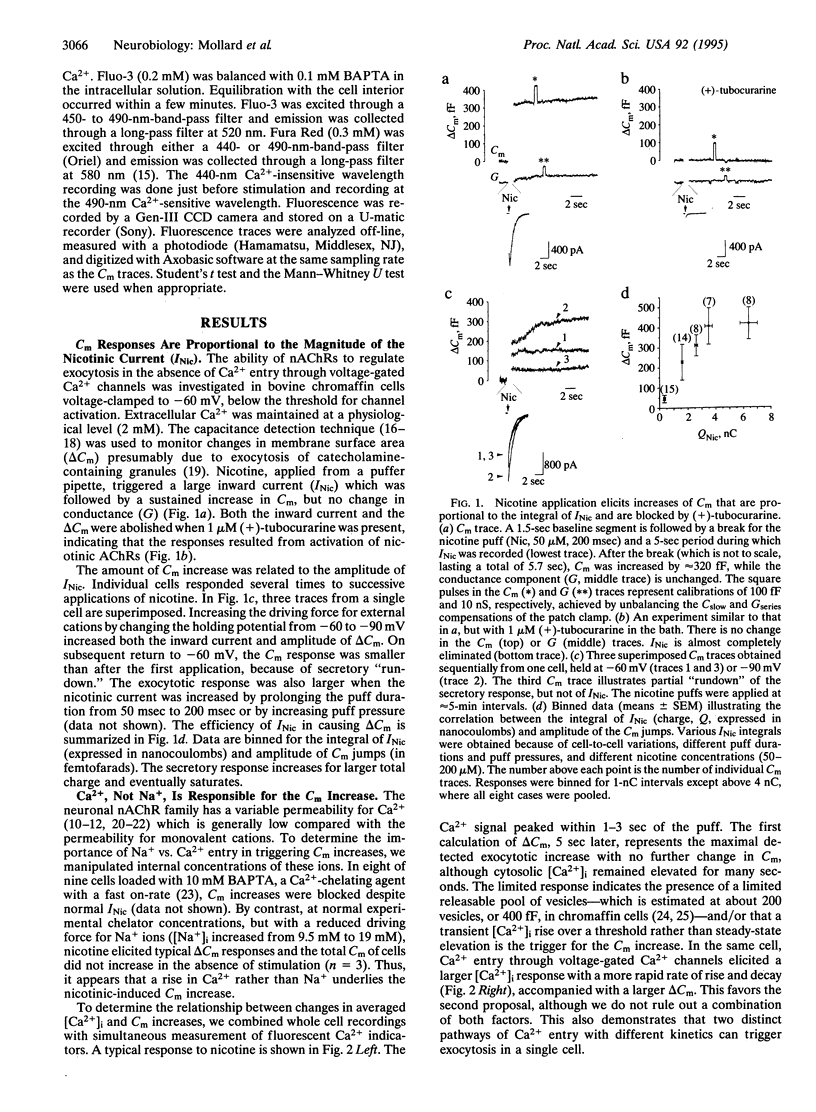
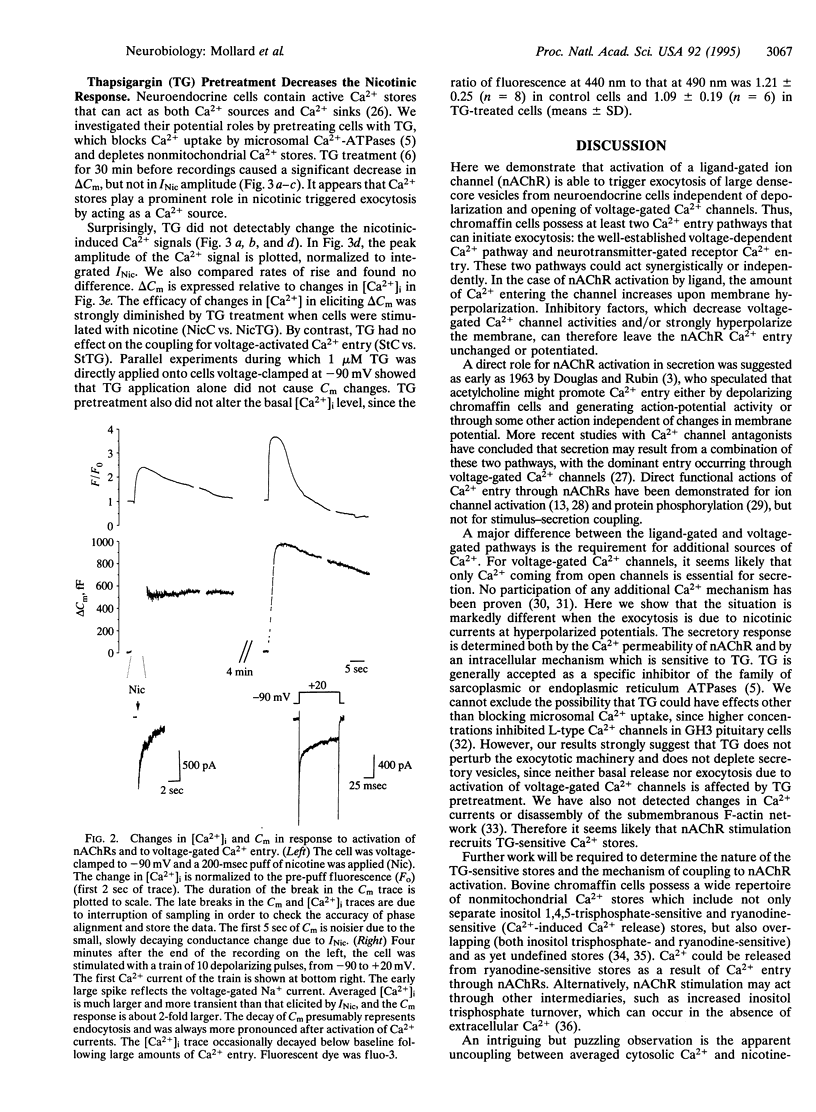
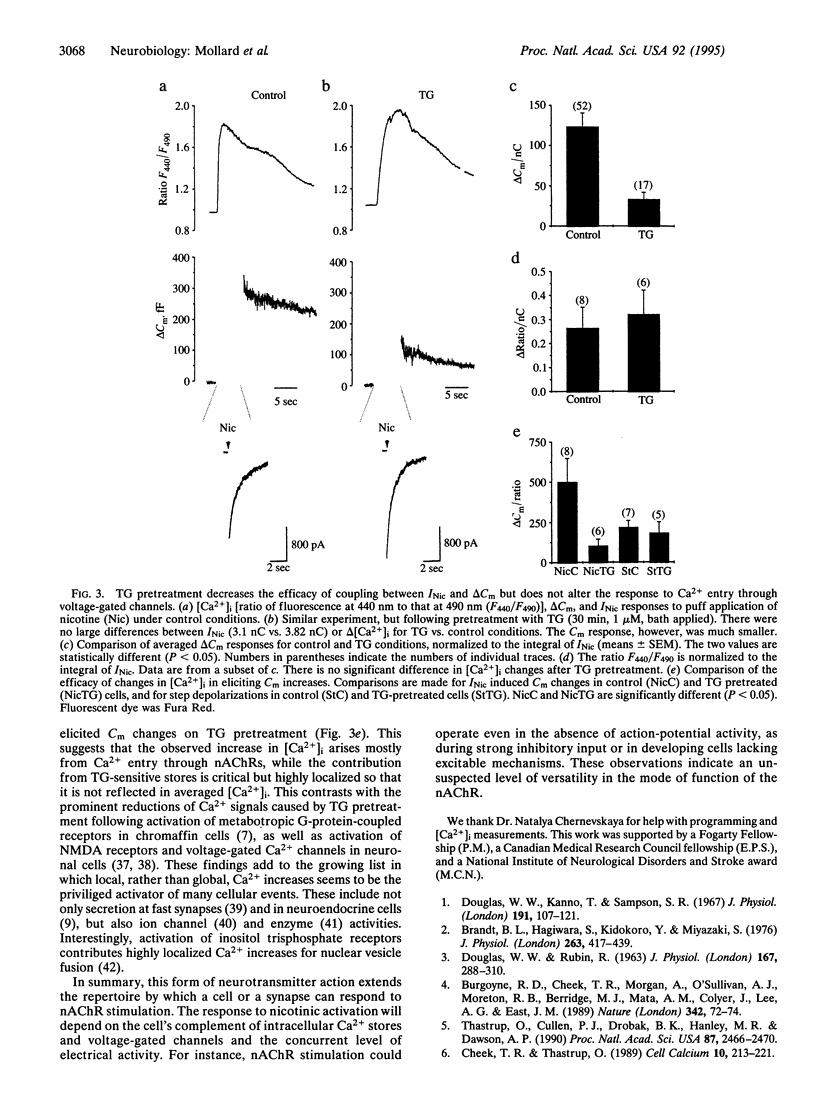
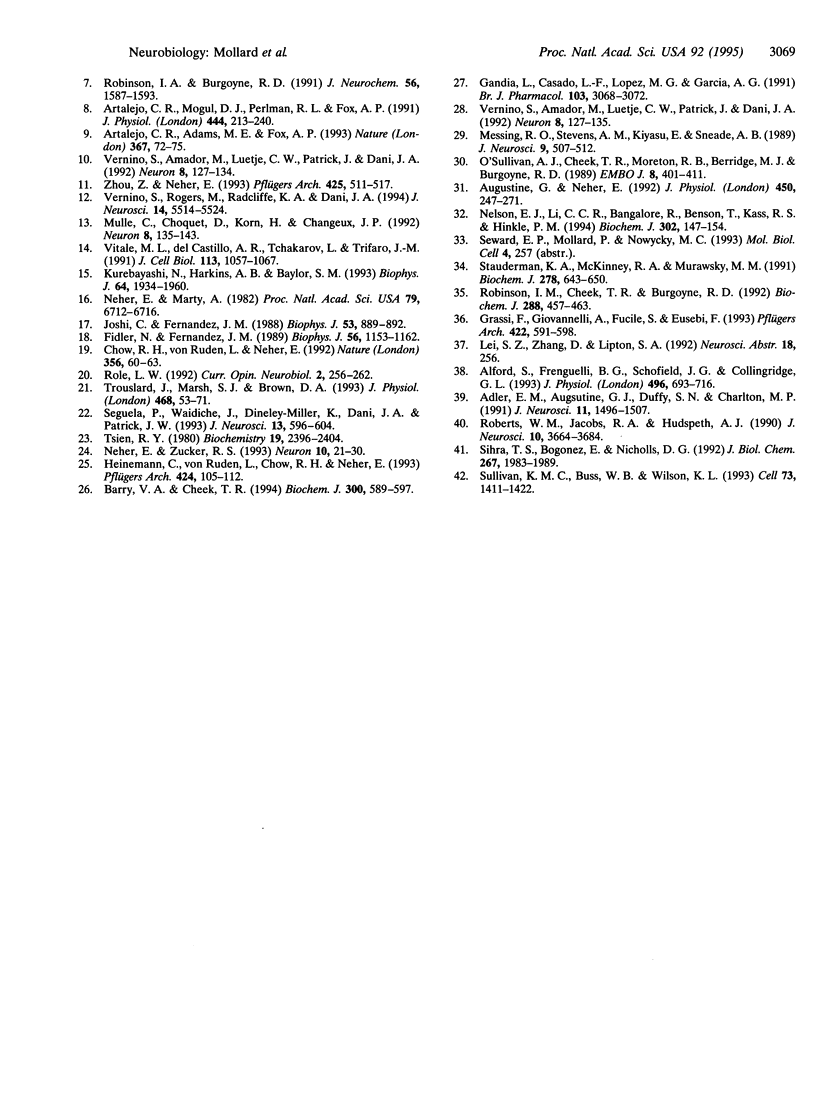
Selected References
These references are in PubMed. This may not be the complete list of references from this article.
- Adler E. M., Augustine G. J., Duffy S. N., Charlton M. P. Alien intracellular calcium chelators attenuate neurotransmitter release at the squid giant synapse. J Neurosci. 1991 Jun;11(6):1496–1507. doi: 10.1523/JNEUROSCI.11-06-01496.1991. [DOI] [PMC free article] [PubMed] [Google Scholar]
- Alford S., Frenguelli B. G., Schofield J. G., Collingridge G. L. Characterization of Ca2+ signals induced in hippocampal CA1 neurones by the synaptic activation of NMDA receptors. J Physiol. 1993 Sep;469:693–716. doi: 10.1113/jphysiol.1993.sp019838. [DOI] [PMC free article] [PubMed] [Google Scholar]
- Artalejo C. R., Mogul D. J., Perlman R. L., Fox A. P. Three types of bovine chromaffin cell Ca2+ channels: facilitation increases the opening probability of a 27 pS channel. J Physiol. 1991 Dec;444:213–240. doi: 10.1113/jphysiol.1991.sp018874. [DOI] [PMC free article] [PubMed] [Google Scholar]
- Augustine G. J., Neher E. Calcium requirements for secretion in bovine chromaffin cells. J Physiol. 1992 May;450:247–271. doi: 10.1113/jphysiol.1992.sp019126. [DOI] [PMC free article] [PubMed] [Google Scholar]
- Barry V. A., Cheek T. R. A caffeine- and ryanodine-sensitive intracellular Ca2+ store can act as a Ca2+ source and a Ca2+ sink in PC12 cells. Biochem J. 1994 Jun 1;300(Pt 2):589–597. doi: 10.1042/bj3000589. [DOI] [PMC free article] [PubMed] [Google Scholar]
- Brandt B. L., Hagiwara S., Kidokoro Y., Miyazaki S. Action potentials in the rat chromaffin cell and effects of acetylcholine. J Physiol. 1976 Dec;263(3):417–439. doi: 10.1113/jphysiol.1976.sp011638. [DOI] [PMC free article] [PubMed] [Google Scholar]
- Burgoyne R. D., Cheek T. R., Morgan A., O'Sullivan A. J., Moreton R. B., Berridge M. J., Mata A. M., Colyer J., Lee A. G., East J. M. Distribution of two distinct Ca2+-ATPase-like proteins and their relationships to the agonist-sensitive calcium store in adrenal chromaffin cells. Nature. 1989 Nov 2;342(6245):72–74. doi: 10.1038/342072a0. [DOI] [PubMed] [Google Scholar]
- Cheek T. R., Thastrup O. Internal Ca2+ mobilization and secretion in bovine adrenal chromaffin cells. Cell Calcium. 1989 May-Jun;10(4):213–221. doi: 10.1016/0143-4160(89)90004-3. [DOI] [PubMed] [Google Scholar]
- Chow R. H., von Rüden L., Neher E. Delay in vesicle fusion revealed by electrochemical monitoring of single secretory events in adrenal chromaffin cells. Nature. 1992 Mar 5;356(6364):60–63. doi: 10.1038/356060a0. [DOI] [PubMed] [Google Scholar]
- Douglas W. W., Kanno T., Sampson S. R. Influence of the ionic environment on the membrane potential of adrenal chromaffin cells and on the depolarizing effect of acetylcholine. J Physiol. 1967 Jul;191(1):107–121. doi: 10.1113/jphysiol.1967.sp008239. [DOI] [PMC free article] [PubMed] [Google Scholar]
- Douglas W. W., Rubin R. P. The mechanism of catecholamine release from the adrenal medulla and the role of calcium in stimulus-secretion coupling. J Physiol. 1963 Jul;167(2):288–310. doi: 10.1113/jphysiol.1963.sp007150. [DOI] [PMC free article] [PubMed] [Google Scholar]
- Fidler N., Fernandez J. M. Phase tracking: an improved phase detection technique for cell membrane capacitance measurements. Biophys J. 1989 Dec;56(6):1153–1162. doi: 10.1016/S0006-3495(89)82762-6. [DOI] [PMC free article] [PubMed] [Google Scholar]
- Grassi F., Giovannelli A., Fucile S., Eusebi F. Activation of the nicotinic acetylcholine receptor mobilizes calcium from caffeine-insensitive stores in C2C12 mouse myotubes. Pflugers Arch. 1993 Mar;422(6):591–598. doi: 10.1007/BF00374007. [DOI] [PubMed] [Google Scholar]
- Heinemann C., von Rüden L., Chow R. H., Neher E. A two-step model of secretion control in neuroendocrine cells. Pflugers Arch. 1993 Jul;424(2):105–112. doi: 10.1007/BF00374600. [DOI] [PubMed] [Google Scholar]
- Joshi C., Fernandez J. M. Capacitance measurements. An analysis of the phase detector technique used to study exocytosis and endocytosis. Biophys J. 1988 Jun;53(6):885–892. doi: 10.1016/S0006-3495(88)83169-2. [DOI] [PMC free article] [PubMed] [Google Scholar]
- Kurebayashi N., Harkins A. B., Baylor S. M. Use of fura red as an intracellular calcium indicator in frog skeletal muscle fibers. Biophys J. 1993 Jun;64(6):1934–1960. doi: 10.1016/S0006-3495(93)81564-9. [DOI] [PMC free article] [PubMed] [Google Scholar]
- Messing R. O., Stevens A. M., Kiyasu E., Sneade A. B. Nicotinic and muscarinic agonists stimulate rapid protein kinase C translocation in PC12 cells. J Neurosci. 1989 Feb;9(2):507–512. doi: 10.1523/JNEUROSCI.09-02-00507.1989. [DOI] [PMC free article] [PubMed] [Google Scholar]
- Mulle C., Choquet D., Korn H., Changeux J. P. Calcium influx through nicotinic receptor in rat central neurons: its relevance to cellular regulation. Neuron. 1992 Jan;8(1):135–143. doi: 10.1016/0896-6273(92)90115-t. [DOI] [PubMed] [Google Scholar]
- Neher E., Marty A. Discrete changes of cell membrane capacitance observed under conditions of enhanced secretion in bovine adrenal chromaffin cells. Proc Natl Acad Sci U S A. 1982 Nov;79(21):6712–6716. doi: 10.1073/pnas.79.21.6712. [DOI] [PMC free article] [PubMed] [Google Scholar]
- Nelson E. J., Li C. C., Bangalore R., Benson T., Kass R. S., Hinkle P. M. Inhibition of L-type calcium-channel activity by thapsigargin and 2,5-t-butylhydroquinone, but not by cyclopiazonic acid. Biochem J. 1994 Aug 15;302(Pt 1):147–154. doi: 10.1042/bj3020147. [DOI] [PMC free article] [PubMed] [Google Scholar]
- Roberts W. M., Jacobs R. A., Hudspeth A. J. Colocalization of ion channels involved in frequency selectivity and synaptic transmission at presynaptic active zones of hair cells. J Neurosci. 1990 Nov;10(11):3664–3684. doi: 10.1523/JNEUROSCI.10-11-03664.1990. [DOI] [PMC free article] [PubMed] [Google Scholar]
- Robinson I. M., Burgoyne R. D. Characterisation of distinct inositol 1,4,5-trisphosphate-sensitive and caffeine-sensitive calcium stores in digitonin-permeabilised adrenal chromaffin cells. J Neurochem. 1991 May;56(5):1587–1593. doi: 10.1111/j.1471-4159.1991.tb02055.x. [DOI] [PubMed] [Google Scholar]
- Robinson I. M., Cheek T. R., Burgoyne R. D. Ca2+ influx induced by the Ca(2+)-ATPase inhibitors 2,5-di-(t-butyl)-1,4-benzohydroquinone and thapsigargin in bovine adrenal chromaffin cells. Biochem J. 1992 Dec 1;288(Pt 2):457–463. doi: 10.1042/bj2880457. [DOI] [PMC free article] [PubMed] [Google Scholar]
- Sihra T. S., Bogonez E., Nicholls D. G. Localized Ca2+ entry preferentially effects protein dephosphorylation, phosphorylation, and glutamate release. J Biol Chem. 1992 Jan 25;267(3):1983–1989. [PubMed] [Google Scholar]
- Stauderman K. A., McKinney R. A., Murawsky M. M. The role of caffeine-sensitive Ca2+ stores in agonist- and inositol 1,4,5-trisphosphate-induced Ca2+ release from bovine adrenal chromaffin cells. Biochem J. 1991 Sep 15;278(Pt 3):643–650. doi: 10.1042/bj2780643. [DOI] [PMC free article] [PubMed] [Google Scholar]
- Sullivan K. M., Busa W. B., Wilson K. L. Calcium mobilization is required for nuclear vesicle fusion in vitro: implications for membrane traffic and IP3 receptor function. Cell. 1993 Jul 2;73(7):1411–1422. doi: 10.1016/0092-8674(93)90366-x. [DOI] [PubMed] [Google Scholar]
- Thastrup O., Cullen P. J., Drøbak B. K., Hanley M. R., Dawson A. P. Thapsigargin, a tumor promoter, discharges intracellular Ca2+ stores by specific inhibition of the endoplasmic reticulum Ca2(+)-ATPase. Proc Natl Acad Sci U S A. 1990 Apr;87(7):2466–2470. doi: 10.1073/pnas.87.7.2466. [DOI] [PMC free article] [PubMed] [Google Scholar]
- Trouslard J., Marsh S. J., Brown D. A. Calcium entry through nicotinic receptor channels and calcium channels in cultured rat superior cervical ganglion cells. J Physiol. 1993 Aug;468:53–71. doi: 10.1113/jphysiol.1993.sp019759. [DOI] [PMC free article] [PubMed] [Google Scholar]
- Vernino S., Amador M., Luetje C. W., Patrick J., Dani J. A. Calcium modulation and high calcium permeability of neuronal nicotinic acetylcholine receptors. Neuron. 1992 Jan;8(1):127–134. doi: 10.1016/0896-6273(92)90114-s. [DOI] [PubMed] [Google Scholar]
- Vernino S., Amador M., Luetje C. W., Patrick J., Dani J. A. Calcium modulation and high calcium permeability of neuronal nicotinic acetylcholine receptors. Neuron. 1992 Jan;8(1):127–134. doi: 10.1016/0896-6273(92)90114-s. [DOI] [PubMed] [Google Scholar]
- Vernino S., Rogers M., Radcliffe K. A., Dani J. A. Quantitative measurement of calcium flux through muscle and neuronal nicotinic acetylcholine receptors. J Neurosci. 1994 Sep;14(9):5514–5524. doi: 10.1523/JNEUROSCI.14-09-05514.1994. [DOI] [PMC free article] [PubMed] [Google Scholar]
- Vitale M. L., Rodríguez Del Castillo A., Tchakarov L., Trifaró J. M. Cortical filamentous actin disassembly and scinderin redistribution during chromaffin cell stimulation precede exocytosis, a phenomenon not exhibited by gelsolin. J Cell Biol. 1991 Jun;113(5):1057–1067. doi: 10.1083/jcb.113.5.1057. [DOI] [PMC free article] [PubMed] [Google Scholar]