Abstract
To investigate factors regulating ammonia (NH3) production by isolated defined proximal tubule segments, we examined the rates of total NH3 (NH3 + NH+4) production by individual proximal tubule segments perfused in vitro under a variety of perfusion conditions. Segments consisting of late convoluted and early straight portions of superficial proximal tubules were incubated at 37 degrees C in Krebs-Ringer bicarbonate (KRB) buffer containing 0.5 mM L-glutamine and 1.0 mM sodium acetate, pH 7.4. The rate of total ammonia production was calculated from the rate of accumulation of total NH3 in the bath. The total ammonia production rate by unperfused proximal segments was 6.0 +/- 0.2 (+/- SE) pmol/mm per minute, which was significantly lower than segments perfused at a flow rate of 22.7 +/- 3.4 nl/min with KRB buffer (21.5 +/- 1.4 pmol/mm per minute; P less than 0.001) or with KRB buffer containing 0.5 mM L-glutamine (31.9 +/- 2.5; P less than 0.001). The rate of NH3 production was higher in segments perfused with glutamine than in segments perfused without glutamine (P less than 0.01). The perfusion-associated stimulation of NH3 production was characterized further. Analysis of collected luminal fluid samples revealed that the luminal fluid total NH3 leaving the distal end of the perfused proximal segment accounted for 91% of the increment in NH3 production observed with perfusion. Increasing the perfusion flow rate from 3.7 +/- 0.1 to 22.7 +/- 3.4 nl/min by raising the perfusion pressure resulted in an increased rate of total NH3 production in the presence or absence of perfusate glutamine (mean rise in rate of total NH3 production was 14.9 +/- 3.7 pmol/mm per minute in segments perfused with glutamine and 7.8 +/- 0.9 in those perfused without glutamine). In addition, increasing the perfusion flow rate at a constant perfusion pressure increased the rate of luminal output of NH3. Total NH3 production was not affected by reducing perfusate sodium concentration to 25 mM and adding 1.0 mM amiloride to the perfusate, a condition that was shown to inhibit proximal tubule fluid reabsorption. These observations demonstrate that the rate of total NH3 production by the mouse proximal tubule is accelerated by perfusion of the lumen of the segment, by the presence of glutamine in the perfusate, and by increased perfusion flow rates. The increased rate of NH3 production with perfusion seems not to depend upon normal rates of sodium reabsorption. The mechanism underlying the stimulation of NH3 production by luminal flow is unknown and requires further study.
Full text
PDF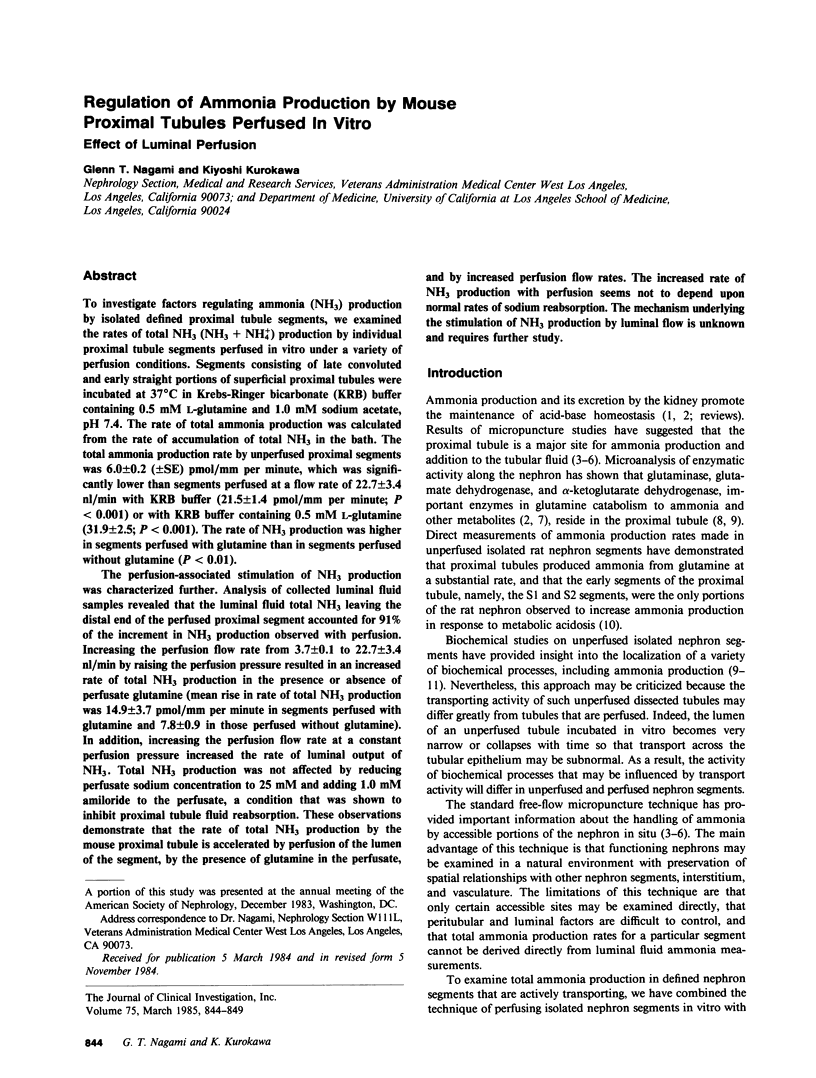
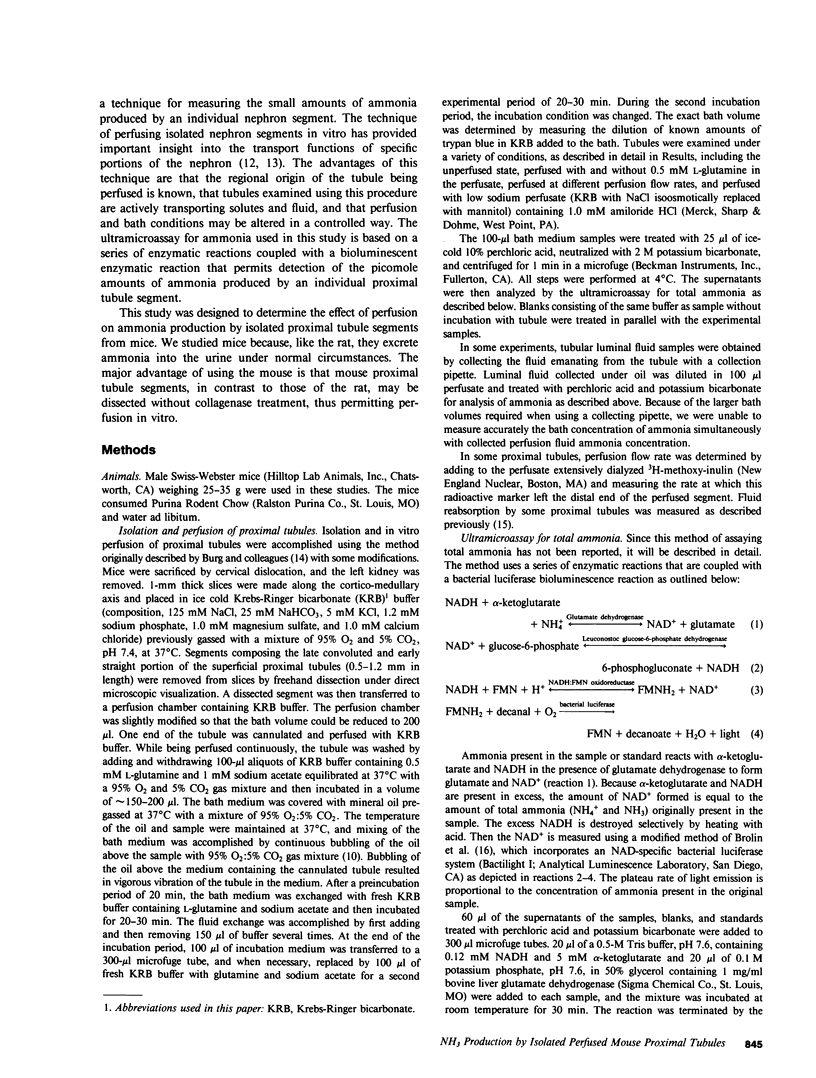
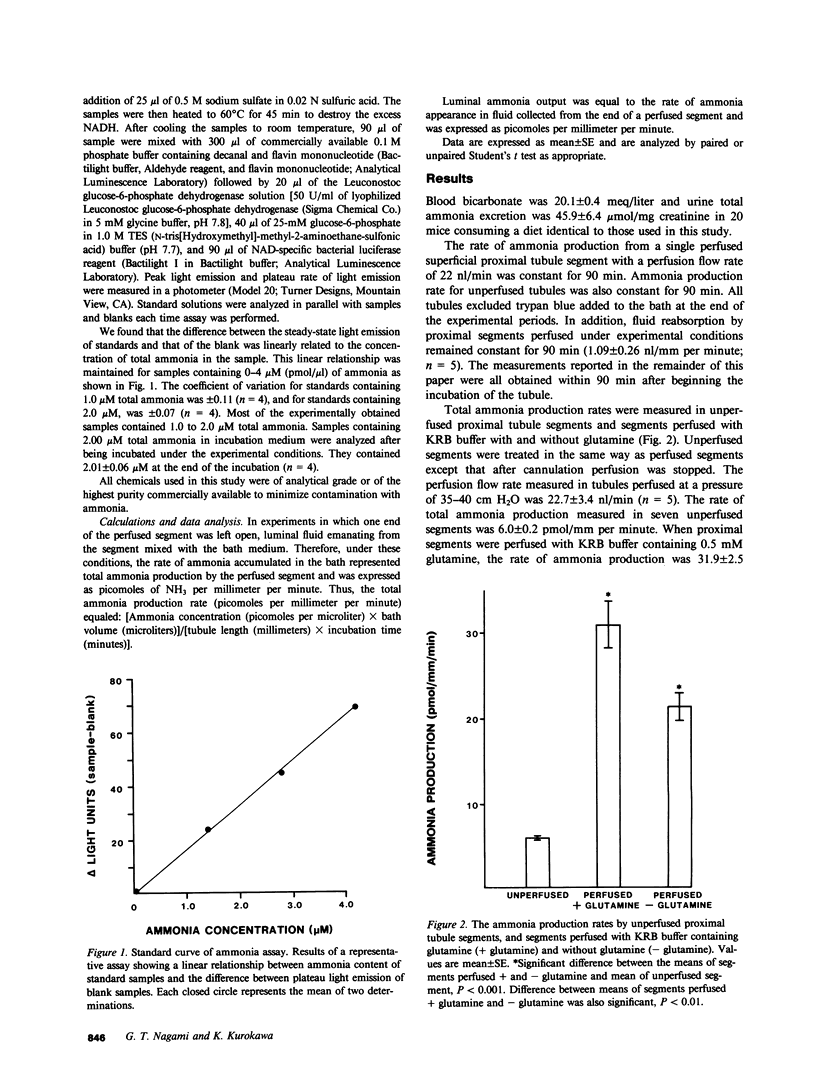
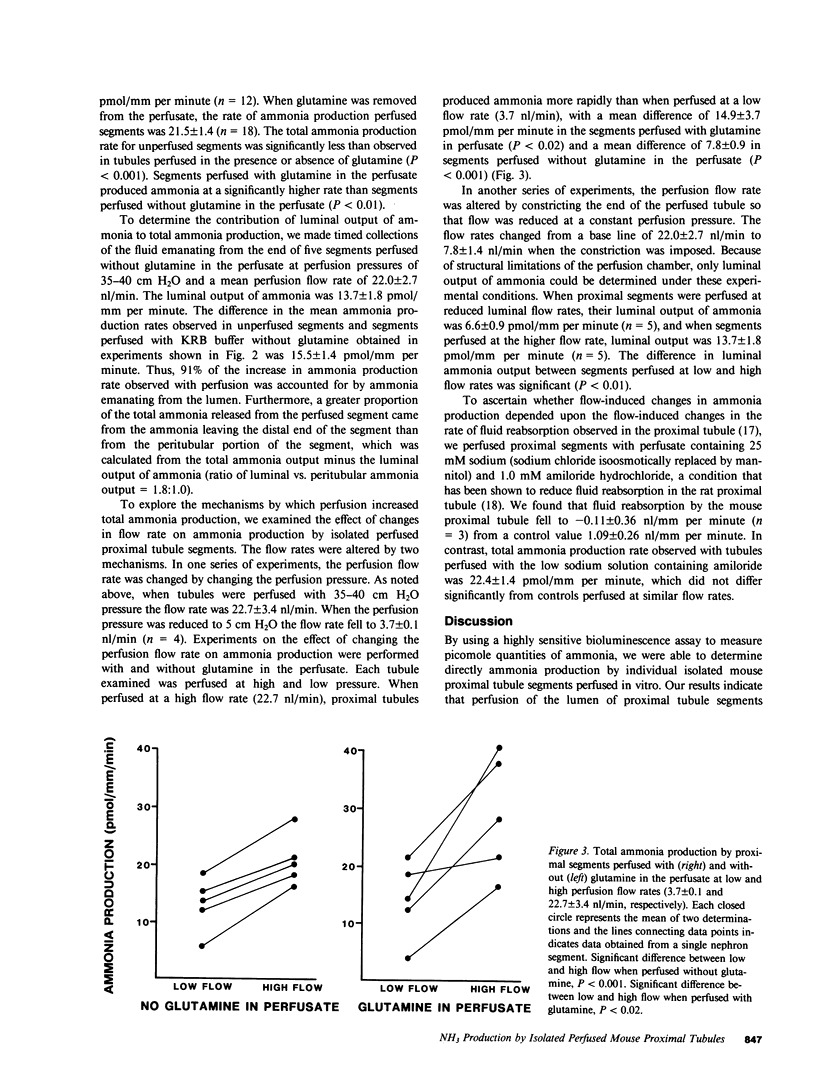
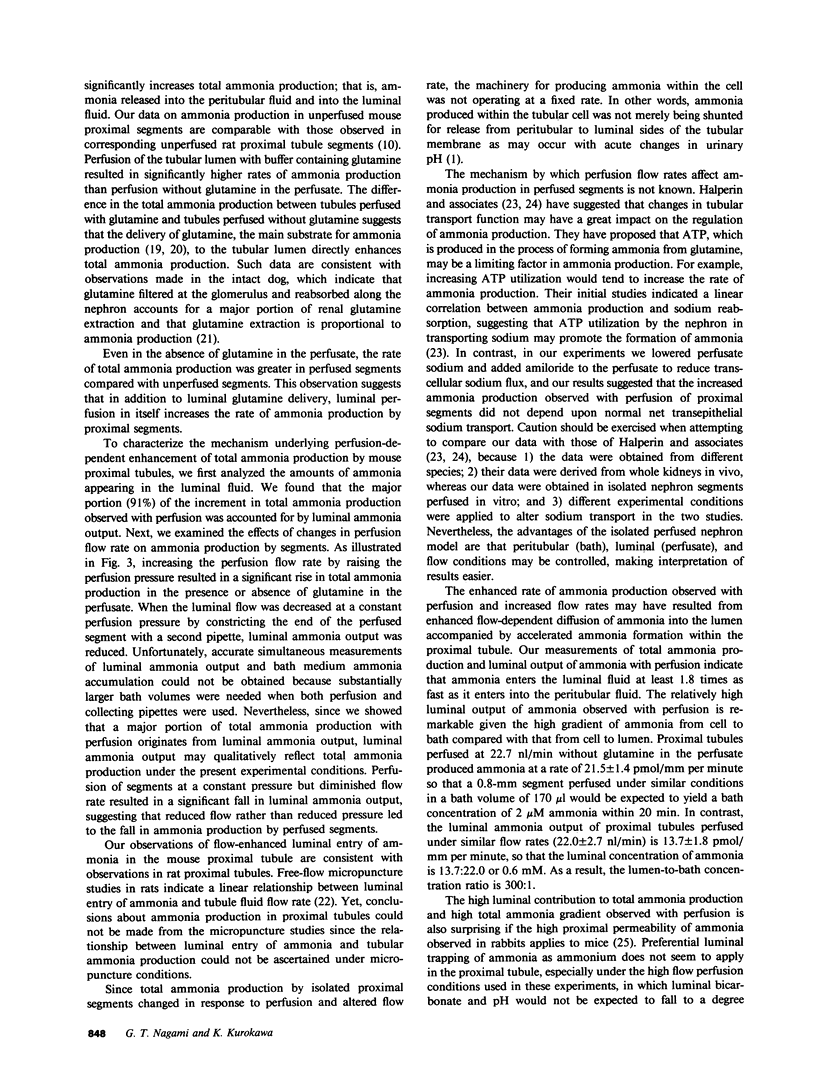
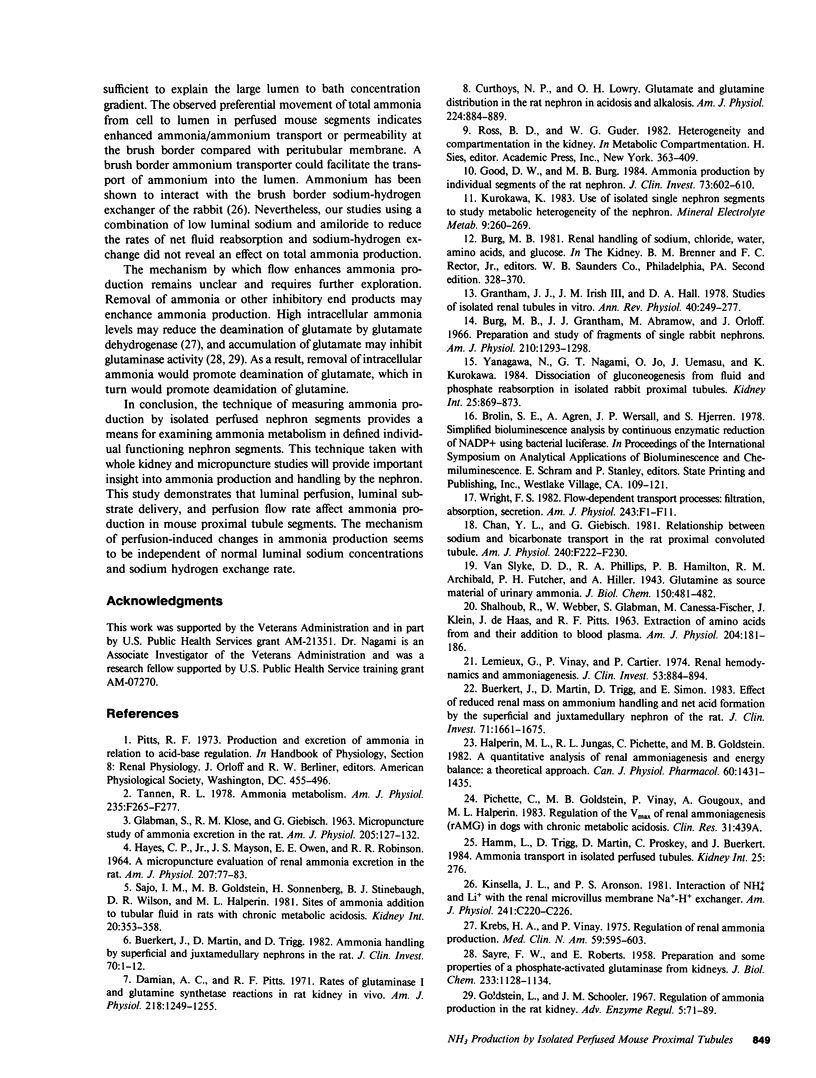
Selected References
These references are in PubMed. This may not be the complete list of references from this article.
- Buerkert J., Martin D., Trigg D. Ammonium handling by superficial and juxtamedullary nephrons in the rat. Evidence for an ammonia shunt between the loop of Henle and the collecting duct. J Clin Invest. 1982 Jul;70(1):1–12. doi: 10.1172/JCI110581. [DOI] [PMC free article] [PubMed] [Google Scholar]
- Buerkert J., Martin D., Trigg D., Simon E. Effect of reduced renal mass on ammonium handling and net acid formation by the superficial and juxtamedullary nephron of the rat. Evidence for impaired reentrapment rather than decreased production of ammonium in the acidosis of uremia. J Clin Invest. 1983 Jun;71(6):1661–1675. doi: 10.1172/JCI110921. [DOI] [PMC free article] [PubMed] [Google Scholar]
- Burg M., Grantham J., Abramow M., Orloff J. Preparation and study of fragments of single rabbit nephrons. Am J Physiol. 1966 Jun;210(6):1293–1298. doi: 10.1152/ajplegacy.1966.210.6.1293. [DOI] [PubMed] [Google Scholar]
- Chan Y. L., Giebisch G. Relationship between sodium and bicarbonate transport in the rat proximal convoluted tubule. Am J Physiol. 1981 Mar;240(3):F222–F230. doi: 10.1152/ajprenal.1981.240.3.F222. [DOI] [PubMed] [Google Scholar]
- Curthoys N. P., Lowry O. H. Glutamate and glutamine distribution in the rat nephron in acidosis and alkalosis. Am J Physiol. 1973 Apr;224(4):884–889. doi: 10.1152/ajplegacy.1973.224.4.884. [DOI] [PubMed] [Google Scholar]
- Damian A. C., Pitts R. F. Rates of glutaminase I and glutamine synthetase reactions in rat kidney in vivo. Am J Physiol. 1970 May;218(5):1249–1255. doi: 10.1152/ajplegacy.1970.218.5.1249. [DOI] [PubMed] [Google Scholar]
- GLABMAN S., KOSE R. M., GIEBISCH G. Micropuncture study of ammonia excretion in the rat. Am J Physiol. 1963 Jul;205:127–132. doi: 10.1152/ajplegacy.1963.205.1.127. [DOI] [PubMed] [Google Scholar]
- Good D. W., Burg M. B. Ammonia production by individual segments of the rat nephron. J Clin Invest. 1984 Mar;73(3):602–610. doi: 10.1172/JCI111250. [DOI] [PMC free article] [PubMed] [Google Scholar]
- Grantham J. J., Irish J. M., 3rd, Hall D. A. Studies of isolated renal tubules in vitro. Annu Rev Physiol. 1978;40:249–277. doi: 10.1146/annurev.ph.40.030178.001341. [DOI] [PubMed] [Google Scholar]
- HAYES C. P., Jr, MAYSON J. S., OWEN E. E., ROBINSON R. R. A MICROPUNCTURE EVALUATION OF RENAL AMMONIA EXCRETION IN THE RAT. Am J Physiol. 1964 Jul;207:77–83. doi: 10.1152/ajplegacy.1964.207.1.77. [DOI] [PubMed] [Google Scholar]
- Halperin M. L., Jungas R. L., Pichette C., Goldstein M. B. A quantitative analysis of renal ammoniagenesis and energy balance: a theoretical approach. Can J Physiol Pharmacol. 1982 Dec;60(12):1431–1435. doi: 10.1139/y82-212. [DOI] [PubMed] [Google Scholar]
- Kinsella J. L., Aronson P. S. Interaction of NH4+ and Li+ with the renal microvillus membrane Na+-H+ exchanger. Am J Physiol. 1981 Nov;241(5):C220–C226. doi: 10.1152/ajpcell.1981.241.5.C220. [DOI] [PubMed] [Google Scholar]
- Krebs H. A., Vinay P. Regulation of renal ammonia production. Med Clin North Am. 1975 May;59(3):595–603. doi: 10.1016/s0025-7125(16)32011-9. [DOI] [PubMed] [Google Scholar]
- Kurokawa K. Use of isolated single nephron segments to study metabolic heterogeneity of the nephron. Miner Electrolyte Metab. 1983;9(4-6):260–269. [PubMed] [Google Scholar]
- Lemieux G., Vinay P., Cartier P. Renal hemodynamics and ammoniagenesis. Characteristics of the antiluminal site for glutamine extraction. J Clin Invest. 1974 Mar;53(3):884–894. doi: 10.1172/JCI107629. [DOI] [PMC free article] [PubMed] [Google Scholar]
- SAYRE F. W., ROBERTS E. Preparation and some properties of a phosphateactivated glutaminase from kidneys. J Biol Chem. 1958 Nov;233(5):1128–1134. [PubMed] [Google Scholar]
- Sajo I. M., Goldstein M. B., Sonnenberg H., Stinebaugh B. J., Wilson D. R., Halperin M. L. Sites of ammonia addition to tubular fluid in rats with chronic metabolic acidosis. Kidney Int. 1981 Sep;20(3):353–358. doi: 10.1038/ki.1981.146. [DOI] [PubMed] [Google Scholar]
- Tannen R. L. Ammonia metabolism. Am J Physiol. 1978 Oct;235(4):F265–F277. doi: 10.1152/ajprenal.1978.235.4.F265. [DOI] [PubMed] [Google Scholar]
- Yanagawa N., Nagami G. T., Jo O., Uemasu J., Kurokawa K. Dissociation of gluconeogenesis from fluid and phosphate reabsorption in isolated rabbit proximal tubules. Kidney Int. 1984 Jun;25(6):869–873. doi: 10.1038/ki.1984.103. [DOI] [PubMed] [Google Scholar]