Abstract
Erythrocytes from patients with homozygous hemoglobin C disease (CC cells) contain less K, Na, and water than do erythrocytes from normal subjects that contain only hemoglobin A (AA cells). In this paper, we provide evidence that the reduced K content and volume of CC cells are due to the activity in these but not in AA cells of a K transport system that is: (a) insensitive to ouabain and bumetanide, and (b) stimulated by increased cell volume, and dependent on internal pH (pHi). When the cation and water content of CC cells was increased (by making the membrane temporarily permeable to cations with nystatin) and the cells were then incubated in an isotonic medium containing 140 mM NaCl and 4 mM KCl, they lost K and shrunk back toward the original volume. This regulatory K and volume decrease was not inhibited by ouabain or bumetanide. When CC cells were incubated in a hypotonic medium, with ouabain and bumetanide, they also lost K and shrunk toward the original volume. This behavior was not observed in control AA cells. The ouabain- and bumetanide-resistant K efflux from CC cells was volume and pH dependent: K efflux from CC cells rose from 5-6 to 20-25 mmol/liter of cells X h, when cell volume was increased by increasing cell solute content (nystatin method) or by exposure to hypotonic media. In CC cells, the dependence of K efflux on pHo had a bell shape, with a maximal flux (20-25 mmol/liter of cells X h) at pHo 6.8-7.0. In contrast, the K efflux from control cells was minimal at pH 7.4 (1.2 mmol/liter of cells X h) and was slightly stimulated by both acid and alkaline pH. In order to study the effect of pHi and pHo on K efflux, CC cells were incubated with 4,4'-diisothiocyanostilbene-2,2'-disulfonic acid (150 microM) and acetazolamide (1 mM) at different pHi (6.7, 7.3, and 7.8), and resuspended in media with different pHo (6.75, 7.4, and 8): K efflux was stimulated by reducing pHi but was independent of pHo. The ouabain- and bumetanide-resistant K efflux from CC cells was not inhibited by some inhibitors of the Ca2+-activated K permeability. It seems likely that the genetically determined change in the primary structure of hemoglobin C directly or indirectly causes this modification in K transport. One possible mechanism could involve an electrostatic interaction between C hemoglobin and components of the erythrocyte membrane.
Full text
PDF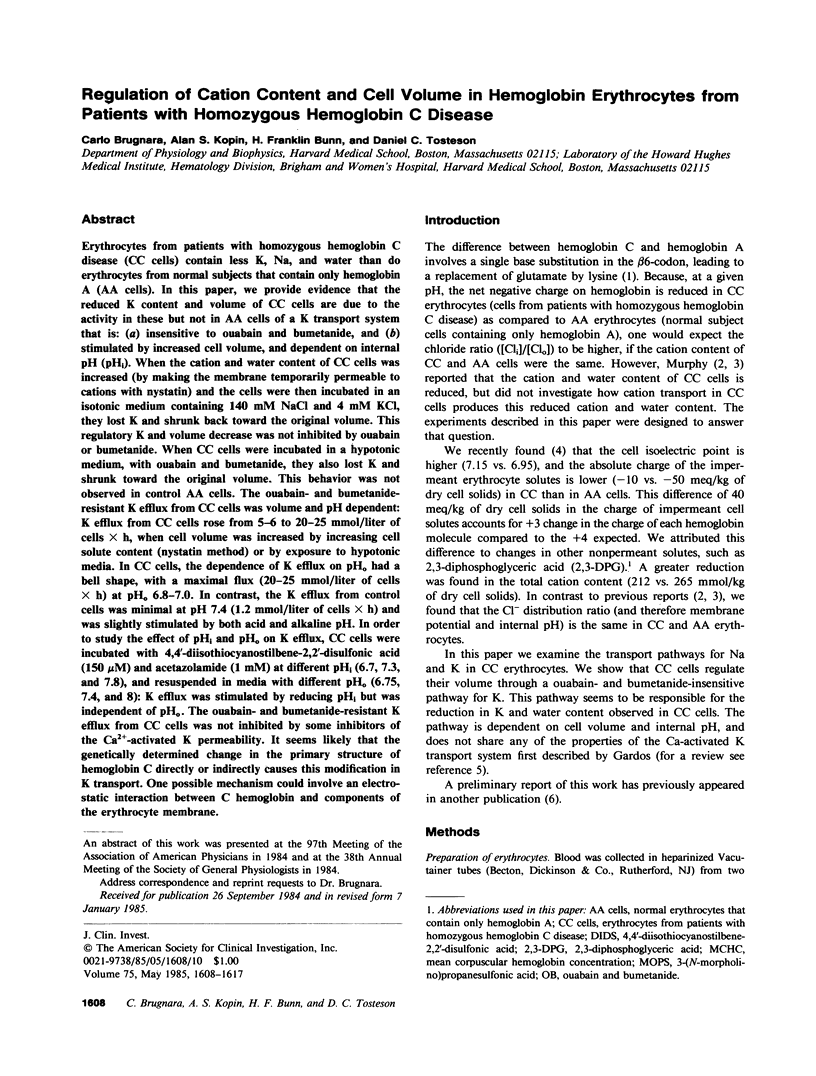
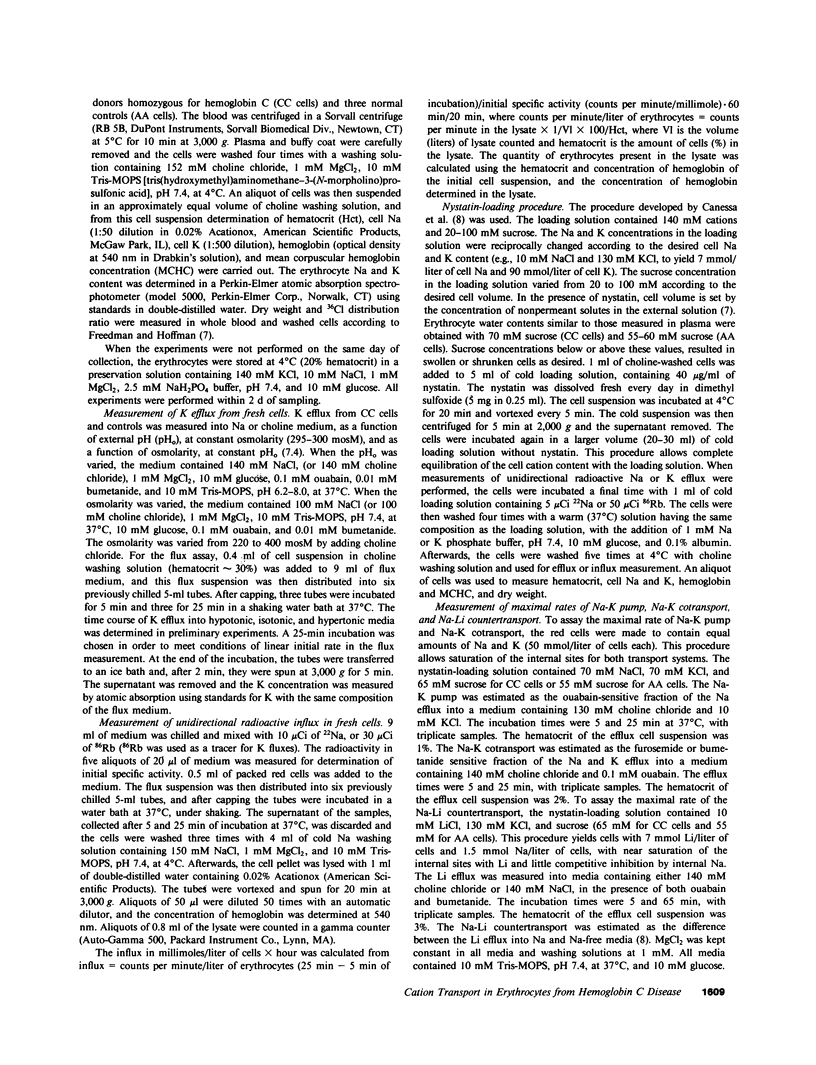
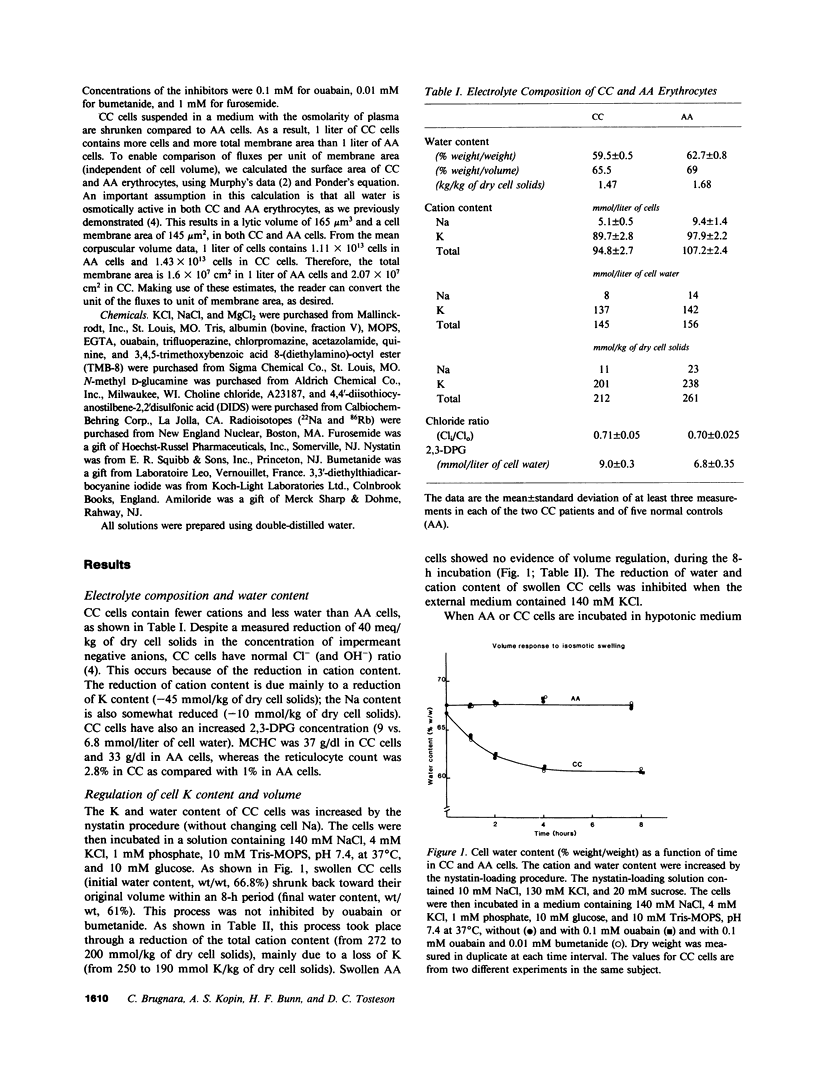
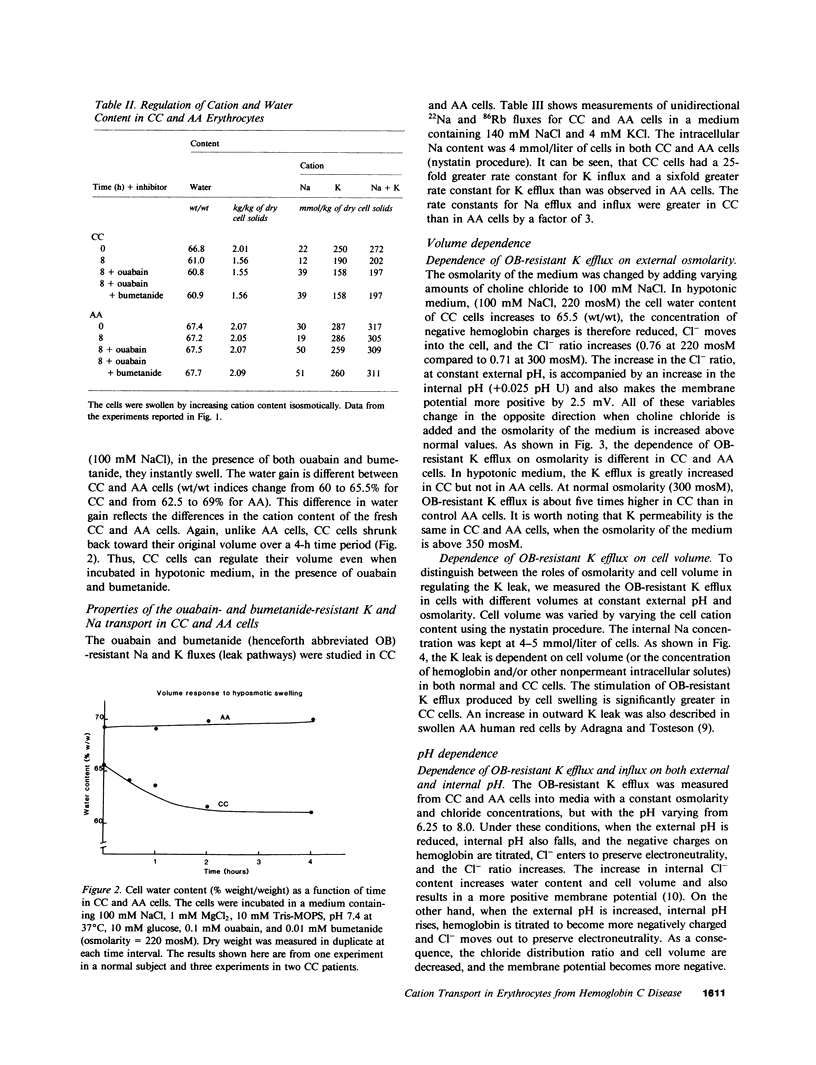
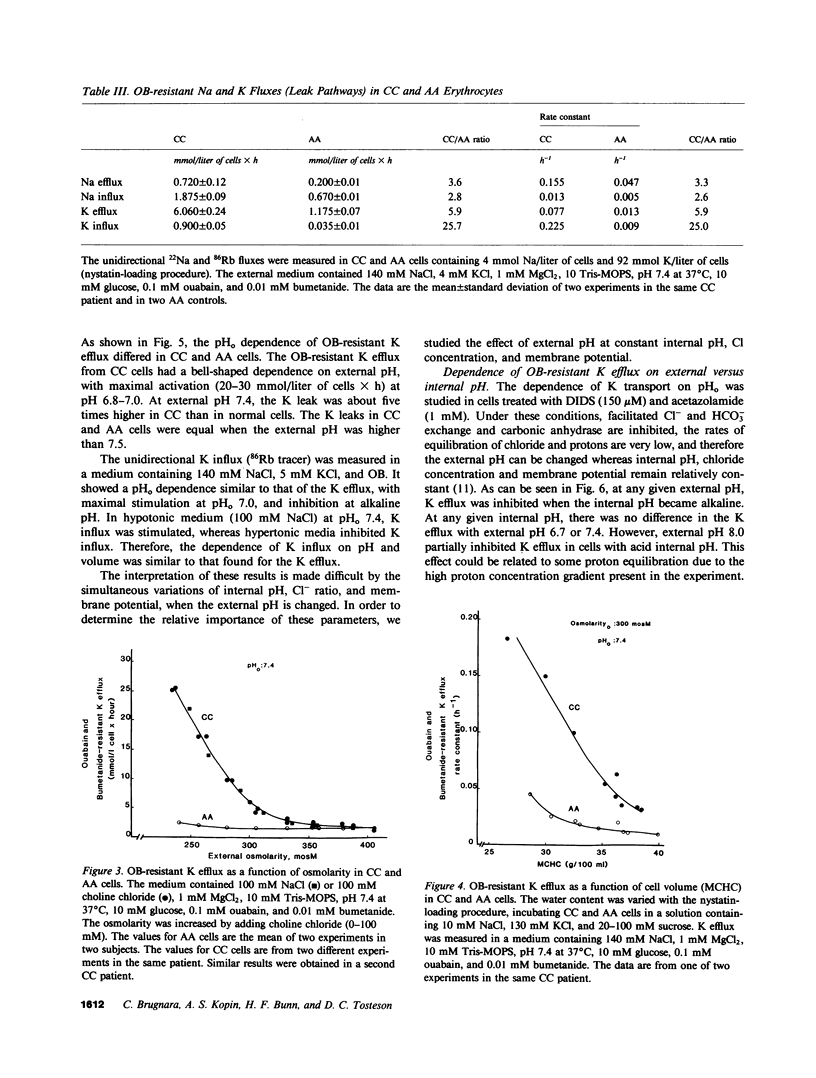
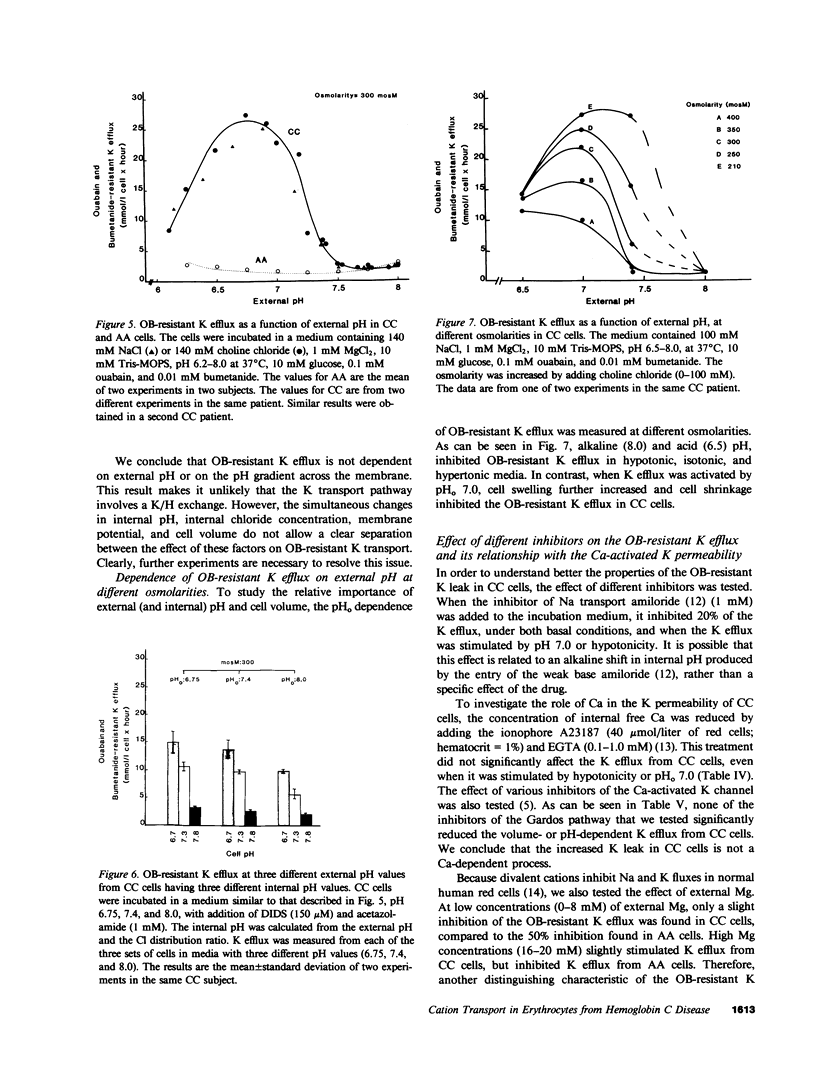
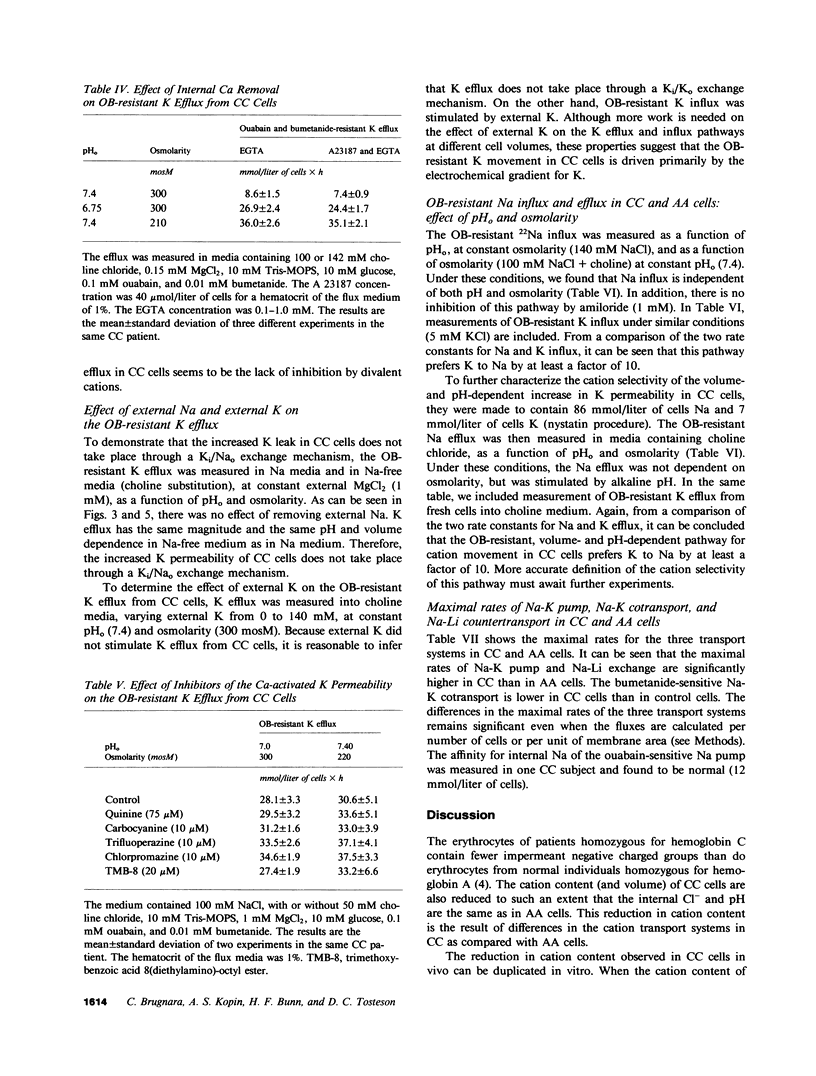
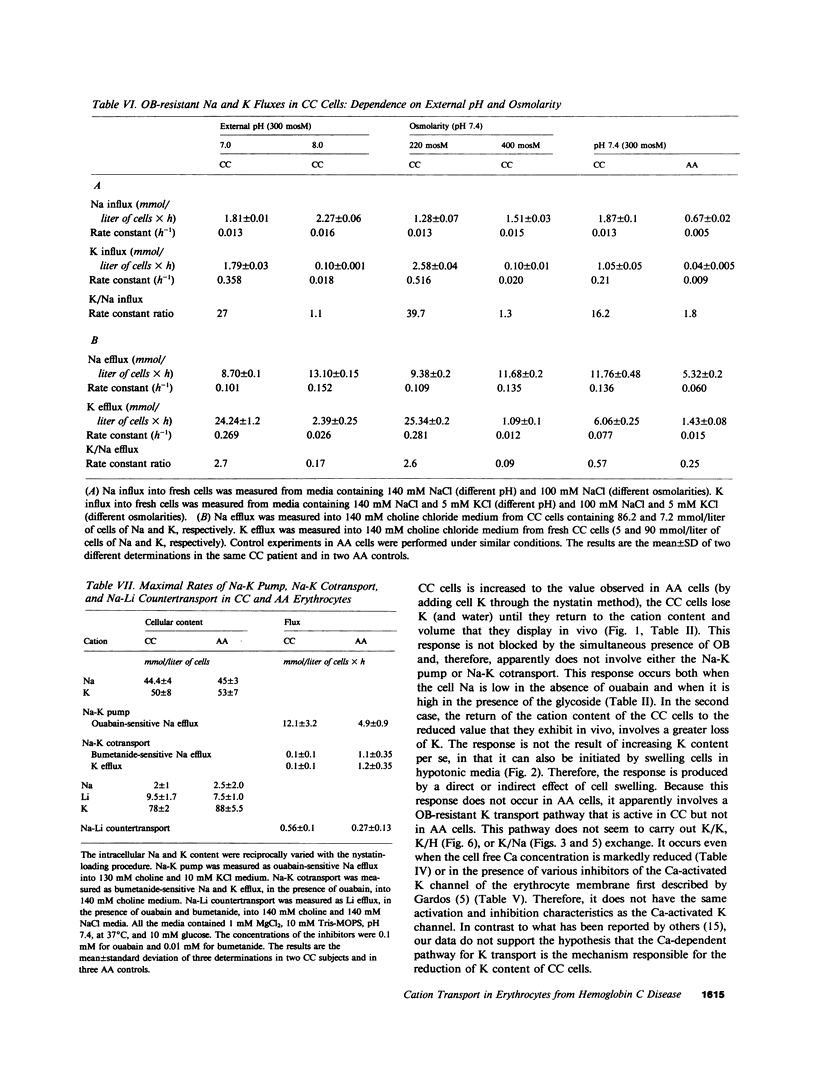
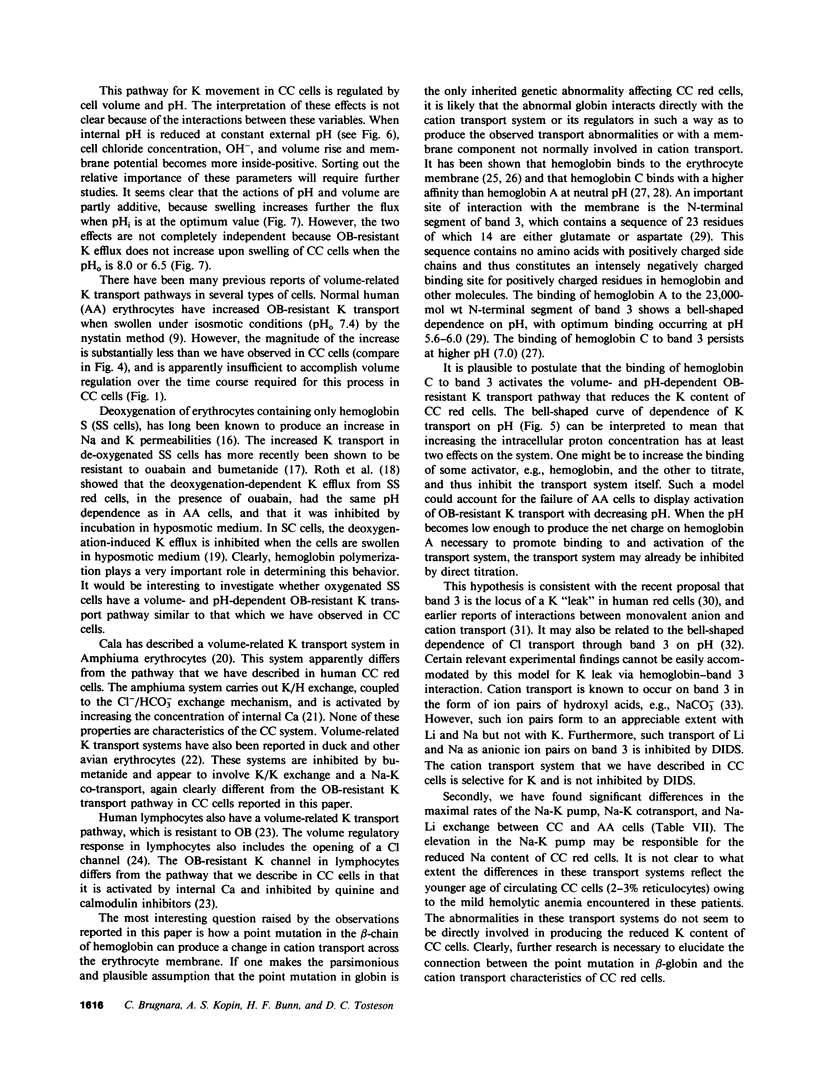
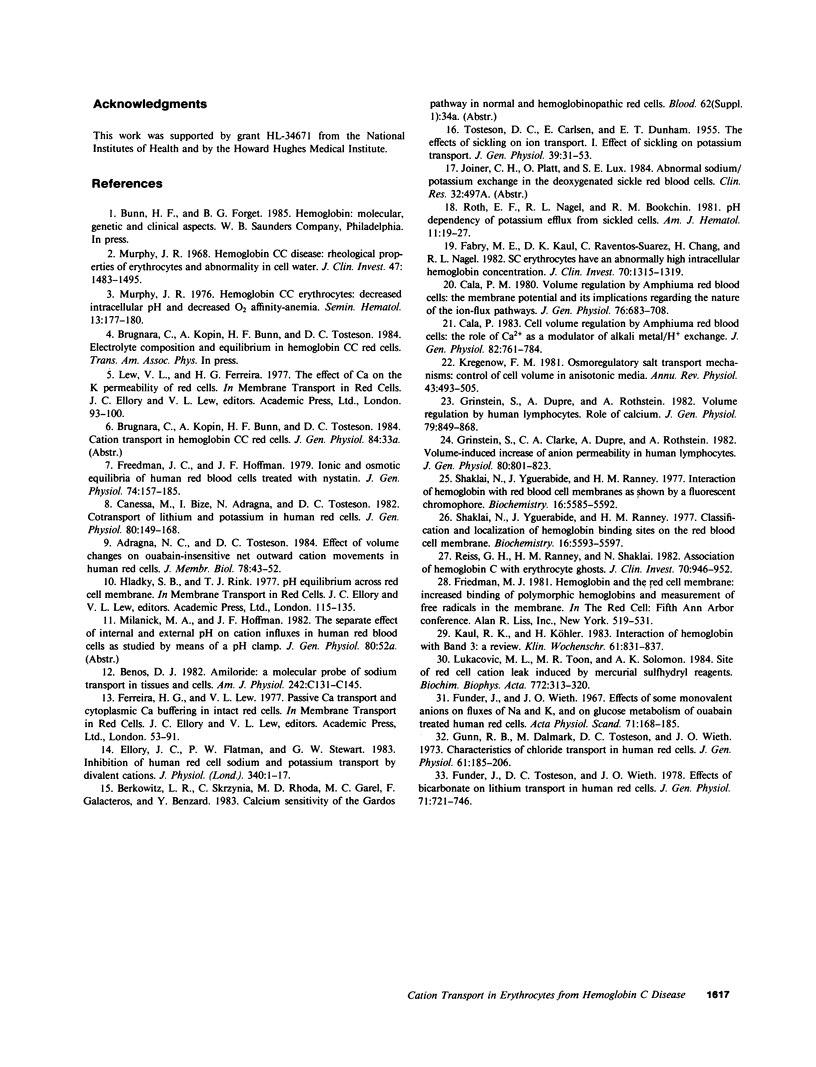
Images in this article
Selected References
These references are in PubMed. This may not be the complete list of references from this article.
- Adragna N. C., Tosteson D. C. Effect of volume changes on ouabain-insensitive net outward cation movements in human red cells. J Membr Biol. 1984;78(1):43–52. doi: 10.1007/BF01872531. [DOI] [PubMed] [Google Scholar]
- Cala P. M. Cell volume regulation by Amphiuma red blood cells. The role of Ca+2 as a modulator of alkali metal/H+ exchange. J Gen Physiol. 1983 Dec;82(6):761–784. doi: 10.1085/jgp.82.6.761. [DOI] [PMC free article] [PubMed] [Google Scholar]
- Cala P. M. Volume regulation by Amphiuma red blood cells. The membrane potential and its implications regarding the nature of the ion-flux pathways. J Gen Physiol. 1980 Dec;76(6):683–708. doi: 10.1085/jgp.76.6.683. [DOI] [PMC free article] [PubMed] [Google Scholar]
- Canessa M., Bize I., Adragna N., Tosteson D. Cotransport of lithium and potassium in human red cells. J Gen Physiol. 1982 Jul;80(1):149–168. doi: 10.1085/jgp.80.1.149. [DOI] [PMC free article] [PubMed] [Google Scholar]
- Ellory J. C., Flatman P. W., Stewart G. W. Inhibition of human red cell sodium and potassium transport by divalent cations. J Physiol. 1983 Jul;340:1–17. doi: 10.1113/jphysiol.1983.sp014746. [DOI] [PMC free article] [PubMed] [Google Scholar]
- Fabry M. E., Kaul D. K., Raventos-Suarez C., Chang H., Nagel R. L. SC erythrocytes have an abnormally high intracellular hemoglobin concentration. Pathophysiological consequences. J Clin Invest. 1982 Dec;70(6):1315–1319. doi: 10.1172/JCI110732. [DOI] [PMC free article] [PubMed] [Google Scholar]
- Freedman J. C., Hoffman J. F. Ionic and osmotic equilibria of human red blood cells treated with nystatin. J Gen Physiol. 1979 Aug;74(2):157–185. doi: 10.1085/jgp.74.2.157. [DOI] [PMC free article] [PubMed] [Google Scholar]
- Funder J., Tosteson D. C., Wieth J. O. Effects of bicarbonate on lithium transport in human red cells. J Gen Physiol. 1978 Jun;71(6):721–746. doi: 10.1085/jgp.71.6.721. [DOI] [PMC free article] [PubMed] [Google Scholar]
- Funder J., Wieth J. O. Effects of some monovalent anions on fluxes of Na and K, and on glucose metabolism of ouabain treated human red cells. Acta Physiol Scand. 1967 Oct-Nov;71(2):168–185. doi: 10.1111/j.1748-1716.1967.tb03723.x. [DOI] [PubMed] [Google Scholar]
- Grinstein S., Clarke C. A., Dupre A., Rothstein A. Volume-induced increase of anion permeability in human lymphocytes. J Gen Physiol. 1982 Dec;80(6):801–823. doi: 10.1085/jgp.80.6.801. [DOI] [PMC free article] [PubMed] [Google Scholar]
- Grinstein S., Dupre A., Rothstein A. Volume regulation by human lymphocytes. Role of calcium. J Gen Physiol. 1982 May;79(5):849–868. doi: 10.1085/jgp.79.5.849. [DOI] [PMC free article] [PubMed] [Google Scholar]
- Gunn R. B., Dalmark M., Tosteson D. C., Wieth J. O. Characteristics of chloride transport in human red blood cells. J Gen Physiol. 1973 Feb;61(2):185–206. doi: 10.1085/jgp.61.2.185. [DOI] [PMC free article] [PubMed] [Google Scholar]
- Kaul R. K., Köhler H. Interaction of hemoglobin with band 3: a review. Klin Wochenschr. 1983 Sep 1;61(17):831–837. doi: 10.1007/BF01537457. [DOI] [PubMed] [Google Scholar]
- Lukacovic M. F., Toon M. R., Solomon A. K. Site of red cell cation leak induced by mercurial sulfhydryl reagents. Biochim Biophys Acta. 1984 May 30;772(3):313–320. doi: 10.1016/0005-2736(84)90148-2. [DOI] [PubMed] [Google Scholar]
- Murphy J. R. Hemoglobin CC disease: rheological properties or erythrocytes and abnormalities in cell water. J Clin Invest. 1968 Jul;47(7):1483–1495. doi: 10.1172/JCI105842. [DOI] [PMC free article] [PubMed] [Google Scholar]
- Murphy J. R. Hemoglobin CC erythrocytes: decreased intracellular pH and decreased O2 affinity--anemia. Semin Hematol. 1976 Jul;13(3):177–180. [PubMed] [Google Scholar]
- Reiss G. H., Ranney H. M., Shaklai N. Association of hemoglobin C with erythrocyte ghosts. J Clin Invest. 1982 Nov;70(5):946–952. doi: 10.1172/JCI110706. [DOI] [PMC free article] [PubMed] [Google Scholar]
- Roth E. F., Jr, Nagel R. L., Bookchin R. M. pH dependency of potassium efflux from sickled red cells. Am J Hematol. 1981;11(1):19–27. doi: 10.1002/ajh.2830110104. [DOI] [PubMed] [Google Scholar]
- Shaklai N., Yguerabide J., Ranney H. M. Classification and localization of hemoglobin binding sites on the red blood cell membrane. Biochemistry. 1977 Dec 13;16(25):5593–5597. doi: 10.1021/bi00644a032. [DOI] [PubMed] [Google Scholar]
- Shaklai N., Yguerabide J., Ranney H. M. Interaction of hemoglobin with red blood cell membranes as shown by a fluorescent chromophore. Biochemistry. 1977 Dec 13;16(25):5585–5592. doi: 10.1021/bi00644a031. [DOI] [PubMed] [Google Scholar]
- TOSTESON D. C., CARLSEN E., DUNHAM E. T. The effects of sickling on ion transport. I. Effect of sickling on potassium transport. J Gen Physiol. 1955 Sep 20;39(1):31–53. doi: 10.1085/jgp.39.1.31. [DOI] [PMC free article] [PubMed] [Google Scholar]