Abstract
Structural and conformational requirements for an electric field-dependent transition between conducting and nonconducting macromolecular systems are: two kinetically interconvertible and energetically similar conformations, one conducting and the other nonconducting, which have axes spanning the lipid layer of biological membranes, but which have different net dipole moments along those axes. Two examples are described. A previously defined helix, the π6LD-helix now termed the β63,3-helix, is proposed as the conducting species, and the linear peptide correlate of the cyclic hexapeptide conformation containing two β-turns and an inversion element of symmetry is proposed as a nonconducting species. The latter is termed an anti-β62-spiral and contains little or no net dipole moment per turn, whereas the β63,3-helix contains a net dipole moment along the helix axis of about 0.5 Debye per dipeptide unit. A related conducting and nonconducting pair with large net dipole moments of opposite sign, termed syn-β62-spiral and β62,4-helix, are also described. The spiral conformations are stabilized in a lipid layer by intermolecular hydrogen bonds, leading to a linear association of transmembrane structures. A conformational transition in one member of the array could lead to destabilization of an adjacent member of the array. The conformational analysis uses a concept of cyclic conformations with linear conformational correlates. The anti-β62-spiral and β63,3-helix are derivable from the conformations of the cyclic structure [unk], whereas the syn-β2-spiral and β62,4-helix may be derived from the cyclic structure [unk].
The conformational analysis leads to the expectation that N-formyl-(L-Ala-L-Ala-Gly)n would form conducting channels.
Keywords: biological membranes, gramicidin A, dipole moments, electric field
Full text
PDF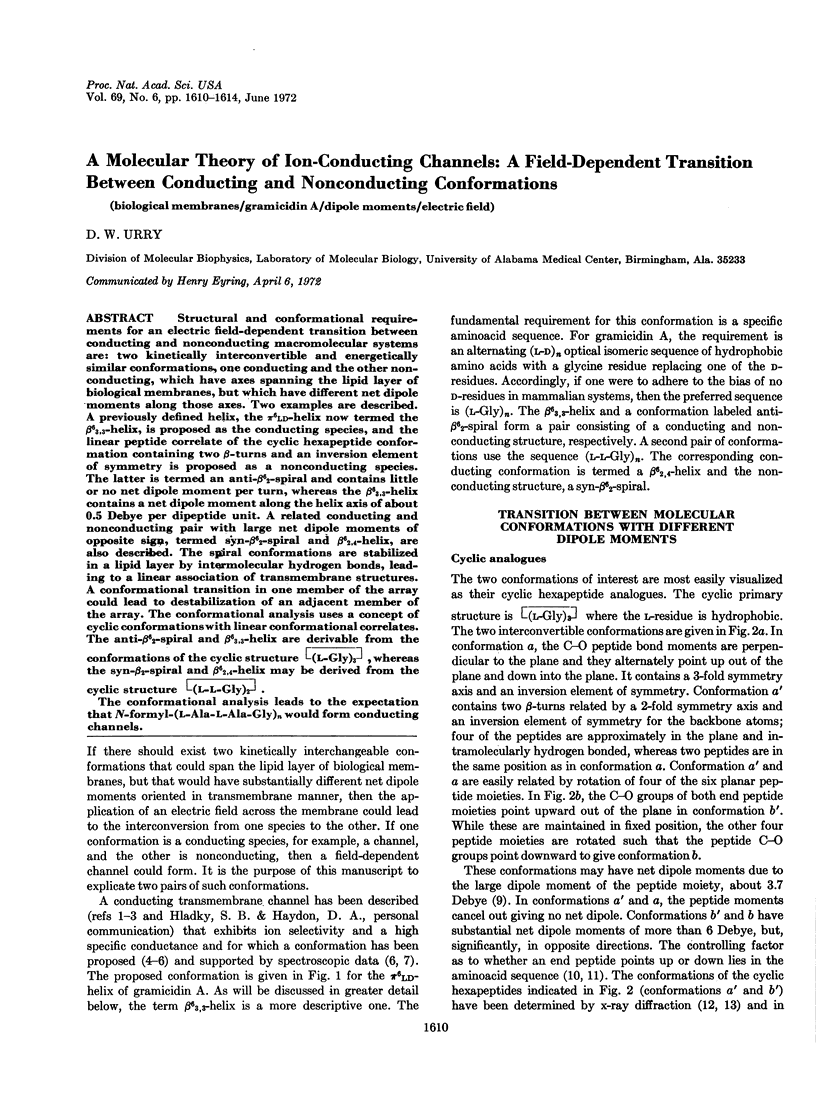
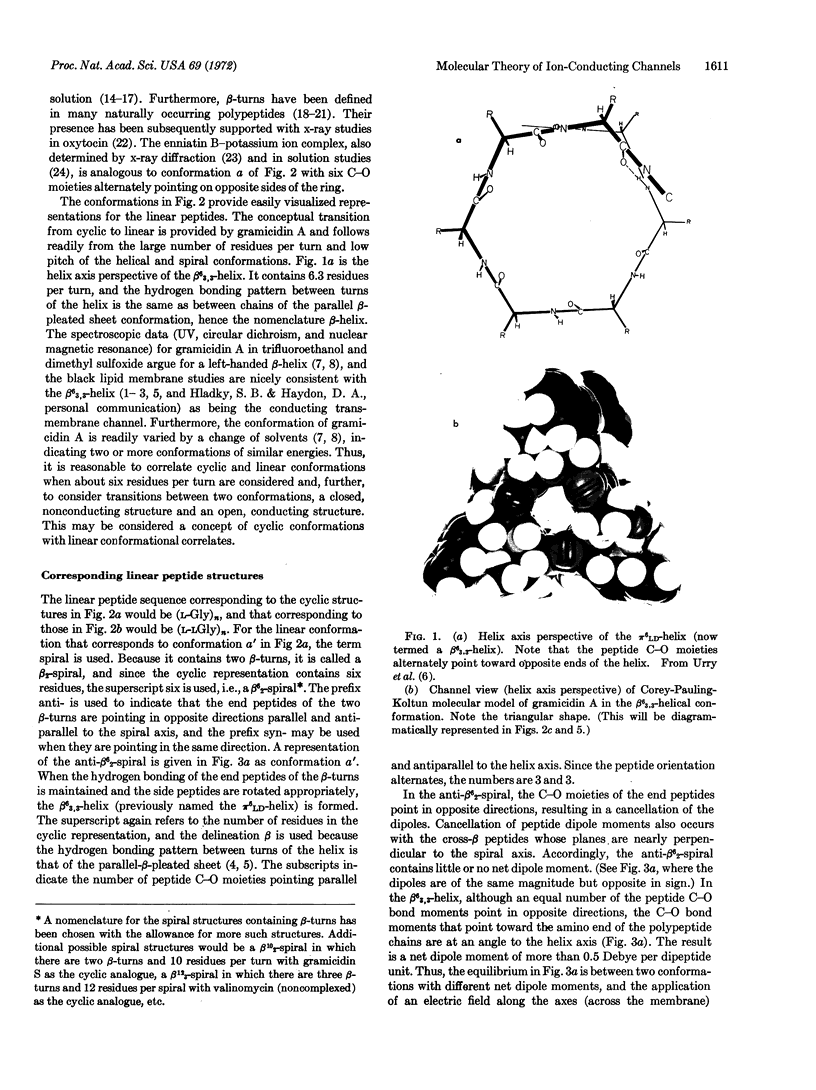
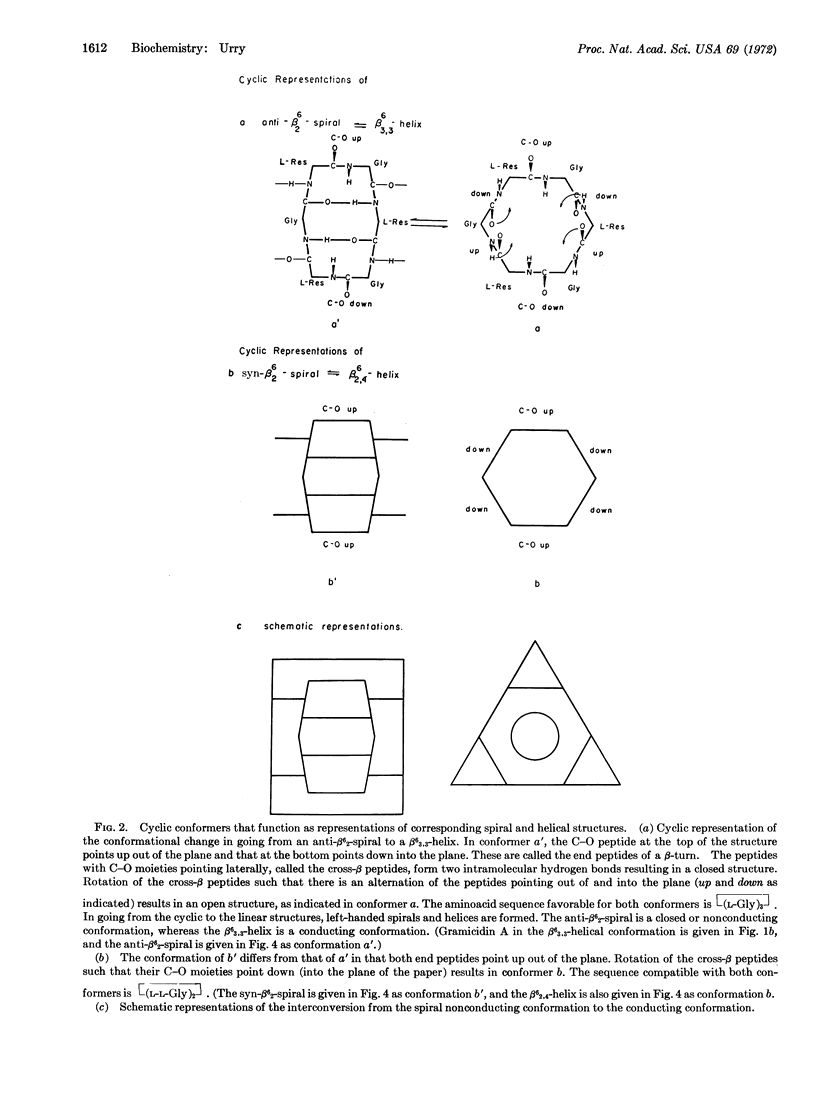
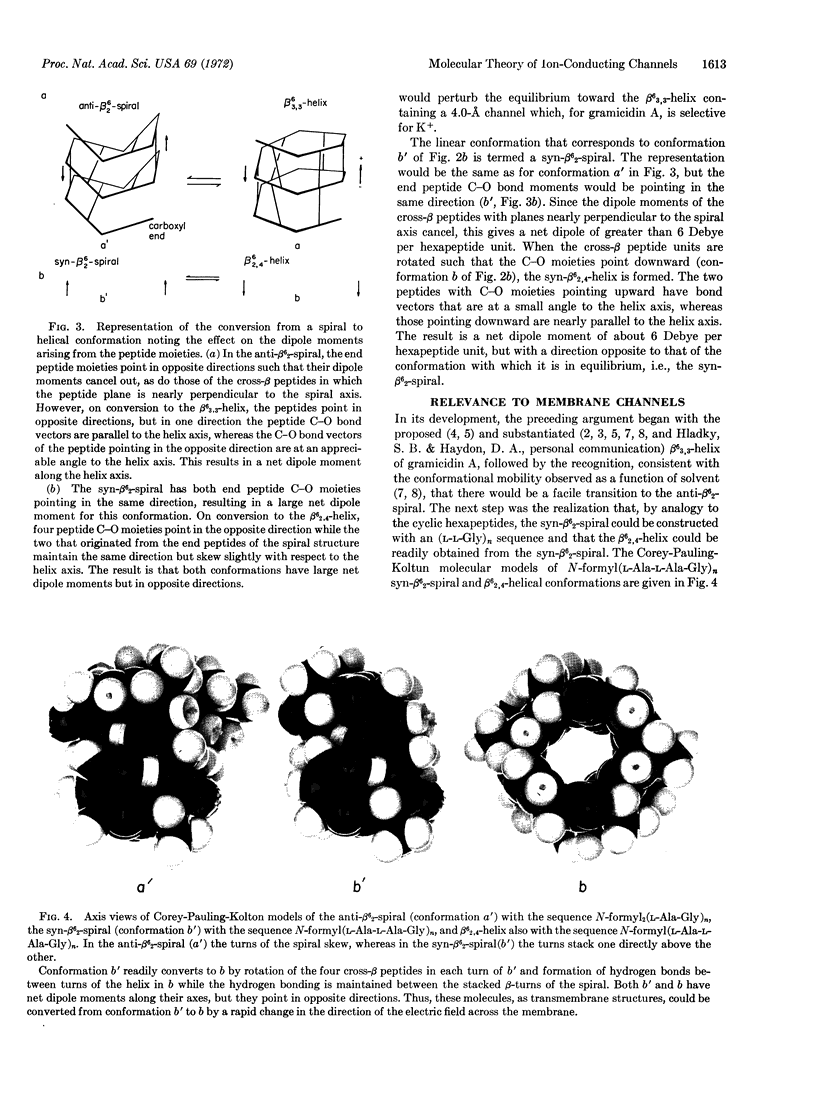
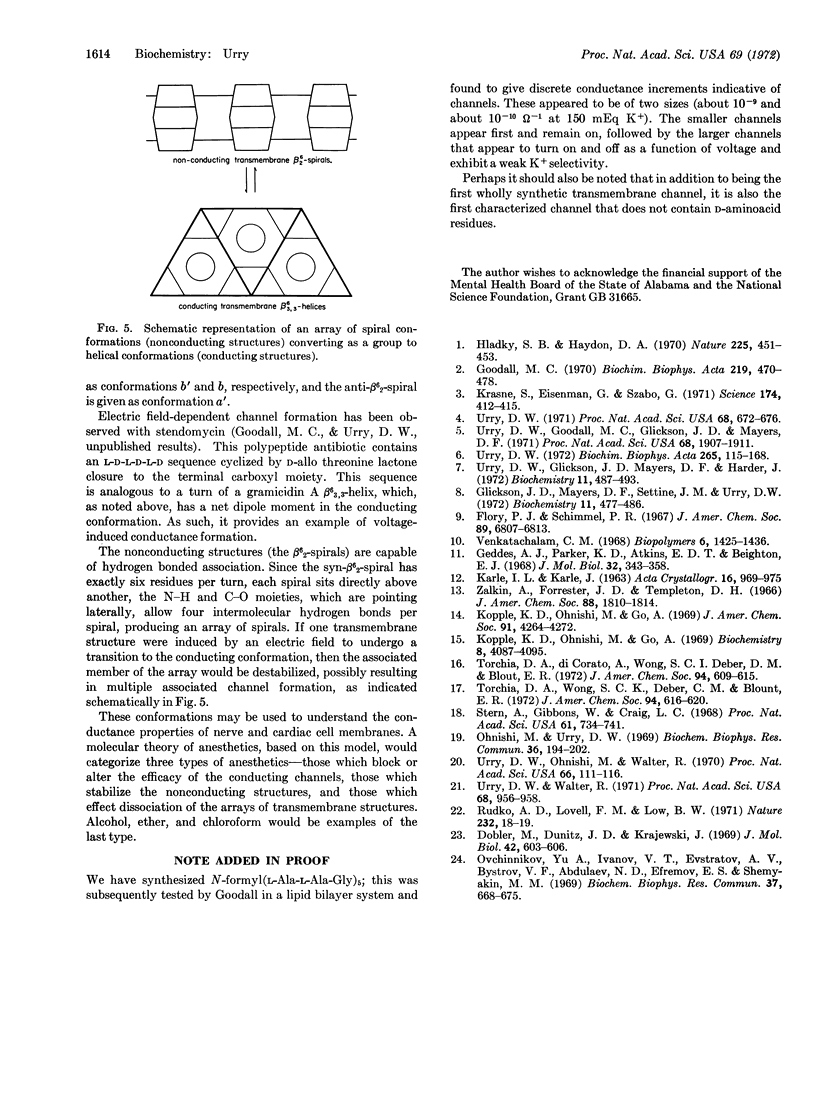
Images in this article
Selected References
These references are in PubMed. This may not be the complete list of references from this article.
- Dobler M., Dunitz J. D., Krajewski J. Structure of the K+ complex with enniatin B, a macrocyclic antibiotic with K+ transport properties. J Mol Biol. 1969 Jun 28;42(3):603–606. doi: 10.1016/0022-2836(69)90249-6. [DOI] [PubMed] [Google Scholar]
- Flory P. J., Schimmel P. R. Dipole moments in relation to configuration of polypeptide chains. J Am Chem Soc. 1967 Dec 20;89(26):6807–6813. doi: 10.1021/ja01002a001. [DOI] [PubMed] [Google Scholar]
- Geddes A. J., Parker K. D., Atkins E. D., Beighton E. "Cross-beta" conformation in proteins. J Mol Biol. 1968 Mar 14;32(2):343–358. doi: 10.1016/0022-2836(68)90014-4. [DOI] [PubMed] [Google Scholar]
- Glickson J. D., Mayers D. F., Settine J. M., Urry D. W. Spectroscopic studies on the conformation of gramicidin A'. Proton magnetic resonance assignments, coupling constants, and H-D exchange. Biochemistry. 1972 Feb 15;11(4):477–486. doi: 10.1021/bi00754a001. [DOI] [PubMed] [Google Scholar]
- Goodall M. C. Structural effects in the action of antibiotics on the ion permeability of lipid bilayers. 3. Gramicidins "A" and "S", and lipid specificity. Biochim Biophys Acta. 1970 Dec 1;219(2):471–478. doi: 10.1016/0005-2736(70)90225-7. [DOI] [PubMed] [Google Scholar]
- Hladky S. B., Haydon D. A. Discreteness of conductance change in bimolecular lipid membranes in the presence of certain antibiotics. Nature. 1970 Jan 31;225(5231):451–453. doi: 10.1038/225451a0. [DOI] [PubMed] [Google Scholar]
- Kopple K. D., Ohnishi M., Go A. Conformations of cyclic peptides. IV. Nuclear magnetic resonance studies of cyclo-pentaglycyl-L-leucyl and cyclo-diglycyl-L-histidyldiglycyl-L-tyrosyl. Biochemistry. 1969 Oct;8(10):4087–4095. doi: 10.1021/bi00838a028. [DOI] [PubMed] [Google Scholar]
- Krasne S., Eisenman G., Szabo G. Freezing and melting of lipid bilayers and the mode of action of nonactin, valinomycin, and gramicidin. Science. 1971 Oct 22;174(4007):412–415. doi: 10.1126/science.174.4007.412. [DOI] [PubMed] [Google Scholar]
- Ohnishi M., Urry D. W. Temperature dependence of amide proton chemical shifts: the secondary structures of gramicidin S and valinomycin. Biochem Biophys Res Commun. 1969 Jul 23;36(2):194–202. doi: 10.1016/0006-291x(69)90314-3. [DOI] [PubMed] [Google Scholar]
- Ovchinnikov Y. A., Ivanov V. T., Evstratov A. V., Bystrov V. F., Abdullaev N. D., Popov E. M., Lipkind G. M., Arkhipova S. F., Efremov E. S., Shemyakin M. M. The physicochemical basis of the functioning of biological membranes: dynamic conformational properties of enniatin B and its K+ complex in solution. Biochem Biophys Res Commun. 1969 Nov 6;37(4):668–676. doi: 10.1016/0006-291x(69)90863-8. [DOI] [PubMed] [Google Scholar]
- Rudko A. D., Lovell F. M., Low B. W. Prolactin synthesis in primates. Nat New Biol. 1971 Jul 7;232(27):18–19. [PubMed] [Google Scholar]
- Stern A., Gibbons W. A., Craig L. C. A conformational analysis of gramicidin S-A by nuclear magnetic resonance. Proc Natl Acad Sci U S A. 1968 Oct;61(2):734–741. doi: 10.1073/pnas.61.2.734. [DOI] [PMC free article] [PubMed] [Google Scholar]
- Torchia D. A., Di Corato A., Wong S. C., Deber C. M., Blout E. R. Cyclic peptides. II. Solution conformations of cyclo(ProlyLserylglycylprolylserylglycyl) from nuclear magnetic resonance. J Am Chem Soc. 1972 Jan 26;94(2):609–615. doi: 10.1021/ja00757a048. [DOI] [PubMed] [Google Scholar]
- Torchia D. A., Wong S. C., Deber C. M., Blout E. R. Cyclic peptides. 3. Solution conformations of cyclo(serylprolylglycylserylprolylglycyl) from nuclear magnetic resonance. J Am Chem Soc. 1972 Jan 26;94(2):616–620. doi: 10.1021/ja00757a049. [DOI] [PubMed] [Google Scholar]
- Urry D. W., Glickson J. D., Mayers D. F., Haider J. Spectroscopic studies on the conformation of gramicidin A'. Evidence for a new helical conformation. Biochemistry. 1972 Feb 15;11(4):487–493. doi: 10.1021/bi00754a002. [DOI] [PubMed] [Google Scholar]
- Urry D. W., Goodall M. C., Glickson J. D., Mayers D. F. The gramicidin A transmembrane channel: characteristics of head-to-head dimerized (L,D) helices. Proc Natl Acad Sci U S A. 1971 Aug;68(8):1907–1911. doi: 10.1073/pnas.68.8.1907. [DOI] [PMC free article] [PubMed] [Google Scholar]
- Urry D. W., Ohnishi M., Walter R. Secondary structure of the cyclic moiety of the peptide hormone oxytocin and its deamino analog. Proc Natl Acad Sci U S A. 1970 May;66(1):111–116. doi: 10.1073/pnas.66.1.111. [DOI] [PMC free article] [PubMed] [Google Scholar]
- Urry D. W. Protein conformation in biomembranes: optical rotation and absorption of membrane suspensions. Biochim Biophys Acta. 1972 Feb 14;265(1):115–168. doi: 10.1016/0304-4157(72)90021-4. [DOI] [PubMed] [Google Scholar]
- Urry D. W. The gramicidin A transmembrane channel: a proposed pi(L,D) helix. Proc Natl Acad Sci U S A. 1971 Mar;68(3):672–676. doi: 10.1073/pnas.68.3.672. [DOI] [PMC free article] [PubMed] [Google Scholar]
- Urry D. W., Walter R. Proposed conformation of oxytocin in solution. Proc Natl Acad Sci U S A. 1971 May;68(5):956–958. doi: 10.1073/pnas.68.5.956. [DOI] [PMC free article] [PubMed] [Google Scholar]
- Venkatachalam C. M. Stereochemical criteria for polypeptides and proteins. V. Conformation of a system of three linked peptide units. Biopolymers. 1968 Oct;6(10):1425–1436. doi: 10.1002/bip.1968.360061006. [DOI] [PubMed] [Google Scholar]
- Zalkin A., Forrester J. D., Templeton D. H. Ferrichrome-A tetrahydrate. Determination of crystal and molecular structure. J Am Chem Soc. 1966 Apr 20;88(8):1810–1814. doi: 10.1021/ja00960a040. [DOI] [PubMed] [Google Scholar]