Abstract
Ultrastructural analysis of 3T3 fibroblasts by freeze-cleavage has demonstrated significant changes in cell-membrane structure associated with cell-to-cell contact and malignant transformation. These changes consist of a rearrangement and redistribution of intramembranous particles on the membrane fracture faces exposed by freeze-cleavage. The results show that noncontacted 3T3 cells in low density contain randomly distributed intramembranous particles. With the development of cell-to-cell contacts during the logarithmic phase of growth however, a pronounced aggregation of intramembranous particles is seen. A direct correlation between the degree of cell contact and the percentage of cells showing intramembranous-particle aggregation has been established. By contrast, transformed SV3T3 and SP3T3 cells show no evidence of intramembranous-particle aggregation even at cell densities where cell-to-cell contact is extensive. In view of recent reports that intramembranous particles represent foci of interaction between certain intrinsic membrane proteins and lipids, we propose that cell-to-cell contact of nontransformed 3T3 cells may initiate a change in the distribution of intrinsic membrane proteins associated with intramembranous particles and that these changes may influence control of cell proliferation.
Keywords: SV40, 3T3, freeze-cleavage, electron microscopy, intramembranous particles, intrinsic membrane proteins, cell proliferation
Full text
PDF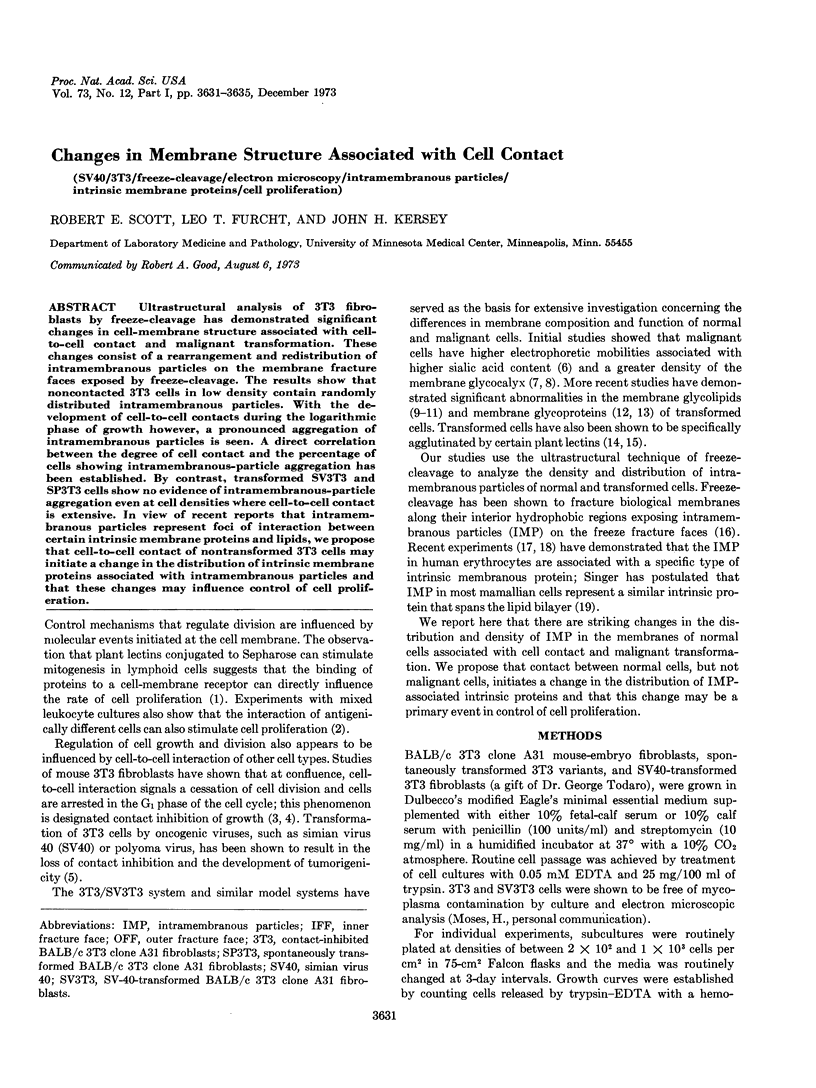
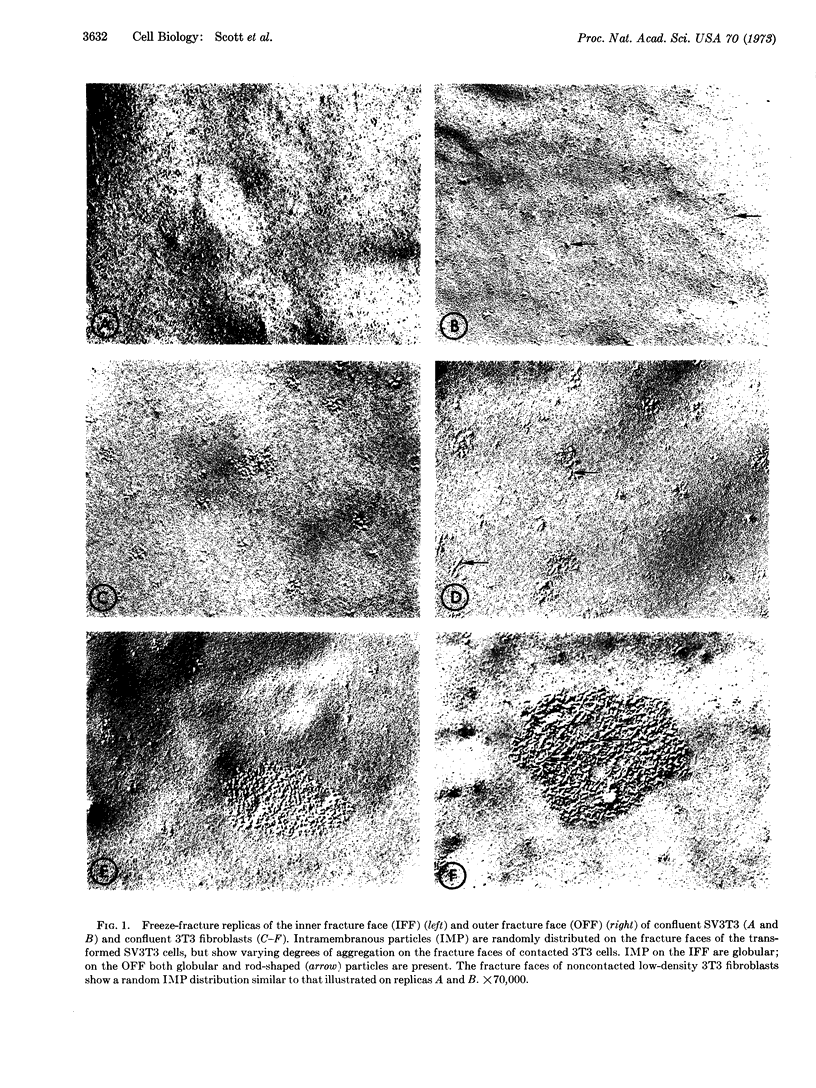
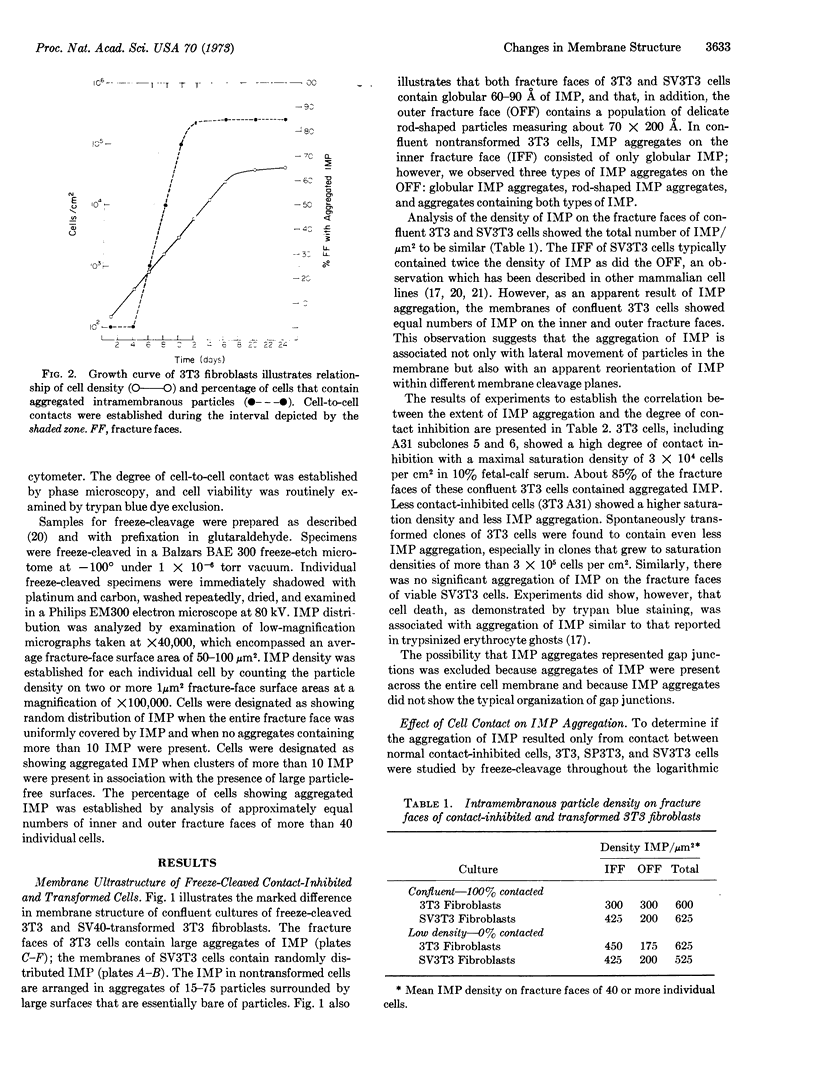
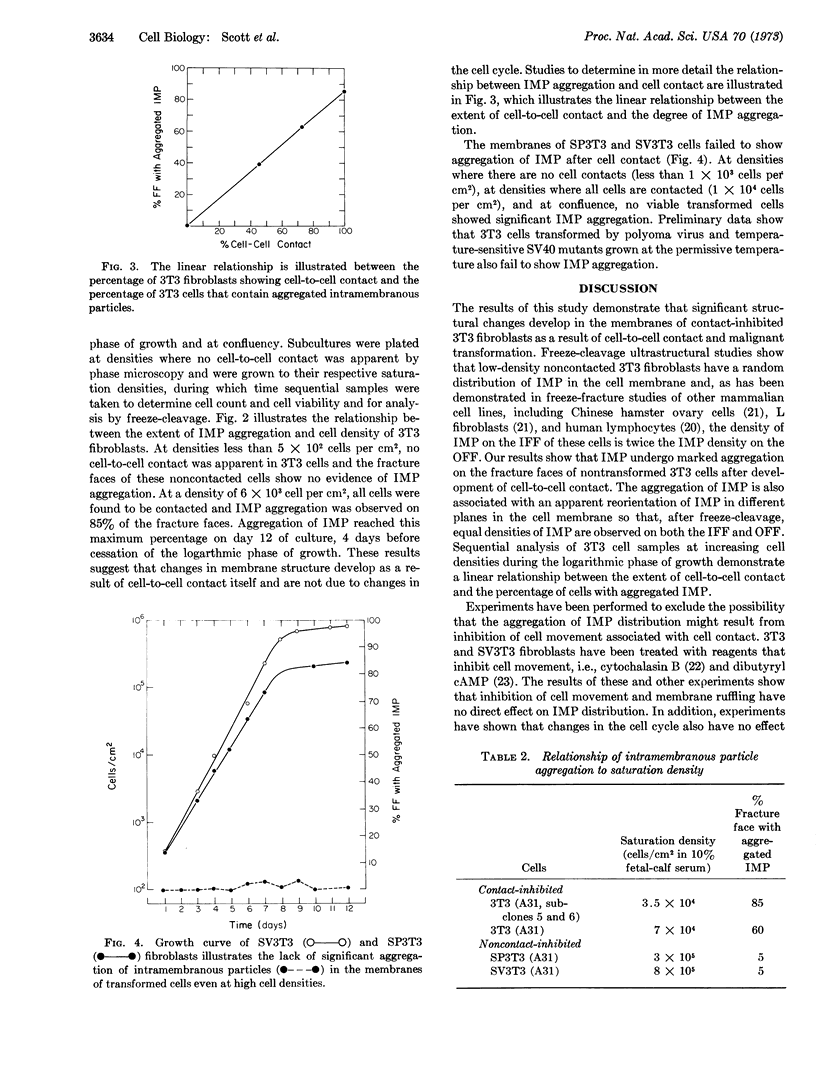
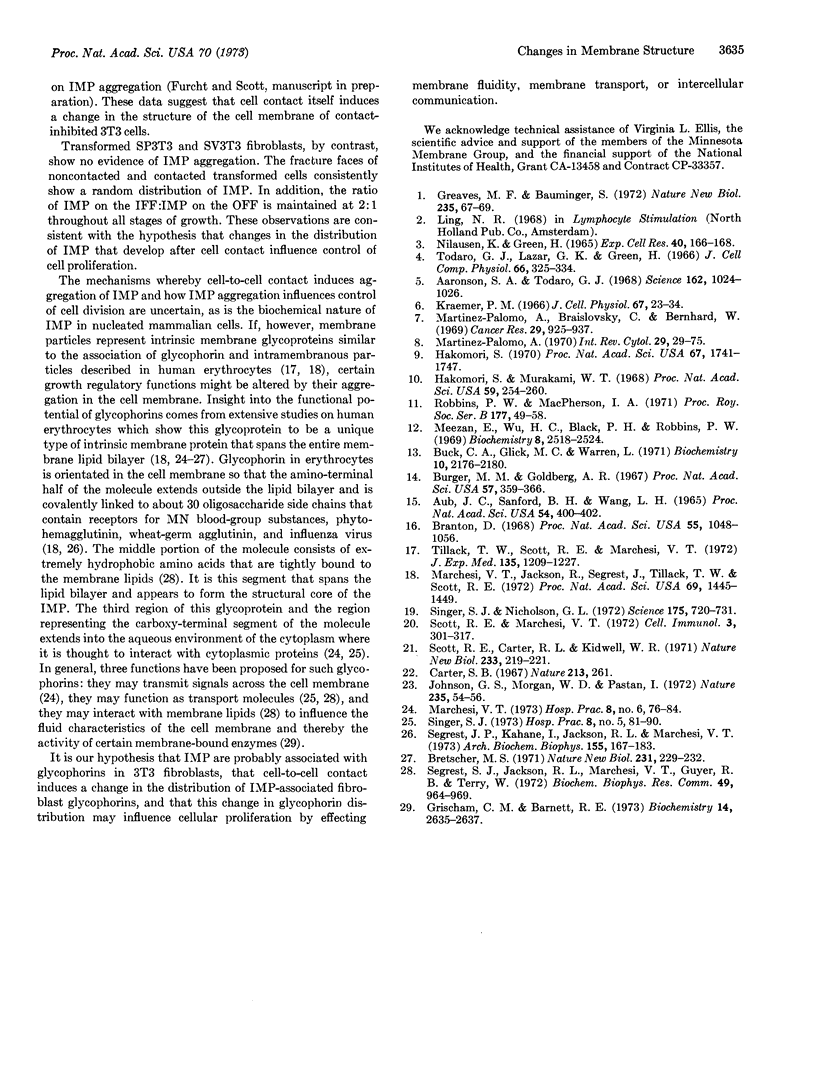
Images in this article
Selected References
These references are in PubMed. This may not be the complete list of references from this article.
- Aaronson S. A., Todaro G. J. Basis for the acquisition of malignant potential by mouse cells cultivated in vitro. Science. 1968 Nov 29;162(3857):1024–1026. doi: 10.1126/science.162.3857.1024. [DOI] [PubMed] [Google Scholar]
- Aub J. C., Sanford B. H., Wang L. H. Reactions of normal and leukemic cell surfaces to a wheat germ agglutinin. Proc Natl Acad Sci U S A. 1965 Aug;54(2):400–402. doi: 10.1073/pnas.54.2.400. [DOI] [PMC free article] [PubMed] [Google Scholar]
- Branton D. Fracture faces of frozen membranes. Proc Natl Acad Sci U S A. 1966 May;55(5):1048–1056. doi: 10.1073/pnas.55.5.1048. [DOI] [PMC free article] [PubMed] [Google Scholar]
- Bretscher M. S. Major human erythrocyte glycoprotein spans the cell membrane. Nat New Biol. 1971 Jun 23;231(25):229–232. doi: 10.1038/newbio231229a0. [DOI] [PubMed] [Google Scholar]
- Buck C. A., Glick M. C., Warren L. Effect of growth on the glycoproteins from the surface of control and Rous sarcoma virus transformed hamster cells. Biochemistry. 1971 May 25;10(11):2176–2180. doi: 10.1021/bi00787a034. [DOI] [PubMed] [Google Scholar]
- Burger M. M., Goldberg A. R. Identification of a tumor-specific determinant on neoplastic cell surfaces. Proc Natl Acad Sci U S A. 1967 Feb;57(2):359–366. doi: 10.1073/pnas.57.2.359. [DOI] [PMC free article] [PubMed] [Google Scholar]
- Carter S. B. Effects of cytochalasins on mammalian cells. Nature. 1967 Jan 21;213(5073):261–264. doi: 10.1038/213261a0. [DOI] [PubMed] [Google Scholar]
- Greaves M. F., Bauminger S. Activation of T and B lymphocytes by insoluble phytomitogens. Nat New Biol. 1972 Jan 19;235(55):67–70. doi: 10.1038/newbio235067a0. [DOI] [PubMed] [Google Scholar]
- Grisham C. M., Barnett R. E. The role of lipid-phase transitions in the regulation of the (sodium + potassium) adenosine triphosphatase. Biochemistry. 1973 Jul 3;12(14):2635–2637. doi: 10.1021/bi00738a013. [DOI] [PubMed] [Google Scholar]
- Hakomori S. I., Murakami W. T. Glycolipids of hamster fibroblasts and derived malignant-transformed cell lines. Proc Natl Acad Sci U S A. 1968 Jan;59(1):254–261. doi: 10.1073/pnas.59.1.254. [DOI] [PMC free article] [PubMed] [Google Scholar]
- Hakomori S. Cell density-dependent changes of glycolipid concentrations in fibroblasts, and loss of this response in virus-transformed cells. Proc Natl Acad Sci U S A. 1970 Dec;67(4):1741–1747. doi: 10.1073/pnas.67.4.1741. [DOI] [PMC free article] [PubMed] [Google Scholar]
- Johnson G. S., Morgan W. D., Pastan I. Regulation of cell motility by cyclic AMP. Nature. 1972 Jan 7;235(5332):54–56. doi: 10.1038/235054a0. [DOI] [PubMed] [Google Scholar]
- Kraemer P. M. Sialic acid of mammalian cell lines. J Cell Physiol. 1966 Feb;67(1):23–34. doi: 10.1002/jcp.1040670104. [DOI] [PubMed] [Google Scholar]
- Marchesi V. T., Tillack T. W., Jackson R. L., Segrest J. P., Scott R. E. Chemical characterization and surface orientation of the major glycoprotein of the human erythrocyte membrane. Proc Natl Acad Sci U S A. 1972 Jun;69(6):1445–1449. doi: 10.1073/pnas.69.6.1445. [DOI] [PMC free article] [PubMed] [Google Scholar]
- Martinez-Palomo A., Braislovsky C., Bernhard W. Ultrastructural modifications of the cell surface and intercellular contacts of some transformed cell strains. Cancer Res. 1969 Apr;29(4):925–937. [PubMed] [Google Scholar]
- Meezan E., Wu H. C., Black P. H., Robbins P. W. Comparative studies on the carbohydrate-containing membrane components of normal and virus-transformed mouse fibroblasts. II. Separation of glycoproteins and glycopeptides by sephadex chromatography. Biochemistry. 1969 Jun;8(6):2518–2524. doi: 10.1021/bi00834a039. [DOI] [PubMed] [Google Scholar]
- Nilausen K., Green H. Reversible arrest of growth in G1 of an established fibroblast line (3T3). Exp Cell Res. 1965 Oct;40(1):166–168. doi: 10.1016/0014-4827(65)90306-x. [DOI] [PubMed] [Google Scholar]
- Robbins P. W., Macpherson I. A. Glycolipid synthesis in normal and transformed animal cells. Proc R Soc Lond B Biol Sci. 1971 Feb 16;177(1046):49–58. doi: 10.1098/rspb.1971.0014. [DOI] [PubMed] [Google Scholar]
- Scott R. E., Carter R. L., Kidwell W. R. Structural changes in memebranes of synchronized cells demonstrated by freeze-cleavage. Nature. 1971 Oct 13;233(5320):219–220. [PubMed] [Google Scholar]
- Scott R. E., Marchesi V. T. Structural changes in membranes of transformed lymphocytes demonstrated by freeze-etching. Cell Immunol. 1972 Feb;3(2):301–317. doi: 10.1016/0008-8749(72)90169-4. [DOI] [PubMed] [Google Scholar]
- Segrest J. P., Jackson R. L., Marchesi V. T., Guyer R. B., Terry W. Red cell membrane glycoprotein: amino acid sequence of an intramembranous region. Biochem Biophys Res Commun. 1972 Nov 15;49(4):964–969. doi: 10.1016/0006-291x(72)90306-3. [DOI] [PubMed] [Google Scholar]
- Segrest J. P., Kahane I., Jackson R. L., Marchesi V. T. Major glycoprotein of the human erythrocyte membrane: evidence for an amphipathic molecular structure. Arch Biochem Biophys. 1973 Mar;155(1):167–183. doi: 10.1016/s0003-9861(73)80019-0. [DOI] [PubMed] [Google Scholar]
- Singer S. J., Nicolson G. L. The fluid mosaic model of the structure of cell membranes. Science. 1972 Feb 18;175(4023):720–731. doi: 10.1126/science.175.4023.720. [DOI] [PubMed] [Google Scholar]
- Tillack T. W., Scott R. E., Marchesi V. T. The structure of erythrocyte membranes studied by freeze-etching. II. Localization of receptors for phytohemagglutinin and influenza virus to the intramembranous particles. J Exp Med. 1972 Jun 1;135(6):1209–1227. doi: 10.1084/jem.135.6.1209. [DOI] [PMC free article] [PubMed] [Google Scholar]
- Todaro G. J., Lazar G. K., Green H. The initiation of cell division in a contact-inhibited mammalian cell line. J Cell Physiol. 1965 Dec;66(3):325–333. doi: 10.1002/jcp.1030660310. [DOI] [PubMed] [Google Scholar]