Abstract
We report that the light-activated bovine metarhodopsin II, upon decay, first forms opsin in the correctly folded form. The latter binds 11-cis-retinal and regenerates the native rhodopsin chromophore. However, when the opsin formed upon metarhodopsin II decay is kept in 0.1% dodecyl maltoside, it converts in a time-dependent manner to a form(s) that does not bind 11-cis-retinal. On subsequent addition of 11-cis-retinal, slow reversal of the non-retinal-binding forms to the correctly folded retinal-binding form has been demonstrated. We have studied the influence, on the above interconversions, of pH, phospholipids (rod outer segment and soybean), dithiothreitol, and a mixture of reduced and oxidized glutathione. Chromophore regeneration in the presence of 11-cis-retinal was highest at pH 6.0-6.3. The addition of dithiothreitol just before bleaching gave back only a small amount (7%) of rhodopsin on the subsequent addition of 11-cis-retinal, whereas the slow phase(s) of chromophore formation was completely abolished. The presence of a mixture of reduced and oxidized glutathione did not significantly affect the results. Addition of phospholipids, either from soybean or rod outer segment, prior to bleaching stabilized the initially formed opsin, resulting in much higher chromophore regeneration. However, addition of the phospholipids after conversion of the opsin to non-retinal-binding form(s) arrested the subsequent reversal of the opsin to the retinal-binding form.
Full text
PDF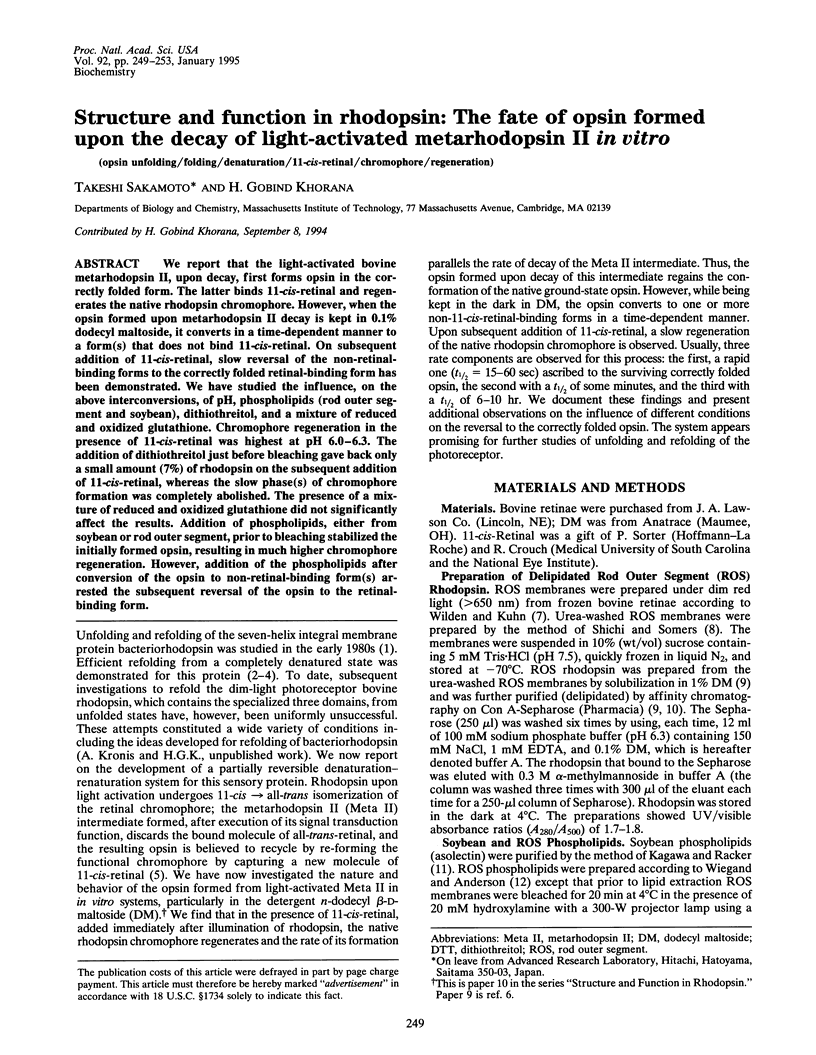
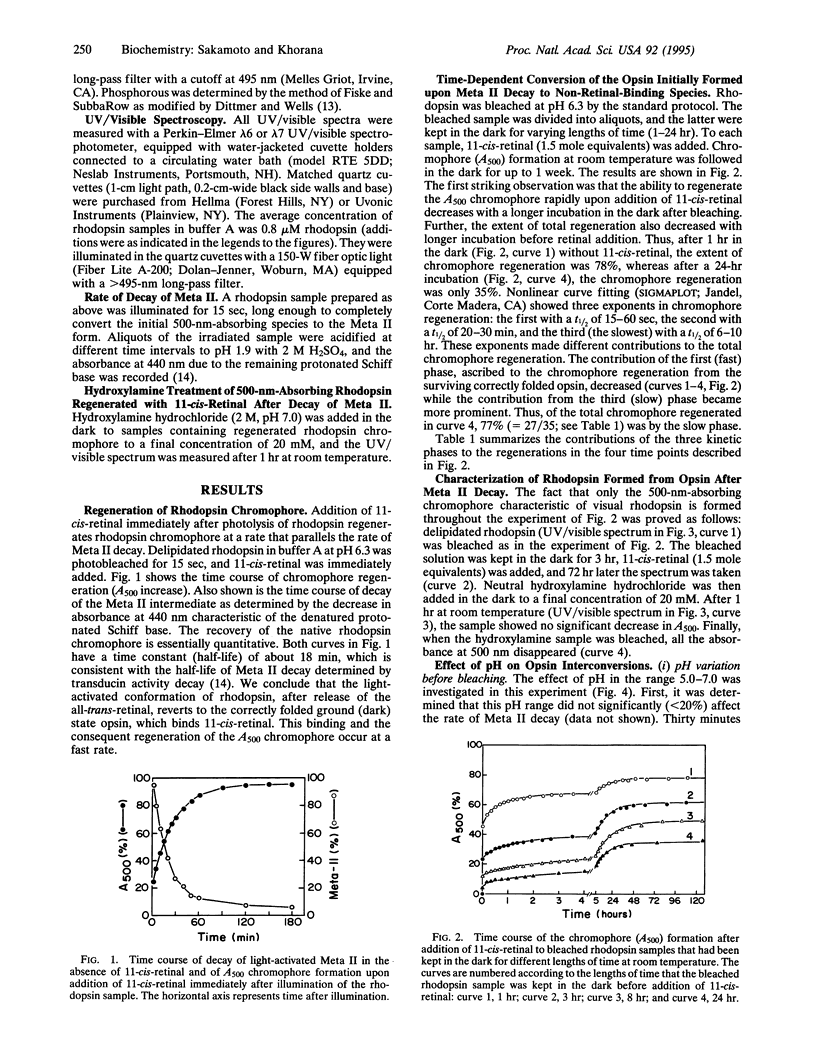
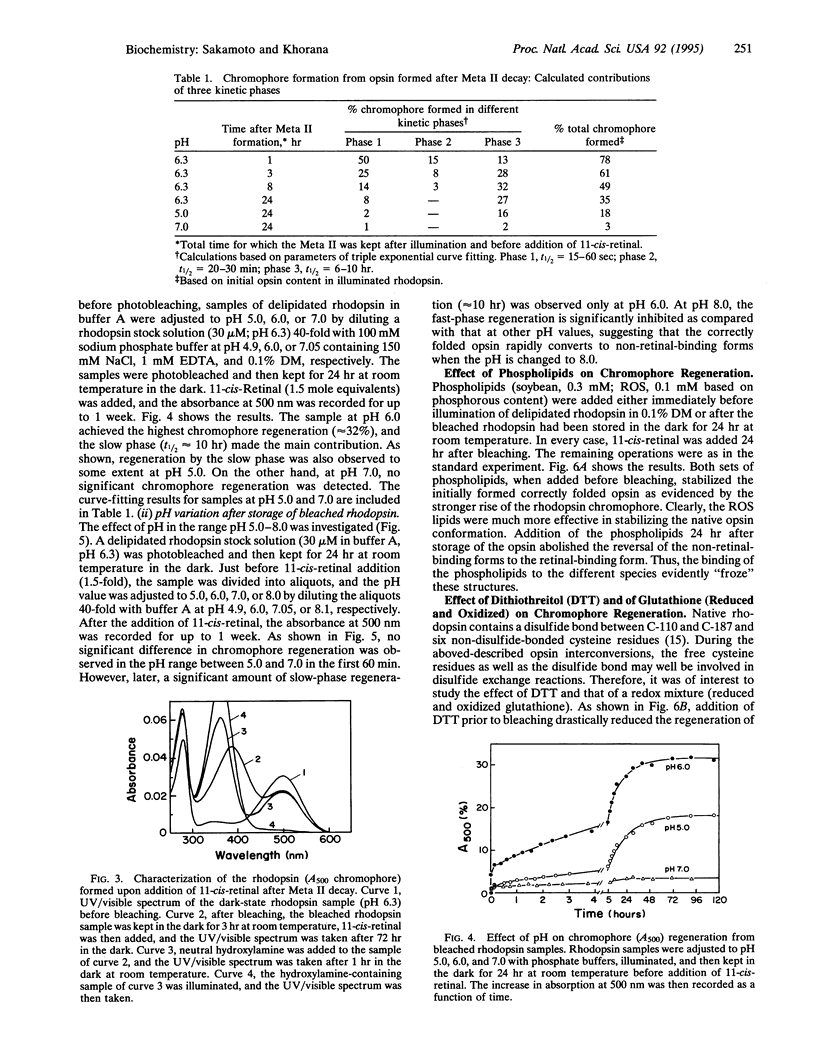
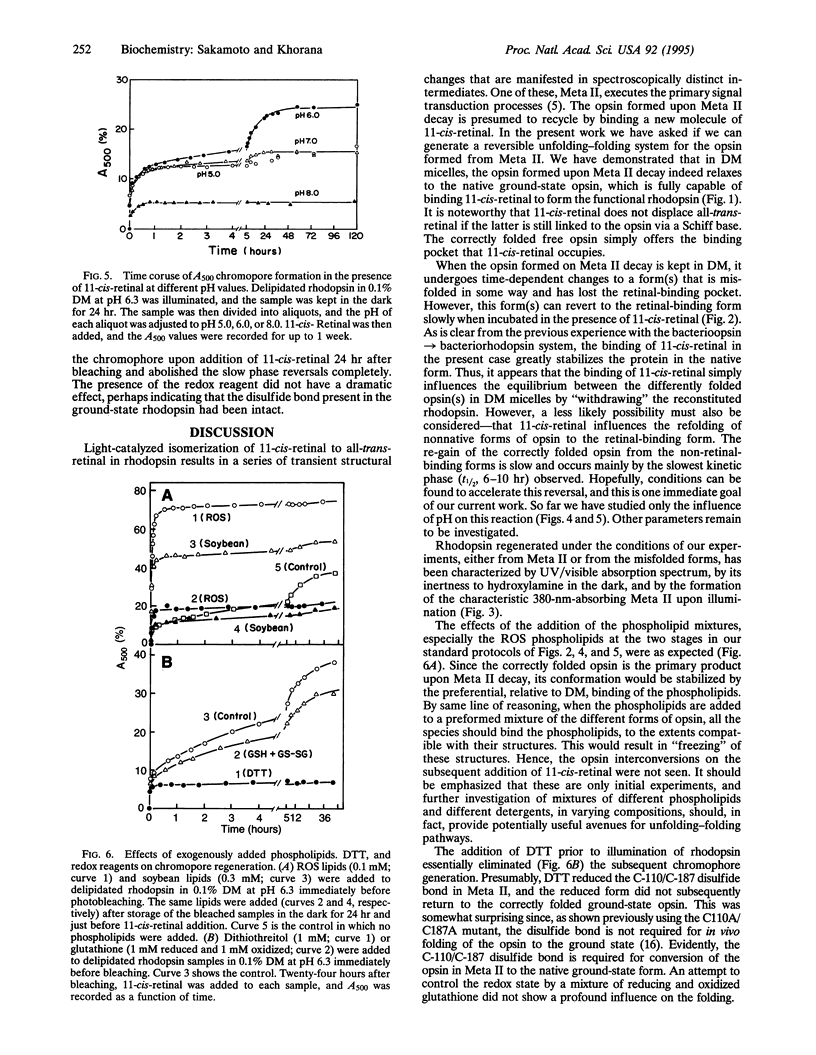
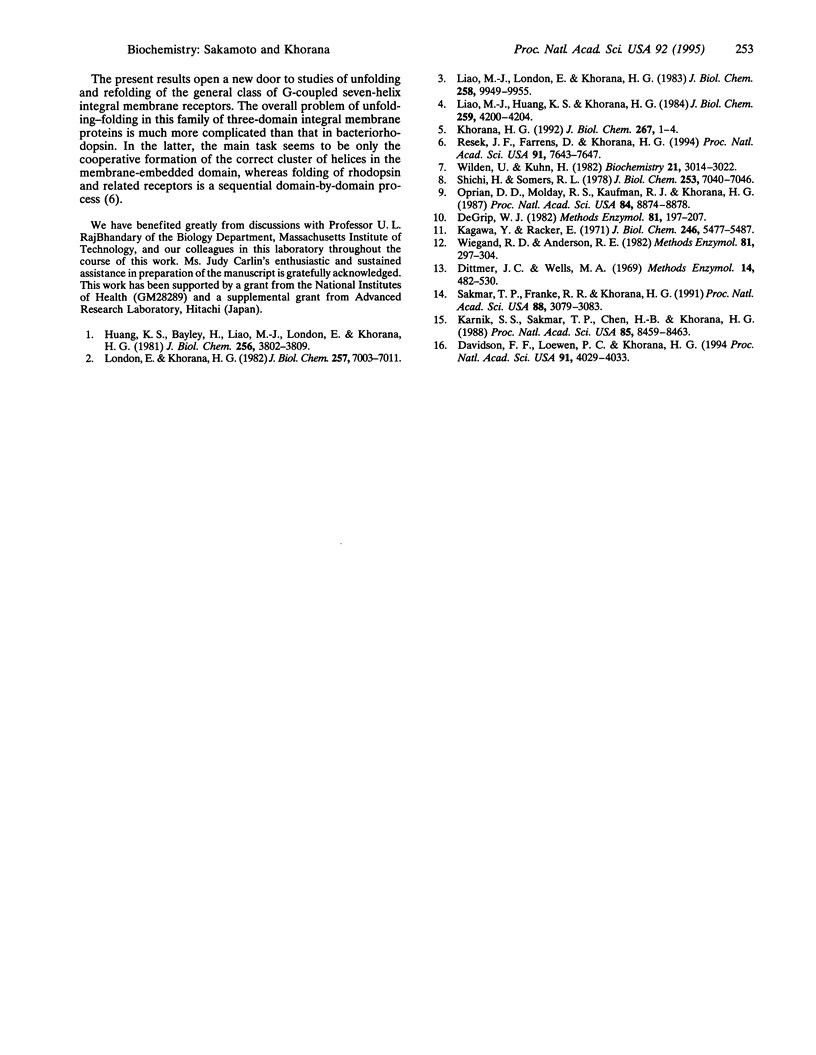
Selected References
These references are in PubMed. This may not be the complete list of references from this article.
- Davidson F. F., Loewen P. C., Khorana H. G. Structure and function in rhodopsin: replacement by alanine of cysteine residues 110 and 187, components of a conserved disulfide bond in rhodopsin, affects the light-activated metarhodopsin II state. Proc Natl Acad Sci U S A. 1994 Apr 26;91(9):4029–4033. doi: 10.1073/pnas.91.9.4029. [DOI] [PMC free article] [PubMed] [Google Scholar]
- De Grip W. J. Purification of bovine rhodopsin over concanavalin A--sepharose. Methods Enzymol. 1982;81:197–207. doi: 10.1016/s0076-6879(82)81032-x. [DOI] [PubMed] [Google Scholar]
- Huang K. S., Bayley H., Liao M. J., London E., Khorana H. G. Refolding of an integral membrane protein. Denaturation, renaturation, and reconstitution of intact bacteriorhodopsin and two proteolytic fragments. J Biol Chem. 1981 Apr 25;256(8):3802–3809. [PubMed] [Google Scholar]
- Karnik S. S., Sakmar T. P., Chen H. B., Khorana H. G. Cysteine residues 110 and 187 are essential for the formation of correct structure in bovine rhodopsin. Proc Natl Acad Sci U S A. 1988 Nov;85(22):8459–8463. doi: 10.1073/pnas.85.22.8459. [DOI] [PMC free article] [PubMed] [Google Scholar]
- Khorana H. G. Rhodopsin, photoreceptor of the rod cell. An emerging pattern for structure and function. J Biol Chem. 1992 Jan 5;267(1):1–4. [PubMed] [Google Scholar]
- Liao M. J., Huang K. S., Khorana H. G. Regeneration of native bacteriorhodopsin structure from fragments. J Biol Chem. 1984 Apr 10;259(7):4200–4204. [PubMed] [Google Scholar]
- Liao M. J., London E., Khorana H. G. Regeneration of the native bacteriorhodopsin structure from two chymotryptic fragments. J Biol Chem. 1983 Aug 25;258(16):9949–9955. [PubMed] [Google Scholar]
- London E., Khorana H. G. Denaturation and renaturation of bacteriorhodopsin in detergents and lipid-detergent mixtures. J Biol Chem. 1982 Jun 25;257(12):7003–7011. [PubMed] [Google Scholar]
- Oprian D. D., Molday R. S., Kaufman R. J., Khorana H. G. Expression of a synthetic bovine rhodopsin gene in monkey kidney cells. Proc Natl Acad Sci U S A. 1987 Dec;84(24):8874–8878. doi: 10.1073/pnas.84.24.8874. [DOI] [PMC free article] [PubMed] [Google Scholar]
- Resek J. F., Farrens D., Khorana H. G. Structure and function in rhodopsin: covalent crosslinking of the rhodopsin (metarhodopsin II)-transducin complex--the rhodopsin cytoplasmic face links to the transducin alpha subunit. Proc Natl Acad Sci U S A. 1994 Aug 2;91(16):7643–7647. doi: 10.1073/pnas.91.16.7643. [DOI] [PMC free article] [PubMed] [Google Scholar]
- Sakmar T. P., Franke R. R., Khorana H. G. The role of the retinylidene Schiff base counterion in rhodopsin in determining wavelength absorbance and Schiff base pKa. Proc Natl Acad Sci U S A. 1991 Apr 15;88(8):3079–3083. doi: 10.1073/pnas.88.8.3079. [DOI] [PMC free article] [PubMed] [Google Scholar]
- Shichi H., Somers R. L. Light-dependent phosphorylation of rhodopsin. Purification and properties of rhodopsin kinase. J Biol Chem. 1978 Oct 10;253(19):7040–7046. [PubMed] [Google Scholar]
- Wiegand R. D., Anderson R. E. Determination of molecular species of rod outer segment phospholipids. Methods Enzymol. 1982;81:297–304. doi: 10.1016/s0076-6879(82)81046-x. [DOI] [PubMed] [Google Scholar]
- Wilden U., Kühn H. Light-dependent phosphorylation of rhodopsin: number of phosphorylation sites. Biochemistry. 1982 Jun 8;21(12):3014–3022. doi: 10.1021/bi00541a032. [DOI] [PubMed] [Google Scholar]