Abstract
Carotid body-mediated ventilatory increases in response to acute hypoxia are attenuated in animals reared in an hypoxic environment. Normally, O2-sensitive K+ channels in neurosecretory type I carotid body cells are intimately involved in excitation of the intact organ by hypoxia. We have therefore studied K+ channels and their sensitivity to acute hypoxia (PO2 12-20 mmHg) in type I cells isolated from neonatal rats born and reared in normoxic and hypoxic environments. When compared with cells from normoxic rats, K+ current density in cells from hypoxic rats was significantly reduced, whereas Ca2+ current density was unaffected. Charybdotoxin (20 nM) inhibited K+ currents in cells from normoxic rats by approximately 25% but was without significant effect in cells from hypoxic rats. However, hypoxia caused similar, reversible inhibitions of K+ currents in cells from the two groups. Resting membrane potentials (measured at 37 degrees C using the perforated-patch technique) were similar in normoxic and hypoxic rats. However, although acute hypoxia depolarized type I cells of normoxic rats, it was without effect on membrane potential in type I cells from hypoxic animals. Charybdotoxin (20 nM) also depolarized cells from normoxic rats. Our results suggest that type I cells from chronically hypoxic rats, like normoxic rats, possess O2-sensing mechanisms. However, they lack charybdotoxin-sensitive K+ channels that contribute to resting membrane potential in normoxically reared rats, and this appears to prevent them from depolarizing (and hence triggering Ca2+ influx and neurosecretion) during acute hypoxia.
Full text
PDF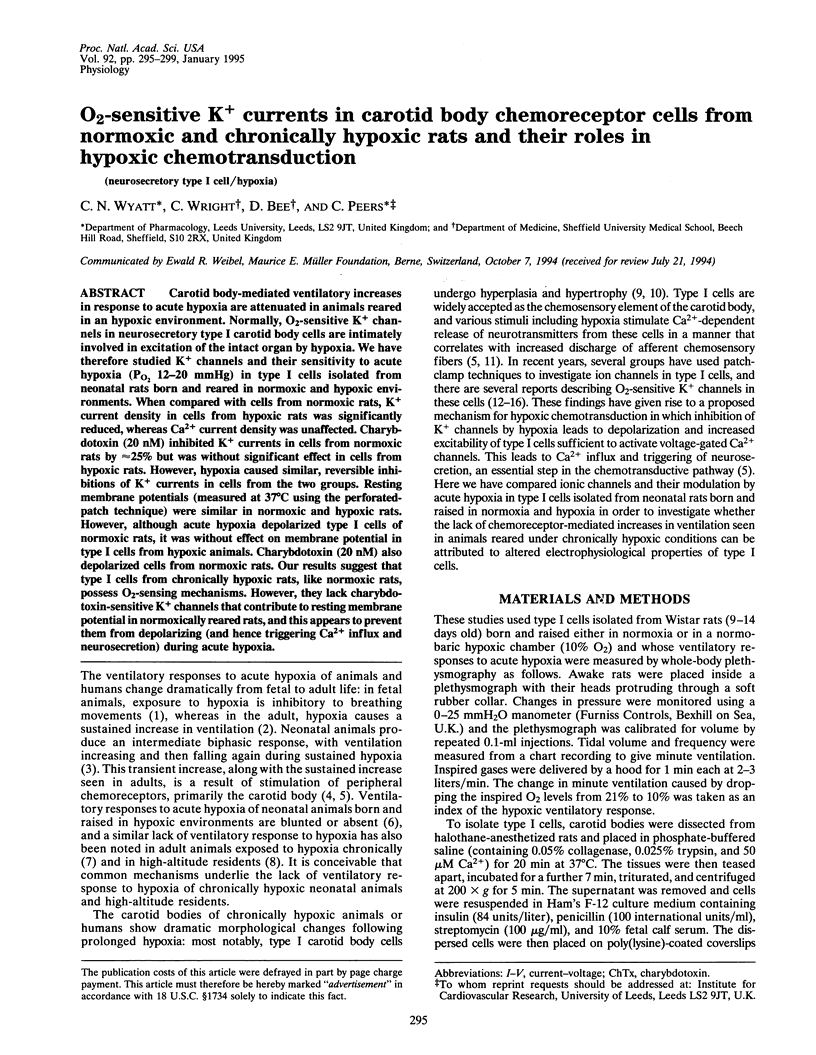
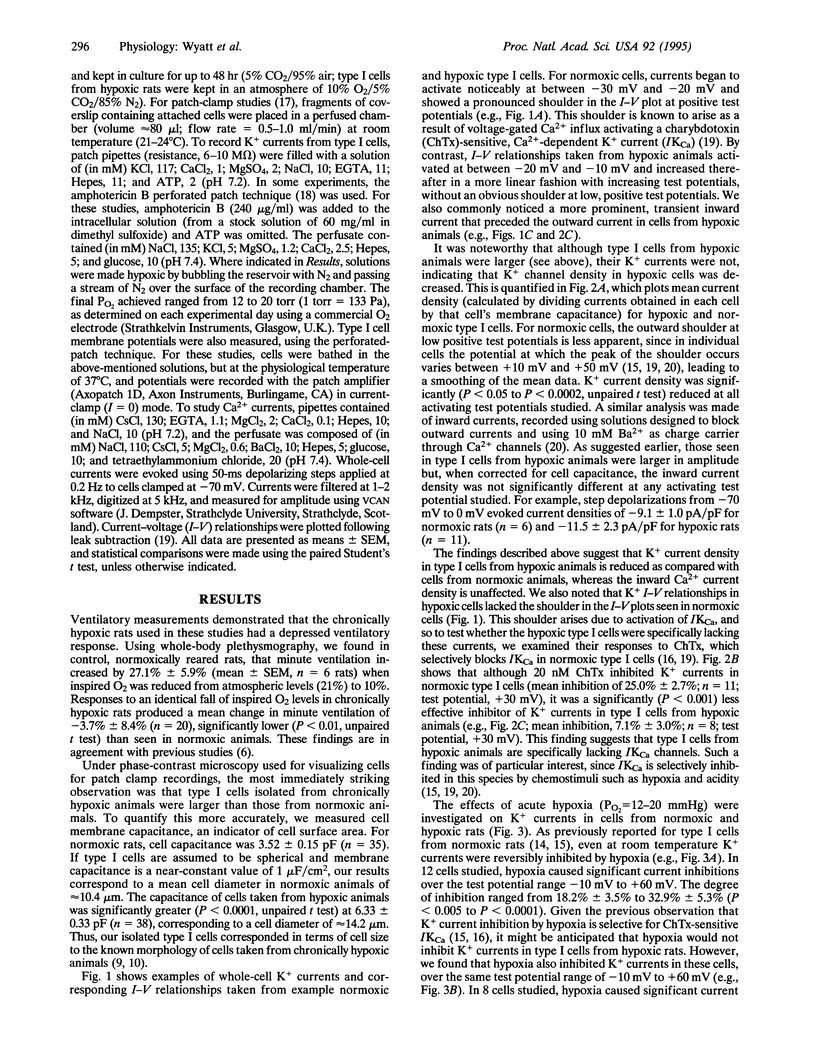
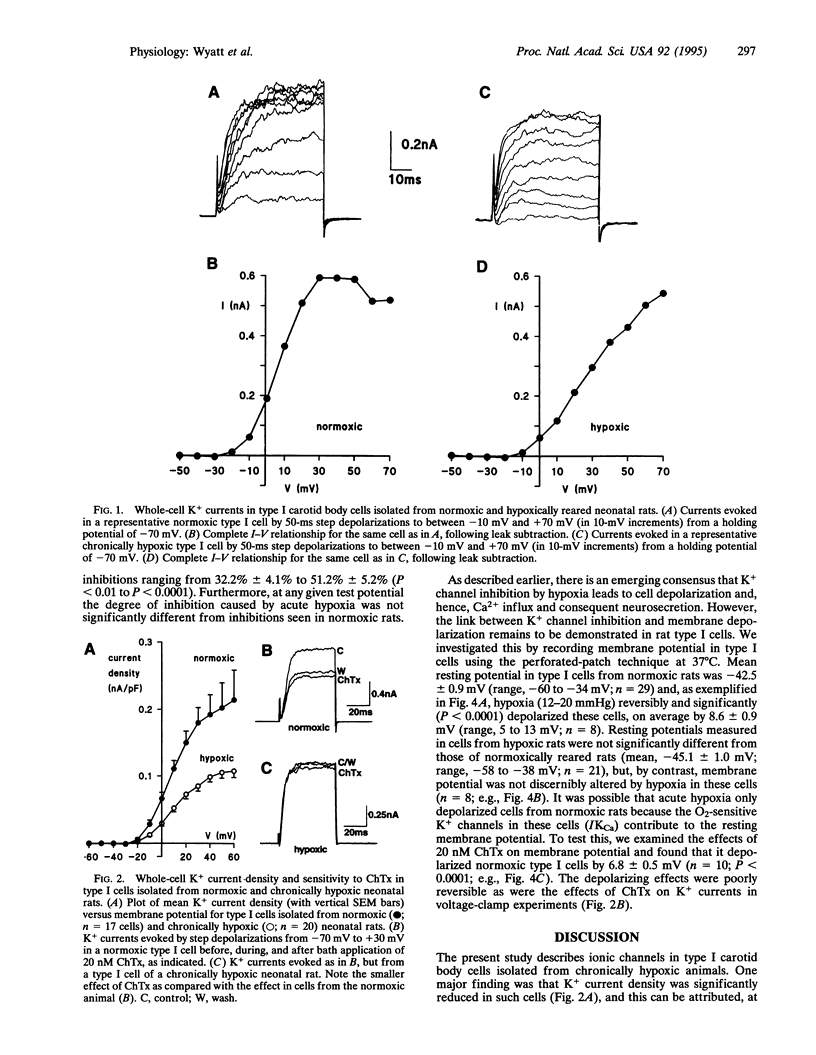
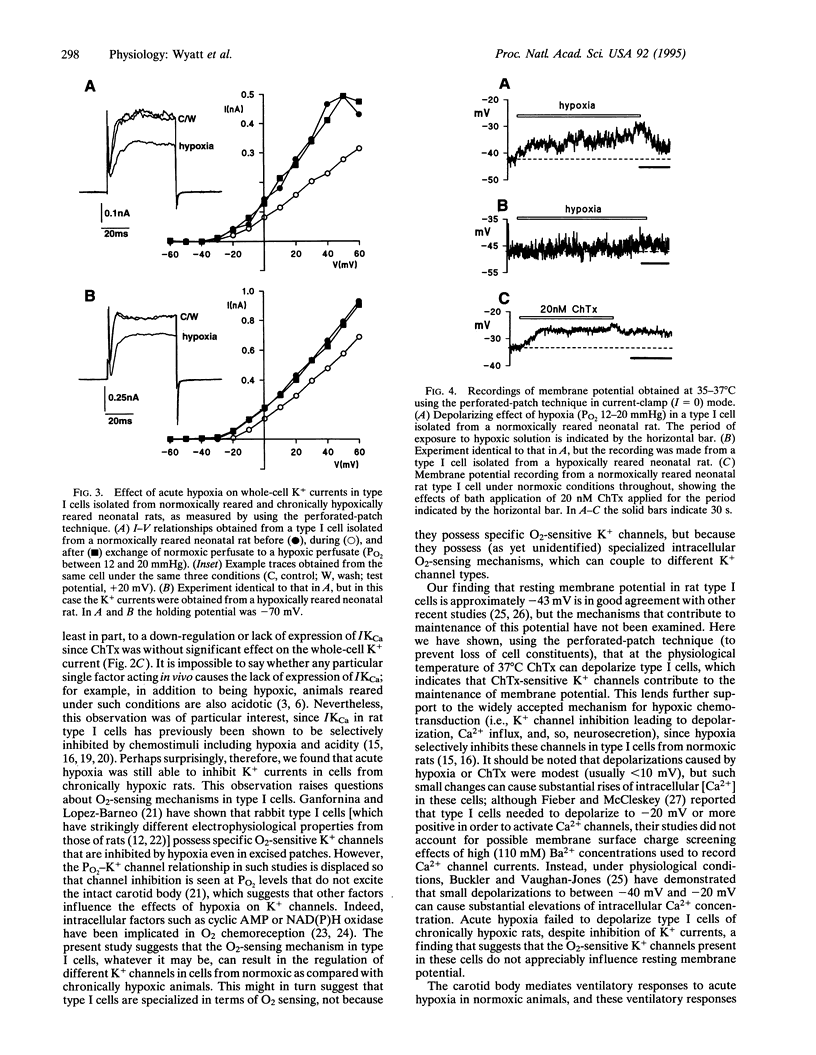
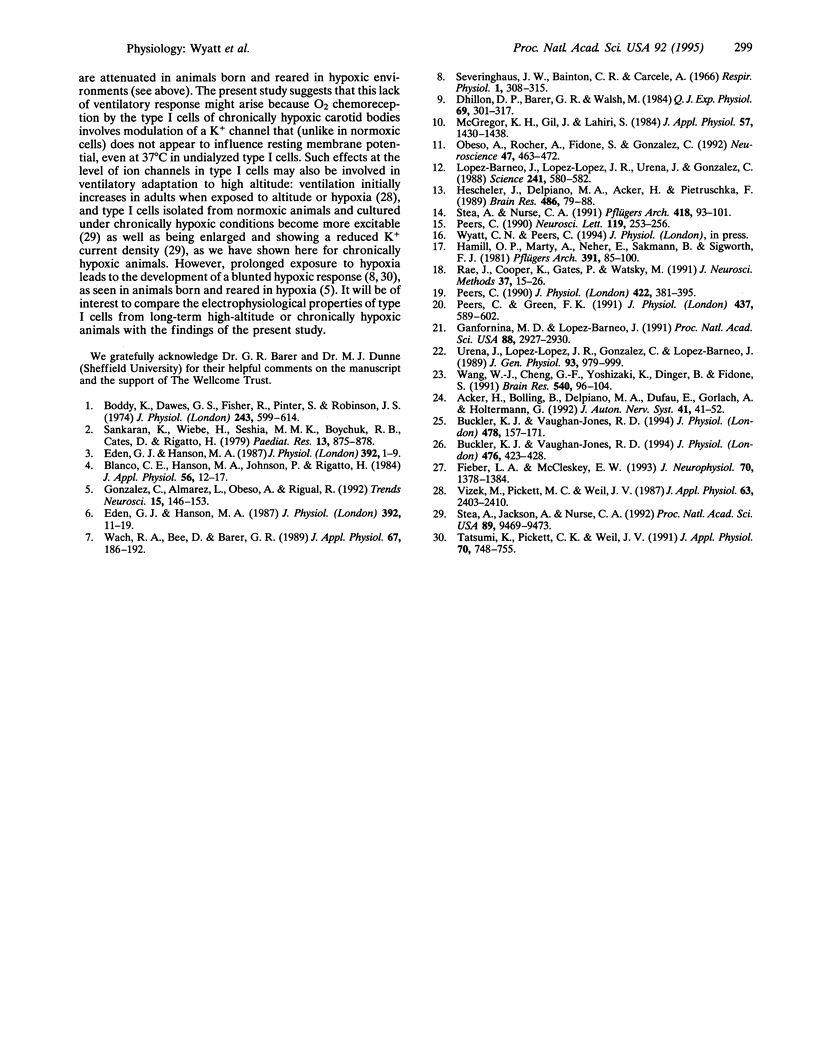
Selected References
These references are in PubMed. This may not be the complete list of references from this article.
- Blanco C. E., Hanson M. A., Johnson P., Rigatto H. Breathing pattern of kittens during hypoxia. J Appl Physiol Respir Environ Exerc Physiol. 1984 Jan;56(1):12–17. doi: 10.1152/jappl.1984.56.1.12. [DOI] [PubMed] [Google Scholar]
- Boddy K., Dawes G. S., Fisher R., Pinter S., Robinson J. S. Foetal respiratory movements, electrocortical and cardiovascular responses to hypoxaemia and hypercapnia in sheep. J Physiol. 1974 Dec;243(3):599–618. doi: 10.1113/jphysiol.1974.sp010768. [DOI] [PMC free article] [PubMed] [Google Scholar]
- Buckler K. J., Vaughan-Jones R. D. Effects of hypercapnia on membrane potential and intracellular calcium in rat carotid body type I cells. J Physiol. 1994 Jul 1;478(Pt 1):157–171. doi: 10.1113/jphysiol.1994.sp020239. [DOI] [PMC free article] [PubMed] [Google Scholar]
- Buckler K. J., Vaughan-Jones R. D. Effects of hypoxia on membrane potential and intracellular calcium in rat neonatal carotid body type I cells. J Physiol. 1994 May 1;476(3):423–428. doi: 10.1113/jphysiol.1994.sp020143. [DOI] [PMC free article] [PubMed] [Google Scholar]
- Dhillon D. P., Barer G. R., Walsh M. The enlarged carotid body of the chronically hypoxic and chronically hypoxic and hypercapnic rat: a morphometric analysis. Q J Exp Physiol. 1984 Apr;69(2):301–317. doi: 10.1113/expphysiol.1984.sp002807. [DOI] [PubMed] [Google Scholar]
- Eden G. J., Hanson M. A. Effects of chronic hypoxia from birth on the ventilatory response to acute hypoxia in the newborn rat. J Physiol. 1987 Nov;392:11–19. doi: 10.1113/jphysiol.1987.sp016766. [DOI] [PMC free article] [PubMed] [Google Scholar]
- Eden G. J., Hanson M. A. Maturation of the respiratory response to acute hypoxia in the newborn rat. J Physiol. 1987 Nov;392:1–9. doi: 10.1113/jphysiol.1987.sp016765. [DOI] [PMC free article] [PubMed] [Google Scholar]
- Fieber L. A., McCleskey E. W. L-type calcium channels in type I cells of the rat carotid body. J Neurophysiol. 1993 Oct;70(4):1378–1384. doi: 10.1152/jn.1993.70.4.1378. [DOI] [PubMed] [Google Scholar]
- Ganfornina M. D., López-Barneo J. Single K+ channels in membrane patches of arterial chemoreceptor cells are modulated by O2 tension. Proc Natl Acad Sci U S A. 1991 Apr 1;88(7):2927–2930. doi: 10.1073/pnas.88.7.2927. [DOI] [PMC free article] [PubMed] [Google Scholar]
- González C., Almaraz L., Obeso A., Rigual R. Oxygen and acid chemoreception in the carotid body chemoreceptors. Trends Neurosci. 1992 Apr;15(4):146–153. doi: 10.1016/0166-2236(92)90357-e. [DOI] [PubMed] [Google Scholar]
- Hamill O. P., Marty A., Neher E., Sakmann B., Sigworth F. J. Improved patch-clamp techniques for high-resolution current recording from cells and cell-free membrane patches. Pflugers Arch. 1981 Aug;391(2):85–100. doi: 10.1007/BF00656997. [DOI] [PubMed] [Google Scholar]
- Hescheler J., Delpiano M. A., Acker H., Pietruschka F. Ionic currents on type-I cells of the rabbit carotid body measured by voltage-clamp experiments and the effect of hypoxia. Brain Res. 1989 May 1;486(1):79–88. doi: 10.1016/0006-8993(89)91280-8. [DOI] [PubMed] [Google Scholar]
- López-Barneo J., López-López J. R., Ureña J., González C. Chemotransduction in the carotid body: K+ current modulated by PO2 in type I chemoreceptor cells. Science. 1988 Jul 29;241(4865):580–582. doi: 10.1126/science.2456613. [DOI] [PubMed] [Google Scholar]
- McGregor K. H., Gil J., Lahiri S. A morphometric study of the carotid body in chronically hypoxic rats. J Appl Physiol Respir Environ Exerc Physiol. 1984 Nov;57(5):1430–1438. doi: 10.1152/jappl.1984.57.5.1430. [DOI] [PubMed] [Google Scholar]
- Obeso A., Rocher A., Fidone S., Gonzalez C. The role of dihydropyridine-sensitive Ca2+ channels in stimulus-evoked catecholamine release from chemoreceptor cells of the carotid body. Neuroscience. 1992;47(2):463–472. doi: 10.1016/0306-4522(92)90260-9. [DOI] [PubMed] [Google Scholar]
- Peers C., Green F. K. Inhibition of Ca(2+)-activated K+ currents by intracellular acidosis in isolated type I cells of the neonatal rat carotid body. J Physiol. 1991 Jun;437:589–602. doi: 10.1113/jphysiol.1991.sp018613. [DOI] [PMC free article] [PubMed] [Google Scholar]
- Peers C. Hypoxic suppression of K+ currents in type I carotid body cells: selective effect on the Ca2(+)-activated K+ current. Neurosci Lett. 1990 Nov 13;119(2):253–256. doi: 10.1016/0304-3940(90)90846-2. [DOI] [PubMed] [Google Scholar]
- Rae J., Cooper K., Gates P., Watsky M. Low access resistance perforated patch recordings using amphotericin B. J Neurosci Methods. 1991 Mar;37(1):15–26. doi: 10.1016/0165-0270(91)90017-t. [DOI] [PubMed] [Google Scholar]
- Sankaran K., Wiebe H., Seshia M. M., Boychuk R. B., Cates D., Rigatto H. Immediate and late ventillatory response to high and low O2 in preterm infants and adult subjects. Pediatr Res. 1979 Aug;13(8):875–878. doi: 10.1203/00006450-197908000-00001. [DOI] [PubMed] [Google Scholar]
- Severinghaus J. W., Bainton C. R., Carcelen A. Respiratory insensitivity to hypoxia in chronically hypoxic man. Respir Physiol. 1966;1(3):308–334. doi: 10.1016/0034-5687(66)90049-1. [DOI] [PubMed] [Google Scholar]
- Stea A., Jackson A., Nurse C. A. Hypoxia and N6,O2'-dibutyryladenosine 3',5'-cyclic monophosphate, but not nerve growth factor, induce Na+ channels and hypertrophy in chromaffin-like arterial chemoreceptors. Proc Natl Acad Sci U S A. 1992 Oct 15;89(20):9469–9473. doi: 10.1073/pnas.89.20.9469. [DOI] [PMC free article] [PubMed] [Google Scholar]
- Stea A., Nurse C. A. Whole-cell and perforated-patch recordings from O2-sensitive rat carotid body cells grown in short- and long-term culture. Pflugers Arch. 1991 Mar;418(1-2):93–101. doi: 10.1007/BF00370457. [DOI] [PubMed] [Google Scholar]
- Tatsumi K., Pickett C. K., Weil J. V. Attenuated carotid body hypoxic sensitivity after prolonged hypoxic exposure. J Appl Physiol (1985) 1991 Feb;70(2):748–755. doi: 10.1152/jappl.1991.70.2.748. [DOI] [PubMed] [Google Scholar]
- Ureña J., López-López J., González C., López-Barneo J. Ionic currents in dispersed chemoreceptor cells of the mammalian carotid body. J Gen Physiol. 1989 May;93(5):979–999. doi: 10.1085/jgp.93.5.979. [DOI] [PMC free article] [PubMed] [Google Scholar]
- Vizek M., Pickett C. K., Weil J. V. Increased carotid body hypoxic sensitivity during acclimatization to hypobaric hypoxia. J Appl Physiol (1985) 1987 Dec;63(6):2403–2410. doi: 10.1152/jappl.1987.63.6.2403. [DOI] [PubMed] [Google Scholar]
- Wach R. A., Bee D., Barer G. R. Dopamine and ventilatory effects of hypoxia and almitrine in chronically hypoxic rats. J Appl Physiol (1985) 1989 Jul;67(1):186–192. doi: 10.1152/jappl.1989.67.1.186. [DOI] [PubMed] [Google Scholar]
- Wang W. J., Cheng G. F., Yoshizaki K., Dinger B., Fidone S. The role of cyclic AMP in chemoreception in the rabbit carotid body. Brain Res. 1991 Feb 1;540(1-2):96–104. doi: 10.1016/0006-8993(91)90495-h. [DOI] [PubMed] [Google Scholar]