Abstract
To determine whether the apparent enhanced force-generating capabilities of smooth muscle relative to skeletal muscle are inherent to the myosin cross-bridge, the isometric steady-state force produced by myosin in the in vitro motility assay was measured. In this assay, myosin adhered to a glass surface pulls on an actin filament that is attached to an ultracompliant (50-200 nm/pN) glass microneedle. The number of myosin cross-bridge heads able to interact with a length of actin filament was estimated by measuring the density of biochemically active myosin adhered to the surface; with this estimate, the average force per cross-bridge head of smooth and skeletal muscle myosins is 0.6 pN and 0.2 pN, respectively. Surprisingly, smooth muscle myosin generates approximately three times greater average force per cross-bridge head than does skeletal muscle myosin.
Full text
PDF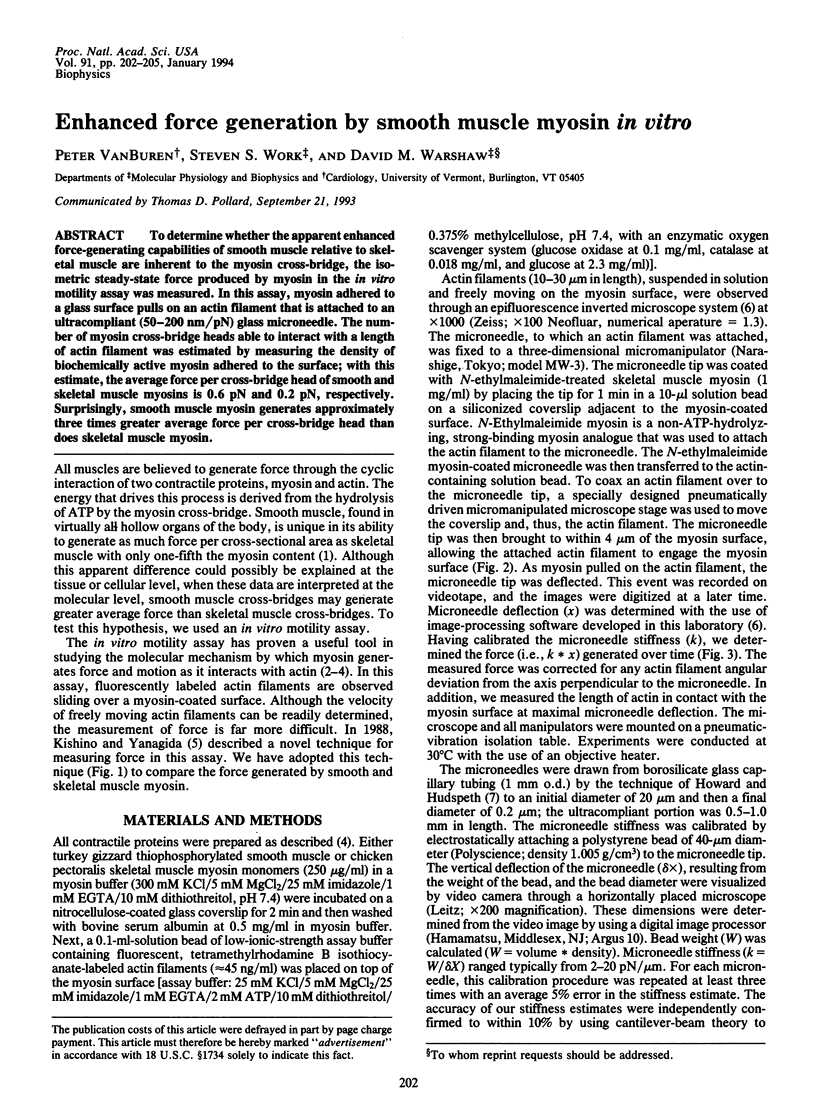
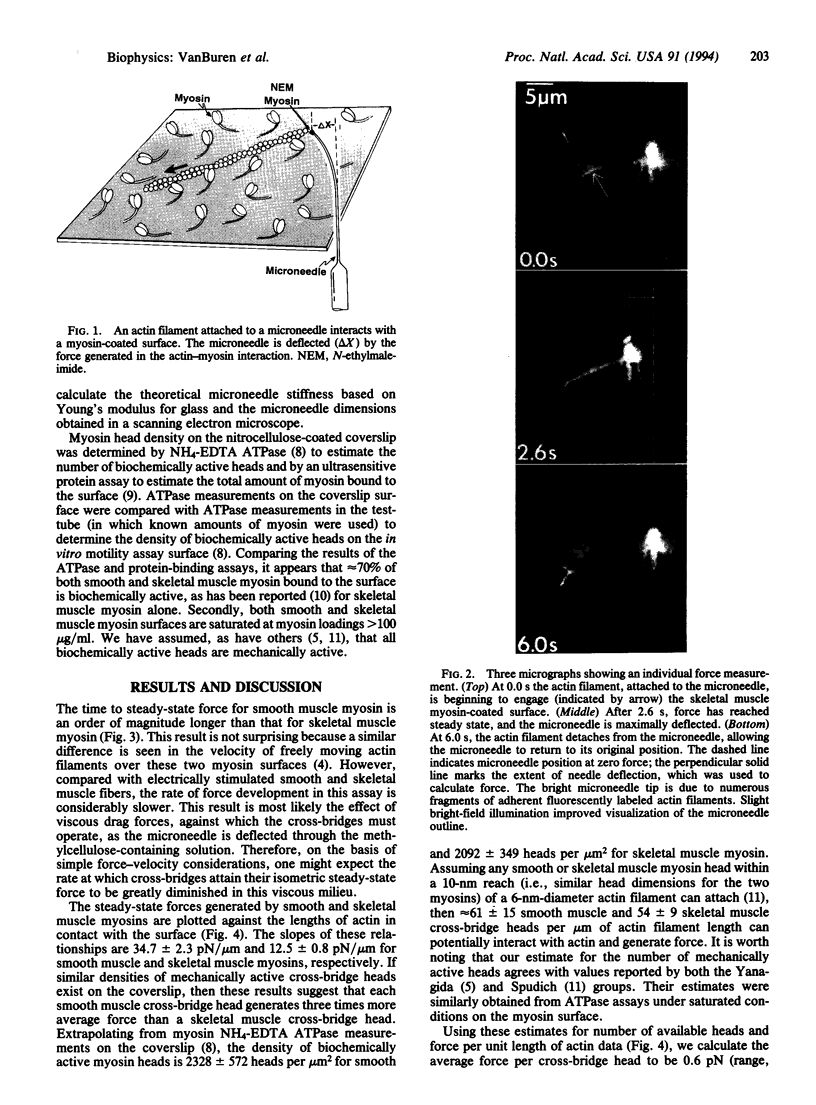
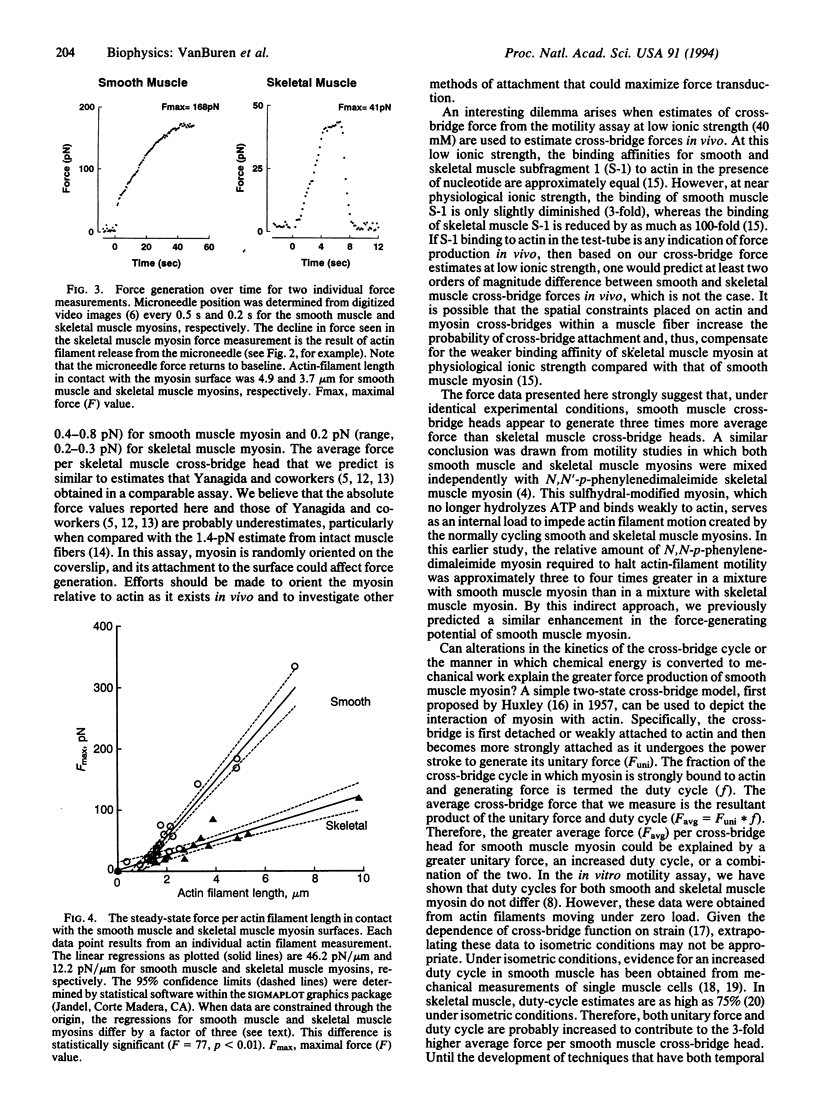
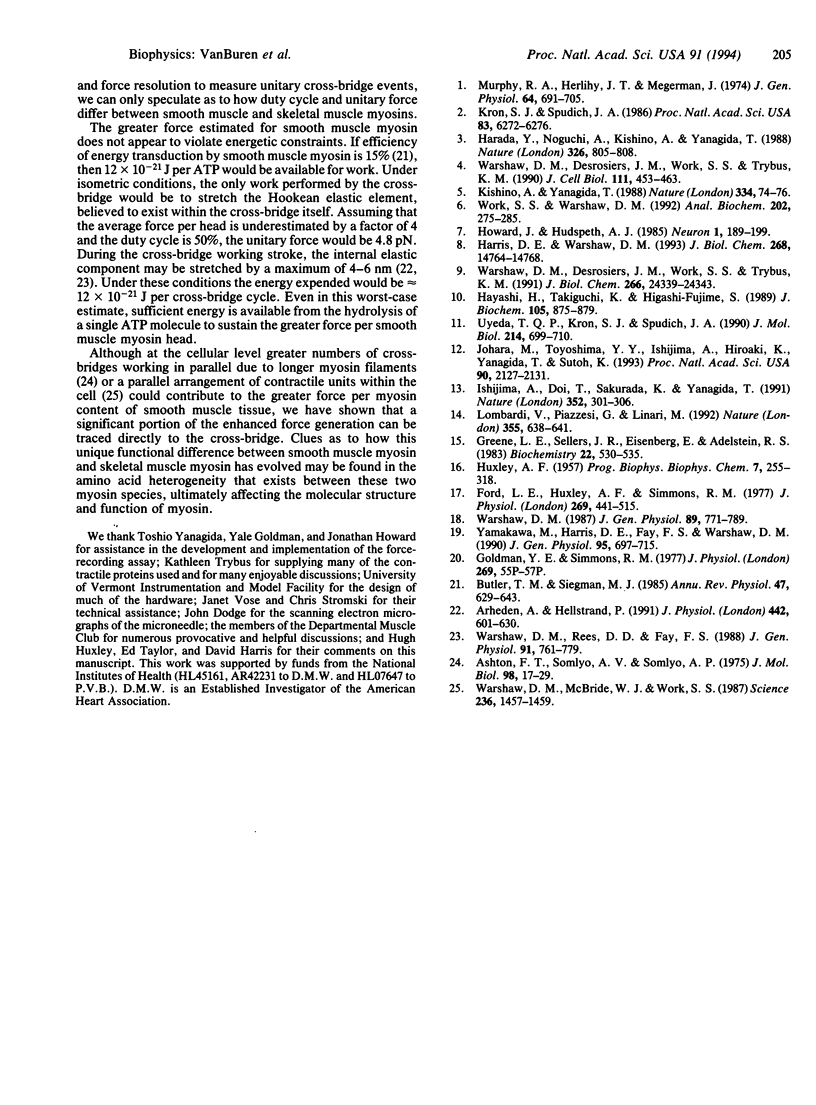
Images in this article
Selected References
These references are in PubMed. This may not be the complete list of references from this article.
- Arheden H., Hellstrand P. Force response to rapid length change during contraction and rigor in skinned smooth muscle of guinea-pig taenia coli. J Physiol. 1991 Oct;442:601–630. doi: 10.1113/jphysiol.1991.sp018811. [DOI] [PMC free article] [PubMed] [Google Scholar]
- Ashton F. T., Somlyo A. V., Somlyo A. P. The contractile apparatus of vascular smooth muscle: intermediate high voltage stereo electron microscopy. J Mol Biol. 1975 Oct 15;98(1):17–29. doi: 10.1016/s0022-2836(75)80098-2. [DOI] [PubMed] [Google Scholar]
- Butler T. M., Siegman M. J. High-energy phosphate metabolism in vascular smooth muscle. Annu Rev Physiol. 1985;47:629–643. doi: 10.1146/annurev.ph.47.030185.003213. [DOI] [PubMed] [Google Scholar]
- Ford L. E., Huxley A. F., Simmons R. M. Tension responses to sudden length change in stimulated frog muscle fibres near slack length. J Physiol. 1977 Jul;269(2):441–515. doi: 10.1113/jphysiol.1977.sp011911. [DOI] [PMC free article] [PubMed] [Google Scholar]
- Goldman Y. E., Simmons R. M. Active and rigor muscle stiffness [proceedings]. J Physiol. 1977 Jul;269(1):55P–57P. [PubMed] [Google Scholar]
- Greene L. E., Sellers J. R., Eisenberg E., Adelstein R. S. Binding of gizzard smooth muscle myosin subfragment 1 to actin in the presence and absence of adenosine 5'-triphosphate. Biochemistry. 1983 Feb 1;22(3):530–535. doi: 10.1021/bi00272a002. [DOI] [PubMed] [Google Scholar]
- HUXLEY A. F. Muscle structure and theories of contraction. Prog Biophys Biophys Chem. 1957;7:255–318. [PubMed] [Google Scholar]
- Harada Y., Noguchi A., Kishino A., Yanagida T. Sliding movement of single actin filaments on one-headed myosin filaments. Nature. 1987 Apr 23;326(6115):805–808. doi: 10.1038/326805a0. [DOI] [PubMed] [Google Scholar]
- Harris D. E., Warshaw D. M. Smooth and skeletal muscle myosin both exhibit low duty cycles at zero load in vitro. J Biol Chem. 1993 Jul 15;268(20):14764–14768. [PubMed] [Google Scholar]
- Hayashi H., Takiguchi K., Higashi-Fujime S. Measurement of ATPase activity of immobilized myosin heads. J Biochem. 1989 Jun;105(6):875–877. doi: 10.1093/oxfordjournals.jbchem.a122771. [DOI] [PubMed] [Google Scholar]
- Howard J., Hudspeth A. J. Compliance of the hair bundle associated with gating of mechanoelectrical transduction channels in the bullfrog's saccular hair cell. Neuron. 1988 May;1(3):189–199. doi: 10.1016/0896-6273(88)90139-0. [DOI] [PubMed] [Google Scholar]
- Ishijima A., Doi T., Sakurada K., Yanagida T. Sub-piconewton force fluctuations of actomyosin in vitro. Nature. 1991 Jul 25;352(6333):301–306. doi: 10.1038/352301a0. [DOI] [PubMed] [Google Scholar]
- Johara M., Toyoshima Y. Y., Ishijima A., Kojima H., Yanagida T., Sutoh K. Charge-reversion mutagenesis of Dictyostelium actin to map the surface recognized by myosin during ATP-driven sliding motion. Proc Natl Acad Sci U S A. 1993 Mar 15;90(6):2127–2131. doi: 10.1073/pnas.90.6.2127. [DOI] [PMC free article] [PubMed] [Google Scholar]
- Kishino A., Yanagida T. Force measurements by micromanipulation of a single actin filament by glass needles. Nature. 1988 Jul 7;334(6177):74–76. doi: 10.1038/334074a0. [DOI] [PubMed] [Google Scholar]
- Kron S. J., Spudich J. A. Fluorescent actin filaments move on myosin fixed to a glass surface. Proc Natl Acad Sci U S A. 1986 Sep;83(17):6272–6276. doi: 10.1073/pnas.83.17.6272. [DOI] [PMC free article] [PubMed] [Google Scholar]
- Lombardi V., Piazzesi G., Linari M. Rapid regeneration of the actin-myosin power stroke in contracting muscle. Nature. 1992 Feb 13;355(6361):638–641. doi: 10.1038/355638a0. [DOI] [PubMed] [Google Scholar]
- Murphy R. A., Herlihy J. T., Megerman J. Force-generating capacity and contractile protein content of arterial smooth muscle. J Gen Physiol. 1974 Dec;64(6):691–705. doi: 10.1085/jgp.64.6.691. [DOI] [PMC free article] [PubMed] [Google Scholar]
- Uyeda T. Q., Kron S. J., Spudich J. A. Myosin step size. Estimation from slow sliding movement of actin over low densities of heavy meromyosin. J Mol Biol. 1990 Aug 5;214(3):699–710. doi: 10.1016/0022-2836(90)90287-V. [DOI] [PubMed] [Google Scholar]
- Warshaw D. M., Desrosiers J. M., Work S. S., Trybus K. M. Effects of MgATP, MgADP, and Pi on actin movement by smooth muscle myosin. J Biol Chem. 1991 Dec 25;266(36):24339–24343. [PubMed] [Google Scholar]
- Warshaw D. M., Desrosiers J. M., Work S. S., Trybus K. M. Smooth muscle myosin cross-bridge interactions modulate actin filament sliding velocity in vitro. J Cell Biol. 1990 Aug;111(2):453–463. doi: 10.1083/jcb.111.2.453. [DOI] [PMC free article] [PubMed] [Google Scholar]
- Warshaw D. M. Force: velocity relationship in single isolated toad stomach smooth muscle cells. J Gen Physiol. 1987 May;89(5):771–789. doi: 10.1085/jgp.89.5.771. [DOI] [PMC free article] [PubMed] [Google Scholar]
- Warshaw D. M., McBride W. J., Work S. S. Corkscrew-like shortening in single smooth muscle cells. Science. 1987 Jun 12;236(4807):1457–1459. doi: 10.1126/science.3109034. [DOI] [PubMed] [Google Scholar]
- Warshaw D. M., Rees D. D., Fay F. S. Characterization of cross-bridge elasticity and kinetics of cross-bridge cycling during force development in single smooth muscle cells. J Gen Physiol. 1988 Jun;91(6):761–779. doi: 10.1085/jgp.91.6.761. [DOI] [PMC free article] [PubMed] [Google Scholar]
- Work S. S., Warshaw D. M. Computer-assisted tracking of actin filament motility. Anal Biochem. 1992 May 1;202(2):275–285. doi: 10.1016/0003-2697(92)90106-h. [DOI] [PubMed] [Google Scholar]
- Yamakawa M., Harris D. E., Fay F. S., Warshaw D. M. Mechanical transients of single toad stomach smooth muscle cells. Effects of lowering temperature and extracellular calcium. J Gen Physiol. 1990 Apr;95(4):697–715. doi: 10.1085/jgp.95.4.697. [DOI] [PMC free article] [PubMed] [Google Scholar]